Abstract
It is estimated that more than 1 million individuals will be affected annually by hepatocellular carcinoma (HCC) by 2025. HCC can be broadly grouped into two major molecular subgroups, each of which is characterized by specific morphological and phenotypic features that mirror the genetic background. The use of these tissue biomarkers in the daily practice of pathologists promises to better allocate patients with HCC with adequate treatments. In turn, this will likely boost the attitude of clinicians toward obtaining a pre-treatment biopsy.
Over the past three decades, the incidence of hepatocellular carcinoma (HCC) has been rising, and this trend is expected to continue through 2030.1 In 2018, liver cancer was the 6th most common cancer worldwide, with 841,080 new cases, and it was also the 4th leading cause of cancer-related deaths globally. By 2025, it is estimated that more than 1 million individuals will be affected by liver cancer annually.2 The vast majority (>90%) of HCC cases occur in the setting of chronic liver disease, and cirrhosis of any etiology is the strongest risk factor for HCC.3,4 Among the various etiologies, chronic hepatitis B virus (HBV) and hepatitis C virus (HCV) infections and non-alcoholic fatty liver disease/non-alcoholic steatohepatitis (NAFLD/NASH) play a major role. The lifetime risk of HBV-infected persons in developing cirrhosis and/or HCC is 15% to 40%.5–7 The introduction of direct acting antiviral therapy resulted in a 50–80% reduction in the risk of developing HCC in patients with HCV infection. 5–7 Nonetheless, chronic HCV infection is still associated with 50% of liver cancer in the USA.8 NAFLD/NASH has a global prevalence of 24%, with the highest rates reported in South America and the Middle East (≈30–35%).9 Since 2010, the proportion of NASH-associated HCC has rapidly increased, and NASH-associated HCC currently represents 15–20% of HCCs in the West.10
In patients with liver cirrhosis, the presence of a typical vascular pattern is sufficient for the diagnosis of HCC without histological confirmation.3,4 HCC lesions are brighter than the surrounding liver in the arterial phase on computed tomography or magnetic resonance imaging and less bright than the surrounding parenchyma in the venous and delayed phases. This phenomenon of ‘arterial enhancement and delayed washout’ is regarded as the radiological hallmark of HCC and has a sensitivity of 89% and a specificity of 96% for HCC.11 Alpha-fetoprotein (AFP) and other serum biomarkers generally have a minor role in the diagnosis of HCC.
Single HCC can be classified as “vaguely nodular” (a nodule with indistinct margins), “expanding nodular” (a round expansile nodule with a distinct margin), “multinodular confluent” (cluster of small and confluent nodules), “nodular with perinodular extension” (extranodular growth in <50% of the circumference), and “infiltrative” (extranodular growth in >50% of the tumor circumference).12 Single HCC with nodular morphology have favorable outcomes compared with those with multinodular, perinodular, or infiltrative growth patterns. 13–16 In up to 30% of cases, HCC present with multiple, clearly separated tumors, in which case the number of lesions should be recorded, and each lesion should be described in detail. A satellite nodule is a small nodule close to the main tumor (<2 cm).
Histological classification of HCC is performed based on the architectural growth patterns (microtrabecular, macrotrabecular, pseudoglandular, compact), cytological aspects (clear cell, fatty change, cholestasis, pleomorphic cells, spindle cells), and tumor grade, as defined by the World Health Organization.17 Approximately one third of HCCs can be classified as specific histotypes described in the following paragraphs (Fig. 1).18 It should be noted that different microscopic features frequently co-exist in the same lesion.
CTNNB1 encodes β-catenin, which is a key intracellular transducer of the WNT signaling pathway that regulates liver physiology and zonation.18 Mutations in CTNNB1 result in β-catenin stabilization and its subsequent nuclear accumulation, where it enhances cell proliferation and survival. CTNNB1-mutated HCCs are characterized by microtrabecular and pseudoglandular growth patterns, intratumoral cholestasis, lack of immune infiltration, glutamine synthetase (GS), and nuclear β-catenin expression at the phenotypical level. 19–23
MTM-HCC is defined by the presence of a macrotrabecular (trabeculae >6 cells thick) architectural pattern that constitutes a major proportion (>50%) of the lesion, regardless of the associated cytological features. They are associated with aggressive clinicopathological characteristics, including frequent satellite nodules, and vascular (micro and/or macro) invasion. Patients presenting with MTM-HCC are mostly infected with HBV and present with high alpha-fetoprotein serum levels.21,24 MTM-HCCs often demonstrate TP53 mutations and/or FGF19 amplification, in addition to angiogenesis activation, with overexpression of angiopoietin 2 and vascular endothelial growth factor A (VEGFA).19,21 A recent study further proved that MTM-HCC is characterized by higher expression of genes related to angiogenesis and identified ESM1 as a specific biomarker, also at the immunohistochemical level, of endothelial cells (EC) of MTM-HCC.25
The scirrhous subtype of HCC is characterized by an admixture of abundant dense fibrous stroma and neoplastic cells. The tumor cells frequently express markers associated with progenitor or cancer stem cells, including CK7, CK19, or CD133. Consistent with its characteristic histological appearance, scirrhous HCC has been associated with transforming growth factor beta (TGF-β) pathway activation, overexpression of VIM, SNAIL, SMAD4, and TWIST, and features of epithelial-to-mesenchymal transition.19,26
These tumors are usually well-differentiated and characterized by intratumoral inflammatory infiltrates, tumor cell ballooning, pericellular fibrosis, and Mallory-Denk bodies.27 At the phenotypical level, the neoplastic cells demonstrate overexpression of C-reactive protein (CRP), which is a target gene of the Janus kinase/signal transducers and activators of transcription protein (JAK/STAT) signaling pathway.19 SH-HCC are significantly enriched in patients with NASH and alcoholic steatohepatitis (ASH). The molecular profiles of SH-HCC developing in the setting of ASH or NASH are similar, suggesting that steatohepatitis per se, rather than the underlying liver disease, plays a major role at the cancer onset. 28
A recent study showed that non-viral HCC, particularly HCC developing in the setting of NASH, is less responsive to immunotherapy, further encouraging an adequate histopathological classification of HCC and surrounding liver background to properly address patients with different treatments. 29
This rare histotype is characterized by the presence of lymphocytes which outnumber the neoplastic cells in most fields on microscopic examination.17 Lymphocyte-rich HCC has been associated with a more favorable overall survival, which supports the hypothesis that intratumoral lymphocytic infiltration may exert an antitumor effect.30 The infiltrating lymphocytes are predominantly cytotoxic CD8+ T-lymphocytes and demonstrate increased PD-L1 and PD1 expression.30,31 Unlike some other cancers, such as colon and lung cancer, in which lymphocytic infiltration has been linked with microsatellite instability and/or high mutational burdens,32 none of these lymphocyte-rich HCCs have been found to possess microsatellite instability or to be associated with a higher number of somatic mutations.20,30
The existence of a specific subgroup of HCC characterized by enrichment in immune features, both at molecular and morphological levels, was first proved by Sia et al.20 The study demonstrated that approximately 25% of HCC cases belong to this immune class; it also showed that this rich immune infiltrate can be either active or exhausted: the former is characterized by overexpression of adaptive immune response genes and favorable outcome, the latter by immunosuppressive signals and poor outcome. However, the study failed to identify significant differences between the active and exhausted subgroups in terms of morpho-phenotypical features, such as the amount of immune infiltration and tertiary lymphoid structures (TLS) or PD-L1/PD-1 expression. The same study also demonstrated the existence of a subgroup of HCC devoid of immune infiltrates associated with CTNNB1 mutations, which was later defined as an immune-exclusion class.33 A further study by Kurebayashi et al.34 better dissected the morphological and phenotypical landscape of the HCC immune microenvironment, suggesting that HCC with an immune-rich, active profile is significantly enriched among specific histotypes such as lymphocyte-rich and SH-HCC or in HCC with specific microscopic features such as low vessel density or mild fibrosis. They also confirmed that most CTNNB1-mutated HCC belong to an immune-exclusion class, even if some exceptions were recorded. Based on these findings, it was postulated that the immune-class, opposite to the immune-exclusion class, might benefit with immune check point inhibitors (ICI) treatment.33 However, this hypothesis has not been proven yet.
Several molecular HCC subclasses have been identified in the last decade. Currently, HCC can be broadly grouped into two major subgroups (Fig. 2).35–42 The non-proliferation class is characterized by chromosomal stability and frequent TERT promoter mutations.42–45 These HCCs show grade 1–2 differentiation, lack vascular invasion, have low levels of AFP, and are related to NASH, ASH, and chronic HCV infection.19 Two distinct subgroups characterized this class: the WNT-β-catenin CTNNB1 subclass with low immune infiltration,19,43,45 and the interferon subclass, which demonstrates a highly activated IL6-JAK-STAT signaling pathway, with a more inflamed tumor microenvironment. The other major class of HCC, the proliferation class, is characterized by high chromosomal instability, global DNA hypomethylation, frequent TP53 mutations, and overexpression of cell cycle-associated genes.16,41,42 These HCCs show poor histological differentiation, frequent vascular invasion, increased serum AFP levels, and more aggressive clinical behavior.19 The proliferation class can be further divided into two subclasses. The S1 subclass (or iCluster 3)44,45 demonstrates Wnt-TGFβ activation and is morphologically characterized by a rich but exhausted immune phenotype20 or as MTM and scirrhous histotypes. The S2 subclass (or iCluster 1)44,45 is characterized by a progenitor-like phenotype, with the expression of stem cell-related markers (CK19, EPCAM) and activation of the IGF2 and EPCAM signaling pathways.19
Despite great advances in HCC knowledge, the identification of molecular subgroups did not identify categories with clearly different prognostic impacts or different responses to treatments. Accordingly, as detailed in the following section, HCC management is still based on clinical features, while specific biomarkers remain an unmet need.
In general, surgical resection or liver transplantation is the first option in treating early-stage HCC, yielding the best outcomes with a 5-year survival of ~70–80%.3,4,46 HCC recurrence after hepatic resection reaches 70% at 5 years, even in patients with a single tumor ≤2 cm.47 The 10-year recurrence rate after transplantation ranges between 10–15% (within Milan criteria) and 20% (down-staged to the Milan criteria).48 Thermal ablation showed outcomes similar to surgical resection in very early-stage disease (tumors <2 cm diameter). Therefore, it is recommended as a first-line treatment due to a lesser invasiveness and lower morbidity compared with surgery.49 Radiofrequency ablation and percutaneous ethanol injection have both been demonstrated to be effective for small tumors, but radiofrequency ablation is superior for tumors larger than 2 cm.50,51 For intermediate-stage HCC, transarterial chemoembolization (TACE) has been the most widely used treatment and the standard of care over the past two decades.49,52–54 TACE is recommended for patients who do not have vascular invasion or extrahepatic spread and are not candidates for liver transplantation, surgical resection, or local ablation due to a large tumor size or multifocality of the tumor,49,53 with an average median overall survival of ~30 months.54 Transarterial radioembolization (TARE) has shown efficacy in phase II investigations but has not been established as a primary standard of care by guidelines.55 Approximately, 50–60% of patients with HCC, mostly at the advanced stage, will be treated with systemic therapies. Significant progress has been made in this field in recent years. Until 2020, the approved treatment (sorafenib56 and lenvatinib57 as first-line treatment; regorafenib,58 cabozantinib, 59 and ramucirumab60 as second treatment) warranted a median survival of 8–10 months. However, this figure was more than doubled by the recently approved combination of atezolizumab (anti-PD-L1 antibody) and bevacizumab (anti-VEGF antibody).61
The significant results obtained with atezolizumab plus bevacizumab suggest that the HCC microenvironment may play a key role in dictating the HCC outcome. Consistent with this, it has been shown that a peculiar vascular phenotype, characterized by CD34+ vessels encapsulating tumor clusters (VETC), strongly predicts the HCC outcome (Fig. 3).23,62 VETC might be present in up to 40% of HCC23 and is associated with a higher attitude of tumoral cells to infiltrate vessels;23,62 moreover, it predicts the response to sorafenib.63 Interestingly, we observed that VETC was associated with a low immune infiltrate and CTNNB1-pathway.23 The relationship between the vascular pattern and immune infiltrate was recently investigated at the deepest level by Kurebayashi et al.64 They proved the existence of distinct immuno-vascular subtypes that reflect the interaction between the immune microenvironment and tumor angiogenesis. In particular, they confirmed that VETC is significantly associated with the CTNNB1 pathway and low immune infiltrate. These HCCs have a worse prognosis and likely represent a good target for anti-angiogenic therapies. In contrast, HCC with rich immune infiltrates are usually angiostatic and behave better. They also observed that not all HCC with a higher expression of angiogenic factors were VETC+ and showed that in these cases, T cells, B cells, and plasma cells were preferentially located around tumor vessels. Accordingly, they speculated that this milieu might inhibit the effects of angiogenic factors on tumor vessels.
Unfortunately, apart from alpha-fetoprotein which is used to select patients for ramucirumab, other biomarkers which may be used to identify patients who may respond to a specific treatment are lacking.65
Notes
Conflicts of Interest
LDT, a contributing editor of the Journal of Liver Cancer, was not involved in the editorial evaluation or decision to publish this article. The remaining author has declared no conflicts of interest.
Ethics Statement
This review article is fully based on the articles which was already published and did not involve additional patient participants. Therefore, IRB approval is not necessary.
Funding Statement
The research leading to these results has received funding from AIRC under IG 2020 - ID. 25087 project – P.I. Di Tommaso Luca.
References
1. Petrick JL, Kelly SP, Altekruse SF, McGlynn KA, Rosenberg PS. Future of hepatocellular carcinoma incidence in the United States forecast through 2030. J Clin Oncol. 2016; 34:1787–1794.


2. International Agency for Research on Cancer. GLOBOCAN 2018 [Internet]. Geneva: World Health Organization;[cited 2022 Feb 1]. Available from: https://gco.iarc.fr/today/online-analysis-map?v=2020&mode=population&mode_population=continents&population=900&populations=900&key=asr&sex=0&cancer=11&type=0&statistic=5&prevalence=0&population_groupearth&color_palette=default&map_scale=uantile&map_nb_colors=5&continent=0&rotate=%255B10%252C0%255D
.
3. Marrero JA, Kulik LM, Sirlin CB, Zhu AX, Finn RS, Abecassis MM, et al. Diagnosis, staging, and management of hepatocellular carcinoma: 2018 practice guidance by the American Association for the Study of Liver Diseases. Hepatology. 2018; 68:723–750.


4. European Association for the Study of the Liver. EASL clinical practice guidelines: management of hepatocellular carcinoma. J Hepatol. 2018; 69:182–236.
5. Fattovich G, Stroffolini T, Zagni I, Donato F. Hepatocellular carcinoma in cirrhosis: incidence and risk factors. Gastroenterology. 2004; 127(5 Suppl 1):S35–S50.


7. Kanwal F, Kramer J, Asch SM, Chayanupatkul M, Cao Y, El-Serag HB. Risk of hepatocellular cancer in HCV patients treated with direct-acting antiviral agents. Gastroenterology. 2017; 153:996–1005e1.


8. Perz JF, Armstrong GL, Farrington LA, Hutin YJ, Bell BP. The contributions of hepatitis B virus and hepatitis C virus infections to cirrhosis and primary liver cancer worldwide. J Hepatol. 2006; 45:529–538.


9. Younossi Z, Anstee QM, Marietti M, Hardy T, Henry L, Eslam M, et al. Global burden of NAFLD and NASH: trends, predictions, risk factors and prevention. Nat Rev Gastroenterol Hepatol. 2018; 15:11–20.


10. Estes C, Razavi H, Loomba R, Younossi Z, Sanyal AJ. Modeling the epidemic of nonalcoholic fatty liver disease demonstrates an exponential increase in burden of disease. Hepatology. 2018; 67:123–133.


11. van der Pol CB, Lim CS, Sirlin CB, McGrath TA, Salameh JP, Bashir MR, et al. Accuracy of the liver imaging reporting and data system in computed tomography and magnetic resonance image analysis of hepatocellular carcinoma or overall malignancy-A systematic review. Gastroenterology. 2019; 156:976–986.


12. Jang JY, Lee JS, Kim HJ, Shim JJ, Kim JH, Kim BH, et al. The general rules for the study of primary liver cancer. J Liver Cancer. 2017; 17:19–44.


13. Hui AM, Takayama T, Sano K, Kubota K, Akahane M, Ohtomo K, et al. Predictive value of gross classification of hepatocellular carcinoma on recurrence and survival after hepatectomy. J Hepatol. 2000; 33:975–979.


14. Shimada M, Rikimaru T, Hamatsu T, Yamashita Y, Terashi T, Taguchi K, et al. The role of macroscopic classification in nodular-type hepatocellular carcinoma. Am J Surg. 2001; 182:177–182.


15. Tsujita E, Yamashita Y, Takeishi K, Matsuyama A, Maeda T, Tsutsui S, et al. The clinicopathological impact of gross classification on solitary small hepatocellular carcinoma. Hepatogastroenterology. 2013; 60:1726–1730.
16. Lee Y, Park H, Lee H, Cho JY, Yoon YS, Choi YR, et al. The clinicopathological and prognostic significance of the gross classification of hepatocellular carcinoma. J Pathol Transl Med. 2018; 52:85–92.


17. WHO. Classification of Tumours Editorial Board. Digestive system tumours. 1:5th ed. Lyon: International Agency for Research on Cancer;2019.
18. Monga SP. β-catenin signaling and roles in liver homeostasis, injury, and tumorigenesis. Gastroenterology. 2015; 148:1294–1310.


19. Calderaro J, Ziol M, Paradis V, Zucman-Rossi J. Molecular and histological correlations in liver cancer. J Hepatol. 2019; 71:616–630.


20. Sia D, Jiao Y, Martinez-Quetglas I, Kuchuk O, Villacorta-Martin C, Castro de Moura M, et al. Identification of an immune-specific class of hepatocellular carcinoma, based on molecular features. Gastroenterology. 2017; 153:812–826.


21. Calderaro J, Couchy G, Imbeaud S, Amaddeo G, Letouzé E, Blanc JF, et al. Histological subtypes of hepatocellular carcinoma are related to gene mutations and molecular tumour classification. J Hepatol. 2017; 67:727–738.


22. Audard V, Grimber G, Elie C, Radenen B, Audebourg A, Letourneur F, et al. Cholestasis is a marker for hepatocellular carcinomas displaying beta-catenin mutations. J Pathol. 2007; 212:345–352.


23. Renne SL, Woo HY, Allegra S, Rudini N, Yano H, Donadon M, et al. Vessels encapsulating tumor clusters (VETC) is a powerful predictor of aggressive hepatocellular carcinoma. Hepatology. 2020; 71:183–195.


24. Ziol M, Poté N, Amaddeo G, Laurent A, Nault JC, Oberti F, et al. Macrotrabecular-massive hepatocellular carcinoma: a distinctive histological subtype with clinical relevance. Hepatology. 2018; 68:103–112.


25. Calderaro J, Meunier L, Nguyen CT, Boubaya M, Caruso S, Luciani A, et al. ESM1 as a marker of macrotrabecular-massive hepatocellular carcinoma. Clin Cancer Res. 2019; 25:5859–5865.


26. Seok JY, Na DC, Woo HG, Roncalli M, Kwon SM, Yoo JE, et al. A fibrous stromal component in hepatocellular carcinoma reveals a cholangiocarcinoma-like gene expression trait and epithelial-mesenchymal transition. Hepatology. 2012; 55:1776–1786.


27. Salomao M, Yu WM, Brown RS Jr, Emond JC, Lefkowitch JH. Steatohepatitic hepatocellular carcinoma (SH-HCC): a distinctive histological variant of HCC in hepatitis C virus-related cirrhosis with associated NAFLD/NASH. Am J Surg Pathol. 2010; 34:1630–1636.
28. Qin J, Higashi T, Nakagawa S, Fujiwara N, Yamashita YI, Beppu T, et al. Steatohepatitic variant of hepatocellular carcinoma is associated with both alcoholic steatohepatitis and nonalcoholic steatohepatitis: a study of 2 cohorts with molecular insights. Am J Surg Pathol. 2020; 44:1406–1412.


29. Pfister D, Núñez NG, Pinyol R, Govaere O, Pinter M, Szydlowska M, et al. NASH limits anti-tumour surveillance in immunotherapy-treated HCC. Nature. 2021; 592:450–456.
30. Chan AW, Tong JH, Pan Y, Chan SL, Wong GL, Wong VW, et al. Lymphoepithelioma-like hepatocellular carcinoma: an uncommon variant of hepatocellular carcinoma with favorable outcome. Am J Surg Pathol. 2015; 39:304–312.
31. Calderaro J, Rousseau B, Amaddeo G, Mercey M, Charpy C, Costentin C, et al. Programmed death ligand 1 expression in hepatocellular carcinoma: relationship with clinical and pathological features. Hepatology. 2016; 64:2038–2046.


32. Turajlic S, Litchfield K, Xu H, Rosenthal R, McGranahan N, Reading JL, et al. Insertion-and-deletion-derived tumour-specific neoantigens and the immunogenic phenotype: a pan-cancer analysis. Lancet Oncol. 2017; 18:1009–1021.


33. Pinyol R, Sia D, Llovet JM. Immune exclusion-Wnt/CTNNB1 class predicts resistance to immunotherapies in HCC. Clin Cancer Res. 2019; 25:2021–2023.


34. Kurebayashi Y, Ojima H, Tsujikawa H, Kubota N, Maehara J, Abe Y, et al. Landscape of immune microenvironment in hepatocellular carcinoma and its additional impact on histological and molecular classification. Hepatology. 2018; 68:1025–1041.


35. Nault JC, Mallet M, Pilati C, Calderaro J, Bioulac-Sage P, Laurent C, et al. High frequency of telomerase reverse-transcriptase promoter somatic mutations in hepatocellular carcinoma and preneoplastic lesions. Nat Commun. 2013; 4:2218.


36. Zucman-Rossi J, Villanueva A, Nault JC, Llovet JM. Genetic landscape and biomarkers of hepatocellular carcinoma. Gastroenterology. 2015; 149:1226–1239e4.


37. Ahn SM, Jang SJ, Shim JH, Kim D, Hong SM, Sung CO, et al. Genomic portrait of resectable hepatocellular carcinomas: implications of RB1 and FGF19 aberrations for patient stratification. Hepatology. 2014; 60:1972–1982.


38. Schulze K, Imbeaud S, Letouzé E, Alexandrov LB, Calderaro J, Rebouissou S, et al. Exome sequencing of hepatocellular carcinomas identifies new mutational signatures and potential therapeutic targets. Nat Genet. 2015; 47:505–511.


39. Guichard C, Amaddeo G, Imbeaud S, Ladeiro Y, Pelletier L, Maad IB, et al. Integrated analysis of somatic mutations and focal copy-number changes identifies key genes and pathways in hepatocellular carcinoma. Nat Genet. 2012; 44:694–698.


40. Totoki Y, Tatsuno K, Covington KR, Ueda H, Creighton CJ, Kato M, et al. Trans-ancestry mutational landscape of hepatocellular carcinoma genomes. Nat Genet. 2014; 46:1267–1273.


41. Hoshida Y, Nijman SM, Kobayashi M, Chan JA, Brunet JP, Chiang DY, et al. Integrative transcriptome analysis reveals common molecular subclasses of human hepatocellular carcinoma. Cancer Res. 2009; 69:7385–7392.


42. Boyault S, Rickman DS, de Reyniès A, Balabaud C, Rebouissou S, Jeannot E, et al. Transcriptome classification of HCC is related to gene alterations and to new therapeutic targets. Hepatology. 2007; 45:42–52.


43. Chiang DY, Villanueva A, Hoshida Y, Peix J, Newell P, Minguez B, et al. Focal gains of VEGFA and molecular classification of hepatocellular carcinoma. Cancer Res. 2008; 68:6779–6788.


44. Cancer Genome Atlas Research Network. Comprehensive and integrative genomic characterization of hepatocellular carcinoma. Cell. 2017; 169:1327–1341e23.
45. Lee JS, Heo J, Libbrecht L, Chu IS, Kaposi-Novak P, Calvisi DF, et al. A novel prognostic subtype of human hepatocellular carcinoma derived from hepatic progenitor cells. Nat Med. 2006; 12:410–416.


46. Mazzaferro V, Regalia E, Doci R, Andreola S, Pulvirenti A, Bozzetti F, et al. Liver transplantation for the treatment of small hepatocellular carcinomas in patients with cirrhosis. N Engl J Med. 1996; 334:693–699.


47. Roayaie S, Obeidat K, Sposito C, Mariani L, Bhoori S, Pellegrinelli A, et al. Resection of hepatocellular cancer ≤2 cm: results from two Western centers. Hepatology. 2013; 57:1426–1435.


48. Tabrizian P, Holzner M, Halazun K, Agopian VG, Busuttil RW, Yao F, et al. A US multicenter analysis of 2529 HCC patients undergoing liver transplantation: 10-year outcome assessing the role of down-staging to within Milan criteria [abstract]. Hepatology. 2019; 70(Suppl 1):10A.
49. Heimbach JK, Kulik LM, Finn RS, Sirlin CB, Abecassis MM, Roberts LR, et al. AASLD guidelines for the treatment of hepatocellular carcinoma. Hepatology. 2018; 67:358–380.


50. Germani G, Pleguezuelo M, Gurusamy K, Meyer T, Isgrò G, Burroughs AK. Clinical outcomes of radiofrequency ablation, percutaneous alcohol and acetic acid injection for hepatocelullar carcinoma: a meta-analysis. J Hepatol. 2010; 52:380–388.


51. Cho YK, Kim JK, Kim MY, Rhim H, Han JK. Systematic review of randomized trials for hepatocellular carcinoma treated with percutaneous ablation therapies. Hepatology. 2009; 49:453–459.


52. Llovet JM, Bruix J. Systematic review of randomized trials for unresectable hepatocellular carcinoma: chemoembolization improves survival. Hepatology. 2003; 37:429–442.


53. Vogel A, Cervantes A, Chau I, Daniele B, Llovet JM, Meyer T, et al. Hepatocellular carcinoma: ESMO clinical practice guidelines for diagnosis, treatment and follow-up. Ann Oncol. 2018; 29(Suppl 4):iv238–iv255.


54. Llovet JM, De Baere T, Kulik L, Haber PK, Greten TF, Meyer T, et al. Locoregional therapies in the era of molecular and immune treatments for hepatocellular carcinoma. Nat Rev Gastroenterol Hepatol. 2021; 18:293–313.


55. Salem R, Gordon AC, Mouli S, Hickey R, Kallini J, Gabr A, et al. Y90 radioembolization significantly prolongs time to progression compared with chemoembolization in patients with hepatocellular carcinoma. Gastroenterology. 2016; 151:1155–1163e2.


56. Llovet JM, Ricci S, Mazzaferro V, Hilgard P, Gane E, Blanc JF, et al. Sorafenib in advanced hepatocellular carcinoma. N Engl J Med. 2008; 359:378–390.


57. Kudo M, Finn RS, Qin S, Han KH, Ikeda K, Piscaglia F, et al. Lenvatinib versus sorafenib in first-line treatment of patients with unresectable hepatocellular carcinoma: a randomised phase 3 non-inferiority trial. Lancet. 2018; 391:1163–1173.


58. Bruix J, Qin S, Merle P, Granito A, Huang YH, Bodoky G, et al. Regorafenib for patients with hepatocellular carcinoma who progressed on sorafenib treatment (RESORCE): a randomised, double-blind, placebo-controlled, phase 3 trial. Lancet. 2017; 389:56–66.


59. Abou-Alfa GK, Meyer T, Cheng AL, El-Khoueiry AB, Rimassa L, Ryoo BY, et al. Cabozantinib in patients with advanced and progressing hepatocellular carcinoma. N Engl J Med. 2018; 379:54–63.


60. Zhu AX, Kang YK, Yen CJ, Finn RS, Galle PR, Llovet JM, et al. Ramucirumab after sorafenib in patients with advanced hepatocellular carcinoma and increased α-fetoprotein concentrations (REACH-2): a randomised, double-blind, placebo-controlled, phase 3 trial. Lancet Oncol. 2019; 20:282–296.


61. Finn RS, Qin S, Ikeda M, Galle PR, Ducreux M, Kim TY, et al. Atezolizumab plus bevacizumab in unresectable hepatocellular carcinoma. N Engl J Med. 2020; 382:1894–1905.


62. Fang JH, Zhou HC, Zhang C, Shang LR, Zhang L, Xu J, et al. A novel vascular pattern promotes metastasis of hepatocellular carcinoma in an epithelial-mesenchymal transition-independent manner. Hepatology. 2015; 62:452–465.


63. Fang JH, Xu L, Shang LR, Pan CZ, Ding J, Tang YQ, et al. Vessels that encapsulate tumor clusters (VETC) pattern is a predictor of sorafenib benefit in patients with hepatocellular carcinoma. Hepatology. 2019; 70:824–839.


64. Kurebayashi Y, Matsuda K, Ueno A, Tsujikawa H, Yamazaki K, Masugi Y, et al. Immunovascular classification of HCC reflects reciprocal interaction between immune and angiogenic tumor microenvironments. Hepatology. 2021; Oct. 17. DOI: 10.1002/hep.32201. [Epub ahead of print].


Figure 1
Microscopic pathology of hepatocellular carcinoma (HCC) (hematoxylin-eosin stain). (A, B) A well-differentiated HCC with a thick perilesional capsule, characterized at higher magnification by pseudoglandular structures filled with bile and showing strong and homogenous immunoreactivity to glutamine synthetase (inset). These findings are consistent with the diagnosis of CTNNB1-mutated-HCC. (C, D) HCC showing a homogenous and diffuse presence of macrotrabeculae, consistent with the diagnosis of macrotrabecular-massive HCC. (E, F) This HCC is characterized by diffuse steatosis at scanning magnification and hepatocytes with Mallory-Denk bodies and ballooning, intermingled with scattered inflammatory infiltrates at higher magnification. These features are consistent with the diagnosis of steatohepatitic HCC. (G, H) HCC showing homogenous presence of a rich immune infiltrate, which at higher magnification are confirmed to be lymphocytes surrounding neoplastic cells. These findings are consistent with the diagnosis of lymphocyte-rich HCC. (I, J) Scirrhous HCC characterized by the diffuse presence of fibrous stroma, surrounding neoplastic cells. (K, L) This HCC is characterized by the diffuse presence of clear cell changes, consistent with a clear cell variant.

Figure 2
Molecular pathology of hepatocellular carcinoma (HCC). A summary of the correlations among molecular, genetic, morphological and clinical features of HCC. TGFβ, transforming growth factor beta; IL6-JAK-STAT, interleukin 6-Janus kinase-signal transducers and activators of transcription protein; HCC, hepatocellular carcinoma; MTM, macrotrabecular-massive; HBV, hepatitis B virus; AFP, α-fetoprotein; CRP, C-reactive protein; GS, glutamine synthetase; HCV, hepatitis C virus; NASH, non-alcoholic steatohepatitis.
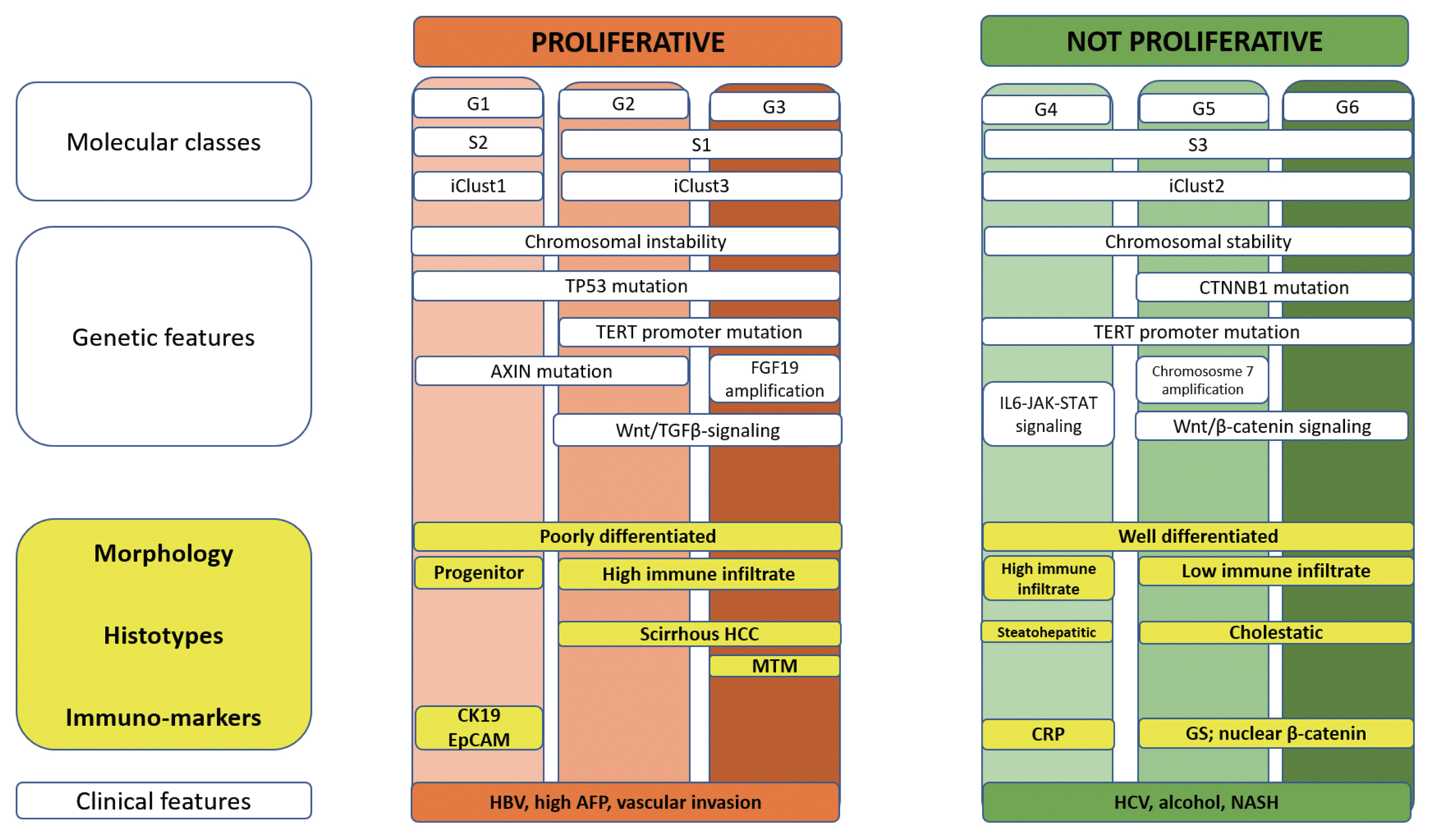
Figure 3
Vascularization of hepatocellular carcinoma (HCC) (CD34 immunohistochemistry, original magnification ×20). (A) Approximately 40% of HCC cases are characterized by a continuous layer of CD34+ vessels encapsulating clusters of neoplastic cells, featuring a peculiar vascular phenotype described as VETC. (B) In the remaining cases, CD34 staining reveals a capillary distribution of vessels.
