Abstract
General anesthesia is critical for various procedures and surgeries. Despite the widespread use of anesthetics, their precise mechanisms remain poorly understood. Anesthetics inevitably act on the brain, primarily through the modulation of target receptors. Even if the action is specific to an individual neuron, however, long-range effects can occur due to the tremendous interconnectedness of neuronal activity. The strength of this connectivity can be understood using mathematical models that allow for the study of neuronal connectivity dynamics. These models also allow researchers to develop hypotheses on the candidate mechanisms of action of different types of anesthesia. This review highlights the theoretical background associated with the study of the mechanisms of action of anesthetics. We propose a candidate framework that describes how anesthetics act on the brain and consciousness in general.
General anesthesia is critical for surgical and invasive procedures. In Korea, more than one million anesthetic cases are performed annually. However, the concept of general anesthesia remains somewhat vague. A prevailing opinion likens general anesthesia to sleep, assuming that general anesthesia is equivalent to ‘sleeping anesthesia’. Another belief is that general anesthesia is similar to unconsciousness. However, general anesthesia is not a lack of consciousness, since unconsciousness is not a phenomenon induced solely by general anesthesia.
The concept of consciousness is vague and difficult to describe. A conscious state is frequently associated with wakefulness, awareness, and consciousness. General anesthesia is associated with a reversible loss of consciousness, sensory function, and autonomic reflexes. It includes hypnotic, antinociceptive, immobility-related, and reflex block components. Among these components, hypnosis is the main one characterizing a general anesthetic state and is closely interwoven with consciousness. In clinical practice, monitors used to quantify the depth of hypnosis are widely used to maintain an acceptable range of general anesthesia. For example, bispectral index monitors can titrate the proper anesthetic depth to improve outcomes during the perioperative period. Despite the widespread use of hypnotic monitors in clinical practice, the mechanisms by which hypnosis serves as an anesthetic are not well understood. In this review, we briefly review the hypotheses that describe the underlying candidate mechanisms of anesthesia, focusing on the hypnotic component of general anesthesia.
All general anesthetics enter the systemic circulation and, hence, the cerebrovasculature. For an anesthetic to have an effect, it must interact with the brain. Meyer-Overton predicted that the potency of anesthetics correlates with lipid solubility [1]. According to the lateral pressure hypothesis, the lipophilicity of an anesthetic allows for it to affect the lipid membrane environment in which protein receptors reside, leading to a perturbation of receptor activity [2]. In terms of maintaining consciousness, receptors can be divided into two classes that are either inhibitory or excitatory to neuronal activity. Augmenting inhibitory receptors or inhibiting excitatory receptors can lead to the impairment of consciousness and other functional activities (perception and memory). Among the receptors in the brain, the gamma-aminobutyric acid (GABA) receptor is the representative inhibitory receptor. Many subtypes of GABA have been identified. Despite the difference in subunits, this receptor is common in heteromeric transmembrane protein complexes that respond to GABA binding, which increases postsynaptic inhibitory currents [3]. Many anesthetics augment the affinity of GABA to its receptor and reduce the possibility of postsynaptic membrane excitation. These inhibitory actions may be associated with behavioral actions (e.g., sedation, amnesia, and anesthesia), which are commonly observed after anesthesia administration. In contrast, glutamate receptors are associated with fast excitatory neurotransmission. N-methyl-d-aspartate (NMDA), alpha-amino-3-hydroxy-5-methyl-4-isoxazole propionic acid (AMPA), and kainite are representative examples of glutamate receptors. These receptors are closely associated with behavioral activities, such as perception, memory, and learning, which represent cognitive functional activity [4,5].
General anesthetics are broadly classified into two administration routes: intravenous and inhalation. Unlike inhaled anesthetics, intravenous anesthetics have hypnotic effects involving less muscle suppression. In addition, each category of anesthesia exerts different actions on receptors. For example, most intravenous anesthetics, such as propofol, etomidate, and barbiturates, act almost exclusively on GABA type A receptors, and rarely act on glycine receptors or potassium channels. Most intravenous anesthetics also involve less muscle suppression compared to inhaled anesthetics.
However, one exception is ketamine, an intravenous anesthetic that mainly acts on NMDA along with other types of receptors. This difference in the primary receptor may explain the unique action of ketamine as an anesthetic.
In contrast to intravenous anesthetics, inhaled anesthetics exhibit different pharmacological actions with greater muscle-suppressing activity. Inhaled anesthetics modulate various types of receptors [6], for example, many inhaled anesthetics augment glycine interactions with its receptor, conferring immobility even in the presence of noxious stimulation. Like intravenous anesthetics, inhaled anesthetics act on the GABA A receptor to produce a hypnotic effect.
The inhibitory actions of anesthetics on receptors are not limited to individual neurons since each neuron is closely connected to other neurons by design so that information can be quickly relayed throughout the nervous system. Hence, anesthetics that affect the receptor activity of a specific neuron will, in turn, modulate both local and long-range connections in the brain (Fig. 1).
General anesthetics have a global effect on the brain. Hence, every brain region could play a role in overall consciousness. The breakdown of consciousness resulting from anesthesia may be due to functional alterations of neural substrates in specific regions. The brain regions that have been studied as potential neural correlates of consciousness include the cerebral cortex, thalamus, brainstem, and basal forebrain (Fig. 2).
A concrete definition of consciousness remains elusive. Two components, arousal and awareness, are deemed essential to describe consciousness [7]. Awareness, which is associated with frontoparietal brain regions, is related to the content of consciousness.
The frontal cortex plays a crucial role in cognitive and executive control. The role of the frontal lobe can be interpreted from lesion data. Massive frontal cortex damage encompassing the majority of the left and right hemispheres impairs cognitive function. Interestingly, even with such damage, perceptions and consciousness are maintained [8]. Other lesion data suggest that even with extensive damage to the bilateral frontal lobe, consciousness is preserved despite a disturbance in cognitive function [9]. These findings suggest that the frontal lobe acts to modulate rather than directly participating in consciousness.
Meanwhile, most patients with extensive bilateral parietal damage, including the corpus callosum, enter a vegetative state [10]. After cardiopulmonary resuscitation, the lesions in this area are closely linked to a permanent coma [11]. Additionally, lesions in the parietal cortex inhibit the perception or recognition of this deficit [12,13].
The default mode network (DMN) and executive control network (ECN) play critical roles in cognitive activities. These networks may be essential for attention and working memory, among other activities. The DMN comprises several brain areas encompassing the prefrontal and parietal areas, such as the anterior and posterior cingulate cortex and the inferior parietal lobe [14]. Interestingly, the DMN is most active when the brain is in a resting state, indicating that the DMN is suppressed by the cognitive activities necessary to facilitate information processing [15–17]. The DMN may be involved in task-negative functions such as self-referent thoughts [18,19]. However, recent studies have suggested a link between this network and various cognitive activities [20]. The ECN is closely related to executive functions that are critical for cognitive functions of daily living. The ECN is also implicated in the integration of sensory and memory information and the processing of working memory [21]. The main component of the ECN is located at the gyri and spreads from the frontal to the parietal area, such as the prefrontal, post-parietal, and anterior cingulate cortices [22]. The ECN is also connected to the DMN and frontoparietal network and plays a crucial role in behavioral functions [21]. Interestingly, disturbances in the DMN and ECN are related to the pathophysiology of impairment that results from cognitive diseases [21,23].
The thalamus is located in the forebrain, which is superior to the midbrain. With connections to the cerebral cortex, midbrain, and brain stem, the thalamus receives sensory information from the periphery and relays those signals to the cortex. Given its widespread connections throughout the brain, the thalamus has been implicated in other behavioral functions, such as maintaining consciousness.
A considerable body of thalamic lesion data supports this implication that the thalamus plays a critical role in consciousness. Thalamic neuronal death is the most common cause of entering a vegetative state after multiple brain injuries [24], and a permanent vegetative state persists in the presence of bilateral thalamic damage. In addition, the pathological rhythms produced in the thalamus may be associated with global cerebral dysfunction [25]. These lesion data suggest that the thalamus plays a vital role in the conscious state [26,27]. Electrical activation of the thalamus disrupts the synchronization of anesthesia-related slow waves, suggesting that thalamic activation may reverse anesthesia-related electroencephalogram (EEG) signatures. Given the widespread connectivity of the thalamus to other brain areas, the modulation of thalamic activity may lead to a disturbance of local and global thalamocortical and thalamic-subcortical interactions, leading to impaired consciousness.
A crucial element of consciousness is arousal at the level of vigilance. The brainstem and forebrain are associated with the brain arousal system. The cholinergic system of the brainstem is implicated in regulating the activities of thalamocortical and cortical neurons. The pedunculopontine/laterodorsal tegmental nuclei are connected to thalamic neurons via projections of the cholinergic pathway. The nucleus basalis projects cholinergic fibers to the cortex and thalamus. This cholinergic system activates the thalamic and cortical neurons closer to the threshold, increasing the probability of switching on these neurons. Collectively, these observations suggest that the brainstem affects consciousness by regulating upstream thalamic and cortical activity.
In the brainstem, the reticular formation also plays a role in consciousness, as it is connected to the intra-thalamic nuclei, which are critical for thalamocortical connections. In a classic experiment, electrical stimulation of the reticular formation elicited EEG patterns in both an awake and attentive state. Additionally, lesions in this region have been shown to diminish consciousness [28]. Indeed, the reticular formation constitutes the core of the reticular activating system responsible for maintaining an alert state.
The forebrain, which consists of both a non-cholinergic and cholinergic neural population, is also implicated in cognitive behavior and wakefulness [29–33]. Basal forebrain cholinergic neurons regulate cortical activity through connections with cortical pyramidal cells [34]. The inhibition of forebrain neurons resulting from antagonists induces behavioral unresponsiveness, followed by EEG changes that are distinct from wakefulness [35,36]. Additionally, damage to this region is linked to disorders associated with cognitive impairment and attention deficits. Additional evidence suggests a link between forebrain lesions and Alzheimer’s-related dementia [37].
The brain regions implicated in consciousness have close physical connections that are unidirectional or bidirectional. This suggests that through modifying and strengthening connections and iteratively tuning into the signals, the information relayed from the outside world is interpreted by referencing it to the relevant area. According to the receptor theory of anesthetic action, the simple up- or downregulation of receptor activity does not directly modulate consciousness.
Considerable research on anesthesia mechanisms of action has focused on how anesthesia integrates or breaks down functional connections between brain regions. Analytical techniques have been introduced to explain the mechanism of general anesthesia, with a primary focus on general anesthesia-induced disturbances in intracerebral communications.
A primary analytical technique is the information integration theory proposed by Tononi [38]. As previously mentioned, consciousness may require the local and global integration of neurons within the brain. Tononi proposed a mathematical model to quantify the information integration of subsets within the consciousness system. In brief, if subset A has causal effects on subset B, regardless of independent noise sources, the measured entropy shared by the source (A) and the target (B) is necessarily due to the causal effects. Therefore, sufficient information on the reciprocity of entropies between subsets A and B can be obtained. In this sense, after searching out bi-partitioned subsets with the least effective information, each subset has more causal interactions. Thus, for each subset, information integration is defined as mutual information for bipartition with minimal information shared between them, which is called minimum information bipartition. For more information on the mathematical calculations involved in information theory, see the review conducted by Tononi [38].
To identify the causal interactions between two information brain regions of interest, cognitive binding and unbinding have been studied. This line of research attempts to explain consciousness and mechanisms associated with general anesthesia using principles that are different from information integration theory, and is based primarily on the theory of Granger causality [39]. In brief, if X1 is known to cause X2, knowledge of past information regarding both X1 and X2 should lead to an improvement in the prediction of X2 compared to the inclusion of only the past information of X2. From a mathematical perspective, if the prediction errors of a model that incorporates past information of both X1 and X2 are significantly lower than those of a model that incorporates only past information of X2, then X1 is causally linked to X2. Such an analysis is performed not only in time series, but also in the frequency domain.
Previous studies have demonstrated that neural oscillations play a critical role in coordinating brain function [40]. Synchronized oscillations characterize the rhythmic activations of local neural ensembles [41] and facilitate information transfer by promoting long-range synchronization within the connected brain network [42]. The approaches are essentially subdivided into directed and non-directed approaches. Non-directed connectivity metrics do not consider the direction of influence; instead, they only capture the coordination between signals, while directed connectivity metrics first establish a statistical inference to identify causation preceding the activity of other areas. Representative analytical approaches include Granger causality, transfer entropy, and dynamic causal modeling for directed analyses (Fig. 3A) and the coherence and phase lag index for non-directed analyses (Fig. 3B).
Despite the differences in the mathematical assumptions and approaches, both methods employ analytical methods to quantify the causal interactions between two regions of interest. Metrics for evaluating these causal interactions are well-summarized in the review conducted by Bastos and Schoffelen [43].
Neural systems have features analogous to phase transitions associated with thermodynamic changes. The framework of criticality, observed as phase transitions in systems, offers functional benefits from which information transmission and dynamic range are optimized in a cortical network [44]. Researchers have recently focused on critical dynamics that are not fully captured by other analytic approaches to decipher state features of the brain [45,46]. The perturbation of a dynamic system close to criticality, which enables long-range communication within the system, does not lead to instability. In contrast, in a dynamic system that is far from criticality, information integration does not occur beyond the point at which the perturbation becomes too dampened. Neuronal activity in the awake state is marginally stable, close to the bifurcation point of a dynamic neural system. However, the dynamic system becomes more stable with the onset of anesthesia [47].
The brain can be considered a well-organized, massive neural network. Hence, some studies have reported the use of network theory to explain the mechanisms of anesthesia. However, it is incredibly challenging to predict how complex networks behave because of the myriad of connections and uncertainties that make explaining causative effects difficult. Complex network theory, a branch of statistical analysis for highly disordered and heterogeneous systems, is an effective tool for understanding macroscopic behavior through analyzing interactions at the microscale [48]. For example, graphical analyses have been used to explore the properties of the brain as a complex network [49]. Within the framework of a network system, the brain can be studied as a vast collection of microscopic and macroscopic connections. Graph theory does not consider the anatomy of the brain; instead, it considers the topology as element sets with some relationship. The edge subsets connect sets of nodes in graph models. Some brain network features can be calculated from edge properties, such as strength and direction (Fig. 3C). Compared to random graphs, the brain has a short path length defined by the number of steps necessary to travel between two nodes across a network, giving rise to high global efficiency. In addition, the brain is associated with considerable clustering, which is defined as the proportion of motifs with triangular shapes connecting three nodes. Unlike a random graph, the brain has a hub structure with highly dense connections [50–53]. Thus, the application of graph theory allows us to investigate the mechanism of general anesthesia by comparing changes in network topology.
Another approach to explain the integration of peripheral stimuli is evoked response potentials. Visual and auditory stimuli play a critical role in maintaining an alert state among other stimuli within our environment. Such stimuli may be important contributors to the neural substrate of consciousness. Although many evoked potentials (EP) have been developed and applied in clinical practice for diagnostic purposes, the auditory evoked potential is widely used in studies of anesthetic mechanisms. During anesthesia, the response to auditory stimuli is well preserved [54], and it is straightforward to apply auditory stimuli to patients. Auditory evoked potentials with short latencies indicate brainstem function, while those with long latencies (> 10 ms) reflect cortical function. The event-related potential (P300 or P3 component) is widely used to evaluate cortical function (Fig. 3D). By examining the components of late EP, estimates of how the brain interacts with and processes stimuli in an integrated manner can be made.
Thus, if we can investigate anesthetic-related changes in late EPs, we can study how the integration and processing of sensory input to the brain is disturbed under general anesthesia. As expected, EPs are significantly altered in the presence of cognitive impairment [55,56], suggesting that the capability of the brain as an information integrator is also impaired as consciousness is disturbed.
To explain the modulation of consciousness induced by propofol in primates, some authors utilize the concept of a dynamically critical regime, describing the change in conscious states as a physical phase transition. The brain is considered a nonlinear system with an inherent instability given the quasi-equilibrium that temporarily attracts dynamics [57]. Propofol makes this system more stable and, hence, less responsive to perturbations [58].
Additionally, cortico-cortical interactions, especially frontoparietal interactions, have gained attention as neural signatures of consciousness [59,60]. As propofol induces loss of consciousness, it selectively impairs feedback frontoparietal connectivity while preserving forward connections [61]. The decrease in connectivity in the frontoparietal network correlates with an increasing concentration of propofol. Some brain networks are called resting-state consciousness networks that are active even when specific tasks are not being performed. Propofol affects the DMN implicated in unconstrained cognitive activities, including self-awareness [62]. The default mode and executive control networks are impaired during propofol-induced loss of consciousness within the frontoparietal network [63]. Additionally, some studies have suggested that frontal network disconnections are the most prominent, resulting in loss of responsiveness [64].
In contrast to the decrease in long-range interactions caused by propofol, local inhibitory connections (self-connections) are enhanced with propofol. For example, as propofol induces loss of consciousness, effective connectivity from the temporal lobe to the frontal lobe is significantly decreased in the presence of an auditory task, presumably due to increased local inhibitory connections in the temporal lobe. Propofol-induced disintegration within the brain has been observed from a temporal (rather than spatial) viewpoint. Propofol-induced local network alterations precede changes in long-range connections, and propofol prolongs the timescales of local interactions, thus impairing connections between distant brain regions.
The breakdown of functional connectivity is not limited to cortico-cortical interactions during propofol-induced unconsciousness, as cortico-thalamic connectivity is also reduced in proportion to the propofol concentration. A decrease in connectivity between the thalamus and the DMN and ECN has been observed in neuroimaging studies [65]. This decrease could be attributed to the reduction of thalamic metabolism that occurs during general anesthesia [66]. However, thalamic connectivity between lower-order networks is preserved during propofol-induced unresponsiveness [67].
In addition to the thalamus, propofol-induced unconsciousness affects the connectivity between the brainstem and the cortex [65,67]. During propofol-induced anesthesia, a significant decrease in connections between the posterior cingulate cortex and precuneus, which are critical areas comprising the DMN, and the brainstem were observed [65]. Considering the importance of the brainstem as a critical element of the reticular arousal system, the anesthetic effect of propofol may be partially due to the inhibition of the arousal mechanism.
Some studies have investigated how propofol disrupts the topology of the brain network. In the awake state, the brain can be modeled as a set of hub structures with high disassortativity, defined as a preference for connections between high-degree and low-degree nodes. Propofol reduces the degree of disassortativity and disrupts the hub structure of the brain, thus randomizing the connections between the nodes and impairing the efficiency of information transfer [68,69]. Propofol-induced unconsciousness is associated with increased clustering and characteristic path length, resulting in a less efficient network. Clustering increases the localization of processing rather than information integration across the brain, thus impairing the efficiency of the brain network [70]. In addition, disturbances in network properties are accompanied by alterations in activity in different frequency bands and states of consciousness [71]. During the transition between responsive states, beta power plays a crucial role. At the same time, delta-band changes are prominent during the fading of consciousness. As consciousness transitions towards an unconscious state, connectivity in the low-frequency band increases, while connectivity in high-frequency bands decreases.
Sedation differs from general anesthesia. As the level of sedation deepens, it eventually leads to a transition from wakefulness to an unconscious state. However, subjects appear to be awake under light sedation, but they are likely not fully responsive to stimuli. Data from numerous studies suggest that sedation is associated with functional states that differ from the states of wakefulness and anesthesia [55,56,72,73]. As the sedation depth increases, functional connectivity patterns are more tied to anatomical connectivity and more rigid functional dynamics with fewer small-world properties and information capacity [72].
Some studies have focused on what changes occur within the brain during the transition from consciousness to unconsciousness or vice versa. As the sedation depth increases, especially when transitioning from moderate to deep sedation, loss of consciousness occurs. Instead of sedation depth preserving wakefulness, deep sedation accompanying loss of consciousness represents a different repertoire of consciousness. Propofol-induced moderate sedation is a different state from either the awake or unconscious states.
The resultant effect of using an established local-global deviant scheme [55] with auditory stimulus during propofol sedation is narrower compared to the anesthetic state in both the non-core and core auditory cortex. In the anesthetized state, the distribution is much wider. Thus, sedation represents a limited repertoire for auditory processing compared to wakefulness, despite a more flexible processing capability from the perspective of novel auditory sound processing and perception [56]. The degree to which the stimulus response is impaired increases with an increasing depth of sedation. During moderate sedation, while in a conscious state, long-range communication is preserved with some impairment [73]. Thus, although propofol blocks information integration in a state of sedation, the overall effect of propofol is considerably decreased during sedation than during general anesthesia.
Unlike other types of general anesthetics, ketamine has a unique mechanism of action, as it interacts with the NMDA receptor. As a result, it possesses strong antinociceptive activity and stimulates both the respiratory and cardiovascular systems.
Despite this different mechanism of action, ketamine shares similar features with other hypnotics in terms of functional connectivity disintegration. Ketamine disturbs the connection from the frontal to the parietal lobes, similar to other hypnotics [58,74]. Additionally, ketamine dissociates the frontal lobe from the other components of the DMN [75], while the executive network and other sensory networks are minimally impaired [75]. Preservation of the executive network during ketamine infusion is a crucial difference from propofol, which usually impairs this network [61]. Interestingly, ketamine also preferentially decreases cortico-cortical interactions, but not thalamocortical interactions. Studies have even shown that ketamine increases thalamocortical interactions [76]. Ketamine can also reorganize the within-brain network, resulting in a psychomimetic effect [75].
Many studies have suggested that criticality is the main feature of resting-state brain networks [77], and ketamine perturbs the topology of a brain network at criticality. Ketamine-induced disruption of the topographic structure is similar to that of other anesthetics and conditions involving impaired consciousness [78]; however, ketamine induces a different EEG response to external stimuli. When transcranial magnetic stimulation is elicited, a series of recurrent, fast-activated waves are generated, which contrasts with the local positive-negative wave (characterized by a rapidly faded low amplitude and no propagation) that exists during propofol anesthesia [79]. Considering that the local positive-negative wave indicates the presence of inhibitor gates for cortico-cortical interactions, this may suggest that ketamine is ineffective at thoroughly disrupting cortico-cortical interactions.
When ketamine is being administered as an anesthetic, frontal-to-parietal connectivity is significantly reduced, especially at the alpha frequency band; however, during ketamine sedation, this connectivity is preserved, although the spectral power is shifted from the alpha to the theta frequency band [80]. The same research group also determined that subanesthetic ketamine reduces the power contained within the alpha frequency band in the precuneus and temporoparietal junction, which is implicated in sensory integration and consciousness [81]. From the viewpoint of information integration, the signals in the theta and alpha frequency bands comprise the bidirectional information flow between the anterior and posterior regions [82,83]. Thus, the ketamine anesthetic effect may originate from the disruption of the breakdown of the resting-state brain network, and the psychomimetic effect may be due to disrupted sensory integration.
Unlike other hypnotics, dexmedetomidine acts on the alpha-2 adrenergic receptor. Its hypnotic effect resembles natural sleep, with an EEG pattern associated with stage 2 non-rapid eye movement sleep (N2) arising from the inhibition of noradrenergic transmission from the locus coeruleus to the ventral preoptic nucleus, leading to cortical arousal system inhibition.
During dexmedetomidine administration, the network topology is disturbed. Dexmedetomidine significantly reduces the efficiency of local and global networks within the brain network, suggesting that information transfer is impaired during sedation with dexmedetomidine [84]. In addition, auditory processing is significantly impaired [85], which is consistent with the alterations in network properties that are observed. From the viewpoint of connectivity, during dexmedetomidine sedation, functional connectivity within higher-order networks (e.g., the DMN and ECN) and between the thalamus and higher-order networks decrease [65]. However, unlike propofol, the connectivity between the anterior cingulate cortex/prefrontal area, thalamus, and mesopontine area are preserved. Given the importance of these regions for enabling quick recovery of the brain, this connectivity could explain why patients respond to stimuli quicker during dexmedetomidine sedation compared to propofol sedation.
Intravenous and inhaled anesthetics are the two primary types that are widely used in clinical practice. Inhaled anesthetics allow for control of hypnosis, sensory/motor blocks, and autonomic reflexes in a dose-dependent manner. To meet the anesthesia demands of surgery, anesthesiologists modulate the anesthetic concentration levels by controlling the vaporizer. Despite its widespread use, the mechanism of action of inhaled anesthetics remains unclear.
Classic experiments exploring the mechanisms of inhaled anesthetics have focused on isoflurane. Inhaled anesthetics are classified as GABA agonists because they enhance the interaction of GABA with its receptors. Although some differences have been noted, inhaled anesthetics in general act on the GABA receptor, similar to hypnotics such as propofol. Isoflurane inhibits metabolism in the thalamus and disrupts both thalamocortical connectivity [86,87] and connectivity between the anterior and posterior cortical areas [88]. Additionally, during isoflurane anesthesia, the brain tends to be in an overall state that is less complex and efficient, with a decreased sensitivity to external stimuli [78]. Since GABAergic interneurons play a significant role in modulating cortico-cortical connections [89], GABAergic effects may disrupt functional connectivity.
During sevoflurane anesthesia, feedback connectivity from the anterior to posterior regions is inhibited (as with propofol) and feedforward connectivity is preserved [58]. Sevoflurane at a 2–3 vol% concentration is associated with a significant reduction in directed anterior-to-posterior functional connectivity in the frontal and anterior parts of the DMN and a reduction in thalamocortical functional connectivity [90]. During sevoflurane-induced unconsciousness, disruptions of the phase relationship between the anterior and posterior regions have been noted [91]. Despite consistent results for the concentrations of sevoflurane used for general anesthesia, results are contradictory among studies involving sevoflurane sedation. Even at sub-anesthetic sevoflurane concentrations, intracortical functional connectivity is disturbed. However, functional connectivity within the DMN is either unaltered [92] or reduced [93] during sevoflurane sedation.
Sevoflurane has a significant effect on EP. Auditory and task-evoked brain activities are disturbed in various brain areas (auditory, visual, and motor cortex) [94,95]. This perturbation has also been seen at subanesthetic concentrations, at which the brain’s ability to discriminate odd stimuli from background stimuli is disturbed [96]. This finding indicates that sevoflurane disturbs the processing of external stimuli, which may be attributed to the disturbance of cerebral networks, making the brain less reactive to stimuli-induced perturbations.
Compared to other inhaled anesthetics, nitrous oxide has distinctive features. First, instead of the GABA receptor, it acts on the NMDA receptor. Additionally, its potency is insufficient for use as the sole agent in general anesthesia. Instead, nitrous oxide has been widely used for sedation, especially in dental practice. The low blood-gas partition coefficient of nitrous oxide leads to rapid induction and recovery, making it widely applicable as a sedative. Like other inhaled anesthetics, nitrous oxide impairs intracortical connectivity, primarily at the alpha frequency bands implicated in cognition [97]. During nitrous oxide sedation, the efficiency of information transfer is significantly disturbed owing to alterations in the network topology [64]. Similar to other anesthetics, the sedation effect inevitably accompanies changes in functional interactions as well as the capacity for global and local information integration.
The cognitive binding/unbinding paradigm expands our understanding of consciousness [38,39]. This paradigm is based on the concept that consciousness can respond to external stimuli through iterative integration within the brain. Traditionally, many attempts have been made to understand anesthesia in a simplified manner. For example, it is widely known that general anesthetics change the spectral power at both low-and high-frequency bands. As the depth of anesthesia increases, the power of low-frequency bands, such as delta and theta, increases, while that of high-frequency bands decreases. Many devices for monitoring the depth of anesthesia are based on anesthetic-related spectral power changes, especially in the frontal area [98]. However, spectral changes in localized brain regions do not fully describe how anesthesia works. These changes provide only a fraction of the information on whole-brain interactions. Studying the dynamic interactions between different brain areas during general anesthesia has substantially advanced our understanding of the mechanism of action of anesthetics. Several topics should be considered when interpreting the findings of previous studies on anesthetic mechanisms.
As described above, regardless of the type of anesthetic agent, disturbances in functional connectivity between regions of interest are observed as anesthetic effects occur. However, the possibility of other sources affecting these disturbances cannot be excluded. For example, most anesthetics disturb the functional connections between the anterior and posterior brain areas; however, these areas are connected to other brain areas. If other areas outside the analyzed regions of interest have a real effect on both sides, the observed causal interactions might be spurious. Researchers have used mathematical analytical methods with a directed approach to identify causal interactions related to anesthetic effects to eliminate such spurious interactions [43]. Network or state-based approaches can be used to observe either connections or system features as they change in the brain as a whole and may help circumvent this limitation. Manipulating specific brain sites with optogenetics in a precisely controlled manner may also help us understand the role of specific areas in anesthetic mechanisms [99].
In addition, considerable effort should be made to avoid the acquisition of signals contaminated by external artifacts when acquiring data. For example, noise sources (e.g., electrical noise and muscle artifacts), which are more prominent during general anesthesia in the operating room, must be minimized during EEG acquisition. If such artifacts are not correctly identified, the likelihood of spurious causal interactions increases.
Brain measurements typically involve EEG and/or neuroimaging modalities, such as magnetic resonance imaging and positron emission tomography. Due to the high spatial resolution of neuroimaging data, we can easily detect which regions are related to anesthetic activity. Regions of interest with changes during anesthesia compared to baseline can be easily tracked by applying relevant mathematical approaches. However, neuroimaging modalities have a low temporal resolution, making it challenging to detect time-varying signals. Furthermore, routine neuroimaging in patients under general anesthesia in real-time is extremely difficult to perform.
In contrast, EEG has a high temporal resolution, allowing practitioners to identify rapidly changing brain states and apply it to patients in the operating room. However, due to its poor spatial resolution, it is difficult to localize the changes in activity associated with anesthetic changes to specific regions of interest. Although mathematical approaches, such as inverse source modeling [100] have been developed to circumvent the limitations of poor spatial resolution, they remain susceptible to various contaminants, such as muscle artifacts. In this regard, a multimodal approach can be utilized to search for neural correlates in both space and time.
Functional near-infrared spectroscopy (fNIRS) may be viable with sufficient spatiotemporal resolution. This technique utilizes infrared light, which is selectively absorbed by oxy- and deoxyhemoglobin and minimally absorbed by other tissue constituents. Oxyhemoglobin has a stronger absorption of light at wavelengths longer than 790 nm, while deoxyhemoglobin has a stronger absorption at wavelengths shorter than 790 nm. Cerebral metabolic activity affects the relative proportions of oxy- and deoxyhemoglobin. A rapid reduction in oxyhemoglobin occurs with local metabolic activity, followed by an increase in total hemoglobin due to a local increase in blood flow. This cerebral response is known as neurovascular coupling [101]. Like EEG, fNIRS can be easily applied to patients under anesthesia; however, with the placement of multiple optical sources and detectors on the scalp surface, a spatial sampling feature can be added. The utility of fNIRS has been well-described in peer-reviewed literature, as previous studies have demonstrated that fNIRS can monitor the depth of anesthesia [102,103]. Combining this modality with EEG may enable a detailed spatiotemporal study of the mechanisms of anesthesia [104].
The mechanism of general anesthesia appears to be a simple phenomenon that results in loss of consciousness. However, in this review, we have described the complexity of the cerebral response to anesthesia. Put simply, we cannot easily distinguish between deep sedation and light general anesthesia. The mechanisms of action differ according to anesthesia type, and the response to anesthetic drugs shows considerable variation even with similar administered drug concentrations. Furthermore, the demographics of patients entering the operating room for anesthesia are extremely heterogeneous. Additionally, there are many clinical situations that present similar to general anesthesia. For example, patients that are asleep, in a vegetative state, and in a coma, all resemble an anesthetic state because the subjects are poorly responsive to external stimuli.
It is reasonable to consider whether there is a common pathway leading to unconsciousness for hypnosis. Numerous studies have not supported the concept of a unitary pathway to loss of consciousness irrespective of the type of impaired consciousness [105,106]. Although loss of consciousness is phenomenologically simple, various pathways to modulate consciousness exist. Additionally, few studies have compared the anesthetic mechanisms between healthy subjects and those with neurological conditions, such as intellectual disability, traumatic injury, and those in a coma. It is likely that the onset of and recovery from anesthesia differ depending on the neurological status. For example, bispectral index values differ between patients with and those without intellectual disabilities [107,108]. Additionally, intellectually disabled patients exhibit different patterns of recovery from general anesthesia [109]. Since the algorithm embedded in depth of anesthesia monitors is primarily based on changes in spectral power compared to healthy patients, the values may be abnormal when the monitors are applied to patients with neurological conditions that affect baseline EEG data.
Spectral power measured solely in one region does not represent functional brain status on a global scale. Hence, spatially localized measurements may not fully reflect anesthesia-induced alterations in functional configurations. To obtain a deeper understanding of the mechanisms of anesthesia, researchers must better understand the spatiotemporal relationships of cerebral activity at different stages of anesthesia. For example, a new quantitative index incorporating the phase relationship between the frontal and prefrontal areas may enable the application of functional connections to measure the depth of anesthesia [110].
General anesthesia induces loss of sensory processing and motor functions, in addition to hypnosis. Antinociception and muscle block are fundamental aspects of any surgical procedure. The effect of neuromuscular blockers can be easily measured using a neuromuscular stimulator. However, the mechanism of anesthesia-induced antinociception remains poorly understood, and monitors to measure the extent of antinociception objectively are not widely available. Commercially available monitors for antinociception are mainly based on changes in autonomic nervous system function due to sympathetic system inhibition. Anesthetics may affect functional connections between the thalamus and sensory cortex, which changes with anesthetic depth [87]. Given that the psychomimetic effects of ketamine seem to arise from a reorganization of sensory processing within other regions [75], it seems plausible that the alterations of sensory networks could give rise to antinociception during anesthesia. However, further studies are required to clarify this issue.
Countless resources have been put into exploring what the brain is and how it is structurally and functionally organized. General anesthesia can be a powerful experimental tool. The identification of regions that are missing or impaired during general anesthesia can help in the identification of functional configurations that are critical for cognitive activities.
Considering that procedures requiring general anesthesia are ubiquitous worldwide, the potential for multimodal data collection using technologies such as EEG and fNIRS could lead to the accumulation of large datasets that could then be used to search for neural correlates of cognitive activities. Hence, anesthesiologists could play a significant role in improving our overall understanding of the brain. The development of objective markers to measure changes in hypnotic and nociceptive levels will, in turn, lead to innovative analytical tools and techniques that are expected to impact studies of brain function and health.
References
1. Missner A, Pohl P. 110 years of the Meyer-Overton rule: predicting membrane permeability of gases and other small compounds. Chemphyschem. 2009; 10:1405–14.


2. Cantor RS. The lateral pressure profile in membranes: a physical mechanism of general anesthesia. Toxicol Lett. 1998; 100:451–8.


3. Sivilotti L, Nistri A. GABA receptor mechanisms in the central nervous system. Prog Neurobiol. 1991; 36:35–92.


4. Kalia LV, Kalia SK, Salter MW. NMDA receptors in clinical neurology: excitatory times ahead. Lancet Neurol. 2008; 7:742–55.


6. Campagna JA, Miller KW, Forman SA. Mechanisms of actions of inhaled anesthetics. N Engl J Med. 2003; 348:2110–24.


8. Markowitsch HJ, Kessler J. Massive impairment in executive functions with partial preservation of other cognitive functions: the case of a young patient with severe degeneration of the prefrontal cortex. Exp Brain Res. 2000; 133:94–102.


9. Barrash J, Stuss DT, Aksan N, Anderson SW, Jones RD, Manzel K, et al. “Frontal lobe syndrome”? Subtypes of acquired personality disturbances in patients with focal brain damage. Cortex. 2018; 106:65–80.


10. Kampfl A, Schmutzhard E, Franz G, Pfausler B, Haring HP, Ulmer H, et al. Prediction of recovery from post-traumatic vegetative state with cerebral magnetic-resonance imaging. Lancet. 1998; 351:1763–7.


11. Bianchi MT, Sims JR. Restricted diffusion in the splenium of the corpus callosum after cardiac arrest. Open Neuroimag J. 2008; 2:1–4.


12. George MS, Parekh PI, Rosinsky N, Ketter TA, Kimbrell TA, Heilman KM, et al. Understanding emotional prosody activates right hemisphere regions. Arch Neurol. 1996; 53:665–70.


13. Sirigu A, Daprati E, Ciancia S, Giraux P, Nighoghossian N, Posada A, et al. Altered awareness of voluntary action after damage to the parietal cortex. Nat Neurosci. 2004; 7:80–4.


15. Fox MD, Raichle ME. Spontaneous fluctuations in brain activity observed with functional magnetic resonance imaging. Nat Rev Neurosci. 2007; 8:700–11.


16. Greicius MD, Krasnow B, Reiss AL, Menon V. Functional connectivity in the resting brain: a network analysis of the default mode hypothesis. Proc Natl Acad Sci U S A. 2003; 100:253–8.


17. Greicius MD, Supekar K, Menon V, Dougherty RF. Resting-state functional connectivity reflects structural connectivity in the default mode network. Cereb Cortex. 2009; 19:72–8.


18. Mason MF, Norton MI, Van Horn JD, Wegner DM, Grafton ST, Macrae CN. Wandering minds: the default network and stimulus-independent thought. Science. 2007; 315:393–5.


19. Andrews-Hanna JR, Smallwood J, Spreng RN. The default network and self-generated thought: component processes, dynamic control, and clinical relevance. Ann N Y Acad Sci. 2014; 1316:29–52.


20. Smith V, Mitchell DJ, Duncan J. Role of the default mode network in cognitive transitions. Cereb Cortex. 2018; 28:3685–96.


21. Francx W, Oldehinkel M, Oosterlaan J, Heslenfeld D, Hartman CA, Hoekstra PJ, et al. The executive control network and symptomatic improvement in attention-deficit/hyperactivity disorder. Cortex. 2015; 73:62–72.


22. Damoiseaux JS, Rombouts SA, Barkhof F, Scheltens P, Stam CJ, Smith SM, et al. Consistent resting-state networks across healthy subjects. Proc Natl Acad Sci U S A. 2006; 103:13848–53.


23. Bonnelle V, Leech R, Kinnunen KM, Ham TE, Beckmann CF, De Boissezon X, et al. Default mode network connectivity predicts sustained attention deficits after traumatic brain injury. J Neurosci. 2011; 31:13442–51.


24. Adams JH, Graham DI, Jennett B. The neuropathology of the vegetative state after an acute brain insult. Brain. 2000; 123:1327–38.


25. Kobylarz EJ, Schiff ND. Neurophysiological correlates of persistent vegetative and minimally conscious states. Neuropsychol Rehabil. 2005; 15:323–32.


27. Moruzzi G, Magoun HW. Brain stem reticular formation and activation of the EEG. Electroencephalogr Clin Neurophysiol. 1949; 1:455–73.


28. Mason-Browne NL. Alteration of consciousness: tumor of the reticular activating system. AMA Arch Neurol Psychiatry. 1956; 76:380–7.


29. Beninger R. Activation to acquisition: functional aspects of the basal forebrain cholinergic system. Behav Pharmacol. 1991; 2:530–2.
30. Abe K, Inokawa M, Kashiwagi A, Yanagihara T. Amnesia after a discrete basal forebrain lesion. J Neurol Neurosurg Psychiatry. 1998; 65:126–30.


31. Kilgard MP, Merzenich MM. Cortical map reorganization enabled by nucleus basalis activity. Science. 1998; 279:1714–8.


32. Pinto L, Goard MJ, Estandian D, Xu M, Kwan AC, Lee SH, et al. Fast modulation of visual perception by basal forebrain cholinergic neurons. Nat Neurosci. 2013; 16:1857–63.


33. Goard M, Dan Y. Basal forebrain activation enhances cortical coding of natural scenes. Nat Neurosci. 2009; 12:1444–9.


34. Dunnett SB, Everitt BJ, Robbins TW. The basal forebrain-cortical cholinergic system: interpreting the functional consequences of excitotoxic lesions. Trends Neurosci. 1991; 14:494–501.


35. Portas CM, Thakkar M, Rainnie DG, Greene RW, McCarley RW. Role of adenosine in behavioral state modulation: a microdialysis study in the freely moving cat. Neuroscience. 1997; 79:225–35.


36. Blanco-Centurion C, Xu M, Murillo-Rodriguez E, Gerashchenko D, Shiromani AM, Salin-Pascual RJ, et al. Adenosine and sleep homeostasis in the basal forebrain. J Neurosci. 2006; 26:8092–100.


37. Perry EK, Perry RH, Blessed G, Tomlinson BE. Changes in brain cholinesterases in senile dementia of Alzheimer type. Neuropathol Appl Neurobiol. 1978; 4:273–7.


38. Tononi G. An information integration theory of consciousness. BMC Neurosci. 2004; 5:42.
39. Granger CW. Investigating causal relations by econometric models and cross-spectral methods. Econometrica. 1969; 37:424–38.


40. Siegel M, Donner TH, Engel AK. Spectral fingerprints of large-scale neuronal interactions. Nat Rev Neurosci. 2012; 13:121–34.


42. Wang XJ. Neurophysiological and computational principles of cortical rhythms in cognition. Physiol Rev. 2010; 90:1195–268.


43. Bastos AM, Schoffelen JM. A tutorial review of functional connectivity analysis methods and their interpretational pitfalls. Front Syst Neurosci. 2016; 9:175.


44. Shew WL, Plenz D. The functional benefits of criticality in the cortex. Neuroscientist. 2013; 19:88–100.


47. Alonso LM, Proekt A, Schwartz TH, Pryor KO, Cecchi GA, Magnasco MO. Dynamical criticality during induction of anesthesia in human ECoG recordings. Front Neural Circuits. 2014; 8:20.


48. Boccaletti S, Latora V, Moreno Y, Chavez M, Hwang DU. Complex networks: structure and dynamics. Phys Rep. 2006; 424:175–308.


49. Bullmore E, Sporns O. Complex brain networks: graph theoretical analysis of structural and functional systems. Nat Rev Neurosci. 2009; 10:186–98.


50. Eguiluz VM, Chialvo DR, Cecchi GA, Baliki M, Apkarian AV. Scale-free brain functional networks. Phys Rev Lett. 2005; 94:018102.
51. Achard S, Salvador R, Whitcher B, Suckling J, Bullmore E. A resilient, low-frequency, small-world human brain functional network with highly connected association cortical hubs. J Neurosci. 2006; 26:63–72.


52. Milo R, Shen-Orr S, Itzkovitz S, Kashtan N, Chklovskii D, Alon U. Network motifs: simple building blocks of complex networks. Science. 2002; 298:824–7.


54. Kumar A, Bhattacharya A, Makhija N. Evoked potential monitoring in anaesthesia and analgesia. Anaesthesia. 2000; 55:225–41.


55. Marti S, Thibault L, Dehaene S. How does the extraction of local and global auditory regularities vary with context? PLoS One. 2014; 9:e107227.


56. Nourski KV, Steinschneider M, Rhone AE, Kawasaki H, Howard MA 3rd, Banks MI. Auditory predictive coding across awareness states under anesthesia: an intracranial electrophysiology study. J Neurosci. 2018; 38:8441–52.


58. Alonso LM, Solovey G, Yanagawa T, Proekt A, Cecchi GA, Magnasco MO. Single-trial classification of awareness state during anesthesia by measuring critical dynamics of global brain activity. Sci Rep. 2019; 9:4927.


59. Lee U, Kim S, Noh GJ, Choi BM, Hwang E, Mashour GA. The directionality and functional organization of frontoparietal connectivity during consciousness and anesthesia in humans. Conscious Cogn. 2009; 18:1069–78.


60. Lee U, Ku S, Noh G, Baek S, Choi B, Mashour GA. Disruption of frontal-parietal communication by ketamine, propofol, and sevoflurane. Anesthesiology. 2013; 118:1264–75.


61. Boly M, Moran R, Murphy M, Boveroux P, Bruno MA, Noirhomme Q, et al. Connectivity changes underlying spectral EEG changes during propofol-induced loss of consciousness. J Neurosci. 2012; 32:7082–90.


62. Buckner RL, Andrews-Hanna JR, Schacter DL. The brain’s default network: anatomy, function, and relevance to disease. Ann N Y Acad Sci. 2008; 1124:1–38.
63. Boveroux P, Vanhaudenhuyse A, Bruno MA, Noirhomme Q, Lauwick S, Luxen A, et al. Breakdown of within- and between-network resting state functional magnetic resonance imaging connectivity during propofol-induced loss of consciousness. Anesthesiology. 2010; 113:1038–53.


64. Guldenmund P, Gantner IS, Baquero K, Das T, Demertzi A, Boveroux P, et al. Propofol-induced frontal cortex disconnection: a study of resting-state networks, total brain connectivity, and mean BOLD signal oscillation frequencies. Brain Connect. 2016; 6:225–37.


65. Guldenmund P, Demertzi A, Boveroux P, Boly M, Vanhaudenhuyse A, Bruno MA, et al. Thalamus, brainstem and salience network connectivity changes during propofol-induced sedation and unconsciousness. Brain Connect. 2013; 3:273–85.


66. Lee JM, Kim PJ, Kim HG, Hyun HK, Kim YJ, Kim JW, et al. Analysis of brain connectivity during nitrous oxide sedation using graph theory. Sci Rep. 2020; 10:2354.


67. Guldenmund P, Vanhaudenhuyse A, Sanders RD, Sleigh J, Bruno MA, Demertzi A, et al. Brain functional connectivity differentiates dexmedetomidine from propofol and natural sleep. Br J Anaesth. 2017; 119:674–84.


68. Kim M, Mashour GA, Moraes SB, Vanini G, Tarnal V, Janke E, et al. Functional and topological conditions for explosive synchronization develop in human brain networks with the onset of anesthetic-induced unconsciousness. Front Comput Neurosci. 2016; 10:1.


69. Lee U, Müller M, Noh GJ, Choi B, Mashour GA. Dissociable network properties of anesthetic state transitions. Anesthesiology. 2011; 114:872–81.


70. Monti MM, Lutkenhoff ES, Rubinov M, Boveroux P, Vanhaudenhuyse A, Gosseries O, et al. Dynamic change of global and local information processing in propofol-induced loss and recovery of consciousness. PLoS Comput Biol. 2013; 9:e1003271.


71. Lee M, Sanders RD, Yeom SK, Won DO, Seo KS, Kim HJ, et al. Network properties in transitions of consciousness during propofol-induced sedation. Sci Rep. 2017; 7:16791.


72. Barttfeld P, Uhrig L, Sitt JD, Sigman M, Jarraya B, Dehaene S. Signature of consciousness in the dynamics of resting-state brain activity. Proc Natl Acad Sci U S A. 2015; 112:887–92.


73. Kim PJ, Kim HG, Noh GJ, Koo YS, Shin TJ. Disruption of frontal-parietal connectivity during conscious sedation by propofol administration. Neuroreport. 2017; 28:896–902.


74. Blain-Moraes S, Lee U, Ku S, Noh G, Mashour GA. Electroencephalographic effects of ketamine on power, cross-frequency coupling, and connectivity in the alpha bandwidth. Front Syst Neurosci. 2014; 8:114.


75. Bonhomme V, Vanhaudenhuyse A, Demertzi A, Bruno MA, Jaquet O, Bahri MA, et al. Resting-state network-specific breakdown of functional connectivity during ketamine alteration of consciousness in volunteers. Anesthesiology. 2016; 125:873–88.


76. Höflich A, Hahn A, Küblböck M, Kranz GS, Vanicek T, Windischberger C, et al. Ketamine-induced modulation of the thalamo-cortical network in healthy volunteers as a model for schizophrenia. Int J Neuropsychopharmacol. 2015; 18:pyv040.


77. Wilting J, Priesemann V. 25 years of criticality in neuroscience - established results, open controversies, novel concepts. Curr Opin Neurobiol. 2019; 58:105–11.


78. Lee H, Golkowski D, Jordan D, Berger S, Ilg R, Lee J, et al. Relationship of critical dynamics, functional connectivity, and states of consciousness in large-scale human brain networks. Neuroimage. 2019; 188:228–38.


79. Sarasso S, Boly M, Napolitani M, Gosseries O, Charland-Verville V, Casarotto S, et al. Consciousness and complexity during unresponsiveness induced by propofol, xenon, and ketamine. Curr Biol. 2015; 25:3099–105.


80. Vlisides PE, Bel-Bahar T, Lee U, Li D, Kim H, Janke E, et al. Neurophysiologic correlates of ketamine sedation and anesthesia: a high-density electroencephalography study in healthy volunteers. Anesthesiology. 2017; 127:58–69.
81. Vlisides PE, Bel-Bahar T, Nelson A, Chilton K, Smith E, Janke E, et al. Subanaesthetic ketamine and altered states of consciousness in humans. Br J Anaesth. 2018; 121:249–59.


82. Hillebrand A, Tewarie P, Van Dellen E, Yu M, Carbo EW, Douw L, et al. Direction of information flow in large-scale resting-state networks is frequency-dependent. Proc Natl Acad Sci U S A. 2016; 113:3867–72.


83. Meier J, Zhou X, Hillebrand A, Tewarie P, Stam CJ, Van Mieghem P. The epidemic spreading model and the direction of information flow in brain networks. NeuroImage. 2017; 152:639–46.


84. Hashmi JA, Loggia ML, Khan S, Gao L, Kim J, Napadow V, et al. Dexmedetomidine disrupts the local and global efficiencies of large-scale brain networks. Anesthesiology. 2017; 126:419–30.


85. Frölich MA, Banks C, Ness TJ. The effect of sedation on cortical activation: a randomized study comparing the effects of sedation with midazolam, propofol, and dexmedetomidine on auditory processing. Anesth Analg. 2017; 124:1603–10.
86. Detsch O, Vahle-Hinz C, Kochs E, Siemers M, Bromm B. Isoflurane induces dose-dependent changes of thalamic somatosensory information transfer. Brain Res. 1999; 829:77–89.


87. Cho D, Shin TJ, Ham J, Choi DH, Kim S, Jeong S, et al. Differential modulation of thalamo-parietal interactions by varying depths of isoflurane anesthesia. PLoS One. 2017; 12:e0175191.


88. Imas OA, Ropella KM, Wood JD, Hudetz AG. Isoflurane disrupts anterio-posterior phase synchronization of flash-induced field potentials in the rat. Neurosci Lett. 2006; 402:216–21.


89. Tremblay R, Lee S, Rudy B. GABAergic interneurons in the neocortex: from cellular properties to circuits. Neuron. 2016; 91:260–92.


90. Ranft A, Golkowski D, Kiel T, Riedl V, Kohl P, Rohrer G, et al. Neural correlates of sevoflurane-induced unconsciousness identified by simultaneous functional magnetic resonance imaging and electroencephalography. Anesthesiology. 2016; 125:861–72.


91. Blain-Moraes S, Tarnal V, Vanini G, Alexander A, Rosen D, Shortal B, et al. Neurophysiological correlates of sevoflurane-induced unconsciousness. Anesthesiology. 2015; 122:307–16.


92. Martuzzi R, Ramani R, Qiu M, Rajeevan N, Constable RT. Functional connectivity and alterations in baseline brain state in humans. Neuroimage. 2010; 49:823–34.


93. Deshpande G, Kerssens C, Sebel PS, Hu X. Altered local coherence in the default mode network due to sevoflurane anesthesia. Brain Res. 2010; 1318:110–21.


94. Ramani R, Qiu M, Constable RT. Sevoflurane 0.25 MAC preferentially affects higher order association areas: a functional magnetic resonance imaging study in volunteers. Anesth Analg. 2007; 105:648–55.


95. Kerssens C, Hamann S, Peltier S, Hu XP, Byas-Smith MG, Sebel PS. Attenuated brain response to auditory word stimulation with sevoflurane: a functional magnetic resonance imaging study in humans. Anesthesiology. 2005; 103:11–9.
96. Kim JW, Kim PJ, Kim HG, Shin TJ. Changes of auditory stimulus processing in sevoflurane-induced sedation. Neurosci Lett. 2019; 698:121–5.


97. Ryu JH, Kim PJ, Kim HG, Koo YS, Shin TJ. Investigating the effects of nitrous oxide sedation on frontal-parietal interactions. Neurosci Lett. 2017; 651:9–15.


98. Schneider G, Jordan D, Schwarz G, Bischoff P, Kalkman CJ, Kuppe H, et al. Monitoring depth of anesthesia utilizing a combination of electroencephalographic and standard measures. Anesthesiology. 2014; 120:819–28.


99. Moody OA, Zhang ER, Vincent KF, Kato R, Melonakos ED, Nehs CJ, et al. The neural circuits underlying general anesthesia and sleep. Anesth Analg. 2021; 132:1254–64.


100. Pascual-Marqui RD, Lehmann D, Koenig T, Kochi K, Merlo MC, Hell D, et al. Low resolution brain electromagnetic tomography (LORETA) functional imaging in acute, neuroleptic-naïve, first-episode, productive schizophrenia. Psychiatry Res. 1999; 90:169–79.
101. Chen WL, Wagner J, Heugel N, Sugar J, Lee YW, Conant L, et al. Functional near-infrared spectroscopy and its clinical application in the field of neuroscience: advances and future directions. Front Neurosci. 2020; 14:724.


102. Choi DH, Shin TJ, Kim S, Bae J, Cho D, Ham J, et al. Monitoring cerebral oxygenation and local field potential with a variation of isoflurane concentration in a rat model. Biomed Opt Express. 2016; 7:4114–24.


103. Bae J, Shin TJ, Kim S, Choi DH, Cho D, Ham J, et al. The changes of cerebral hemodynamics during ketamine induced anesthesia in a rat model. J Biophotonics. 2018; 11:e201800081.


104. Vijayakrishnan Nair V, Kish BR, Yang HS, Yu Z, Guo H, Tong Y, et al. Monitoring anesthesia using simultaneous functional Near Infrared Spectroscopy and Electroencephalography. Clin Neurophysiol. 2021; 132:1636–46.


105. Brown EN, Lydic R, Schiff ND. General anesthesia, sleep, and coma. N Engl J Med. 2010; 363:2638–50.


106. Laureys S, Owen AM, Schiff ND. Brain function in coma, vegetative state, and related disorders. Lancet Neurol. 2004; 3:537–46.


107. Choudhry DK, Brenn BR. Bispectral index monitoring: a comparison between normal children and children with quadriplegic cerebral palsy. Anesth Analg. 2002; 95:1582–5.


108. Valkenburg AJ, de Leeuw TG, Tibboel D, Weber F. Lower bispectral index values in children who are intellectually disabled. Anesth Analg. 2009; 109:1428–33.


Fig. 1.
Schematic view of cortico-cortical circuitry. After an anesthetic is administered, it binds to specific receptors and modulates the synaptic function. Consequently, it inevitably affects the transmission of neural signals either downstream or upstream (in this illustration, it acts downstream). On a large scale, even if the anesthetics act on only a single receptor, this leads to the disturbance of interactions between brain areas (in this illustration, the connection between areas A and B is impaired through the impedance of the relay of information). The numbers from I to VI indicate the different cortical layers. The magnified inset indicates the region denoted by the small blue box.
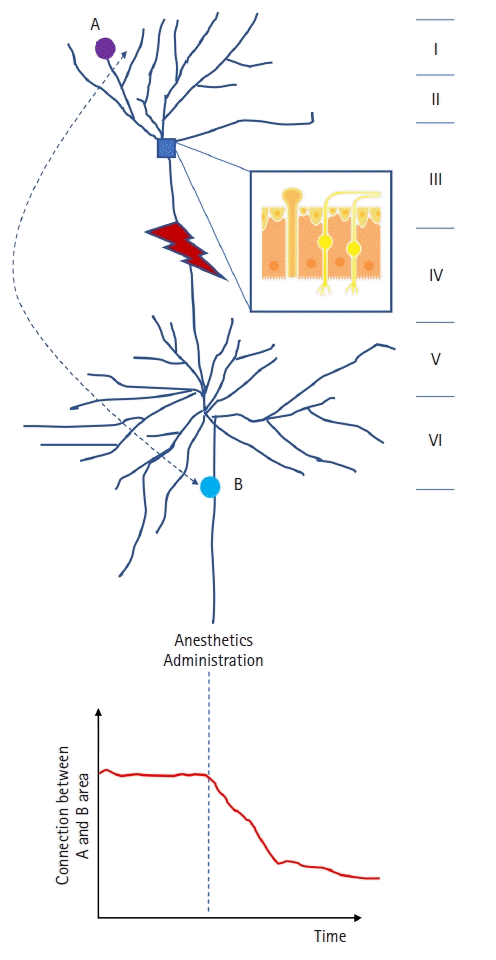
Fig. 2.
Schematic diagram of neural substrates for consciousness. The blue triangle indicates how much the brain network is involved in wakefulness, while the red triangle indicates how much the brain network is involved in awareness. The DMN is more involved in task-free awareness than external awareness responding to the stimuli. RAS: the reticular activating system, DMN: default mode network.
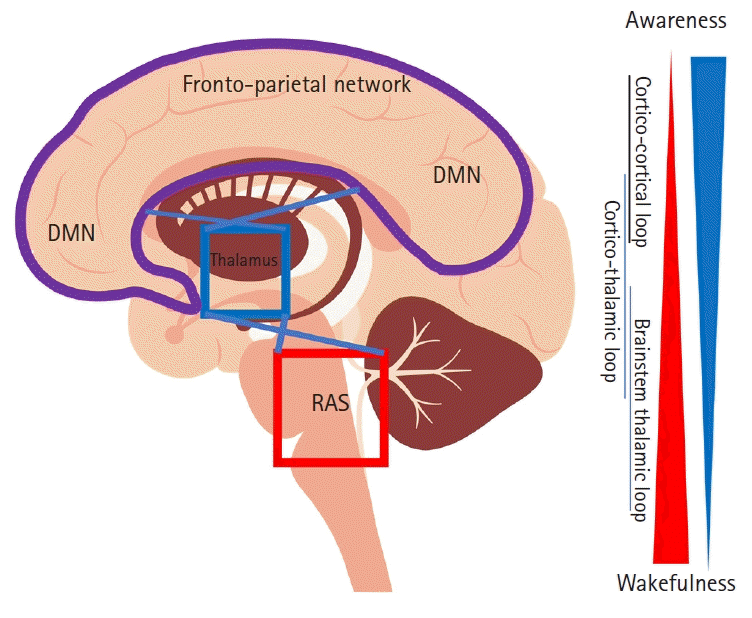
Fig. 3.
Representative scheme of mathematical brain modeling. (A) Granger causality (GC) was obtained between a prediction model with a signal including X1 and X2 and a model only including X2. If you assume the inclusion of X1 improves the prediction of X2, the error will be reduced, so an increase in the value of GC would be expected from the equation. (B) Phase lag index. Time signals are transformed into the frequency domain by applying a Fourier transform. The frequency-domain signals can be displayed in the complex plane, enabling calculation of the amplitude, defined as vector distance, and phase, defined as the angle in relation to the real axis. Two signals from one trial were compared to obtain phase difference (phase lag) with a specific frequency. The phase lag index between the two signals can be calculated by adding up all the trials. If the two signals are phase-coherent, the sum of the phase difference will be augmented without canceling out. (C) Complex network system. The complex network system can be displayed by specific edge relations between two nodes. The brain is represented as nodes and the connections between the nodes. There are many ways to obtain edge relations between two brain nodes. The node differences in a person who is awake (left) vs. under anesthesia (right) can create different networks that explain the differences in information content. The number of edges from red-to-red dots increases on the right side (lower left: the shortest path length is 4 [A → B]; lower right: the path length increases from 4 to 6 [C → D]). (D) Evoked response potential. As event-related potentials accumulate, the signal-to-noise ratio increases because the random noise is canceled. (Left figure) Target stimuli are inserted between the background to obtain twitch responses in the brain signal. The P300, implicated in cognitive processing, shows a different pattern between awake and sedation [see figure in ref 96, which has been approved for reproduction].
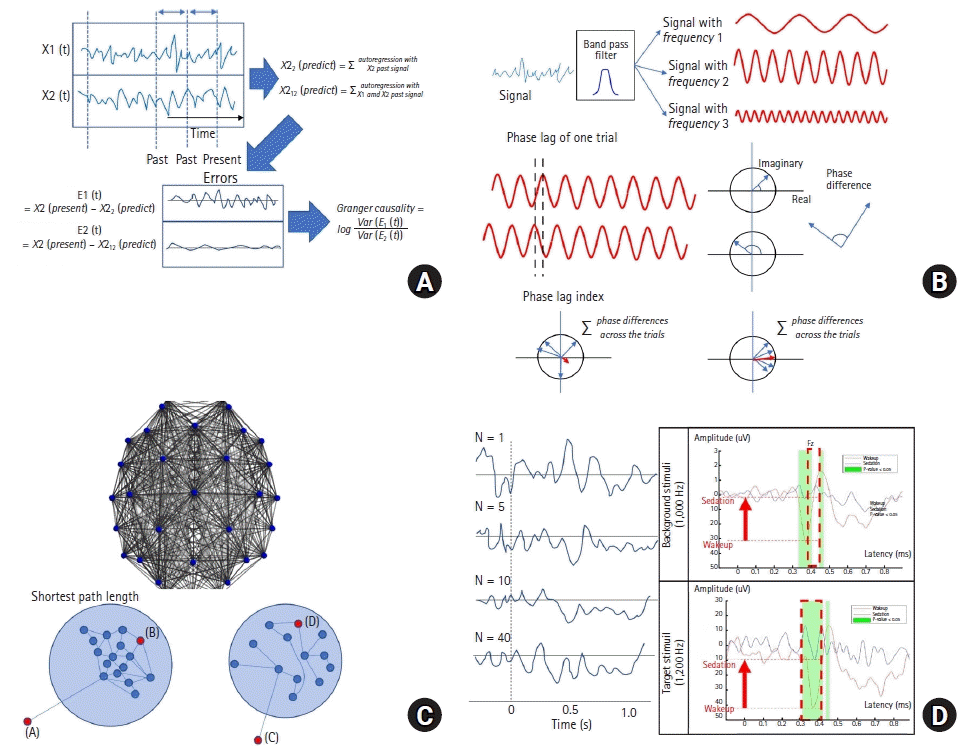