Abstract
Background
The present study was designed to examine the functional recovery following spinal cord injury (SCI) by adjusting the parameters of impact force and dwell-time using the Infinite Horizon (IH) impactor device.
Methods
Sprague-Dawley rats (225–240 g) were divided into eight injury groups based on force of injury (Kdyn) and dwell time (seconds), indicated as Force-Dwell time: 150-4, 150-3, 150-2, 150-1, 150-0, 200-0, 90-2 and sham controls, respectively.
Results
After T10 SCI, higher injury force produced greater spinal cord displacement (P < 0.05) and showed a significant correlation (r = 0.813) between the displacement and the force (P < 0.05). In neuropathic pain-like behavior, the percent of paw withdrawals scores in the hindpaw for the 150-4, 150-3, 150-2, 150-1 and the 200-0 injury groups were significantly lowered compared with sham controls (P < 0.05). The recovery of locomotion had a significant within-subjects effect of time (P < 0.05) and the 150-0 group had increased recovery compared to other groups (P < 0.05). In addition, the 200-0 and the 90-2 recovered significantly better than all the 150 kdyn impact groups that included a dwell-time (P < 0.05). In recovery of spontaneous bladder function, the 150-4 injury group took significantly longer recovery time whereas the 150-0 and the 90-2 groups had the shortest recovery times.
A necessary key in the study of central neuropathic pain (CNP) mechanisms after spinal cord injury (SCI) is the development of a reproducible and clinically relevant rodent animal model for laboratory use: 1) with similar pathophysiology and outcomes observed in people with SCI, and 2) with clear and measurable behavioral outcomes. While there are various rodent animal models of SCI that develop central neuropathic pain [1234567891011], one of the commonly used animal models is the spinal contusion injury model, which parallels the injury profile described in human SCI [481213].
The standardization of contusion parameters yields consistent and reproducible spinal injuries with central neuropathic pain. For example, the New York University (NYU) device allows researchers to develop criteria for inclusion or exclusion based on correlations of behavioral outcomes to specific mechanical parameters of the device, such as measured drop height, impact velocity and cord displacement. Using the NYU impactor, literature reported greater reproducibility and better modeling of spinal injuries with neuropathic pain-like behavioral outcomes [451415161718]. More recently, the "next generation" impact device allows more user input to "dial in" to a desired impact profile, so that the force of impact, spinal cord displacement and duration of compression (dwell-time) is consistently controlled by the programmed device. The OSU device (developed at Ohio State University) [1920] and the Infinite Horizon (IH) impactor (Precision Systems & Instrumentation) [21] are two such devices.
This study was designed to examine chronic neuropathic pain-like behavior, such as mechanical allodynia, in a rat model of SCI by adjusting the parameters of impact force and dwell-time using the IH impactor. In addition, other standards of outcome measures, locomotor function [1522] and spontaneous bladder recovery [23] were analyzed. Based on previous studies [21], we specifically focused our comparison with impact forces of 90, 150 and 200 kdyn combined with dwell-time that had not been previously reported using this device. We experimented with dwell-times ranging from 1 second to 4 seconds in the generation of chronic mechanical allodynia and other standard behavioral measures of locomotor function and bladder function recovery.
Subjects were male Sprague Dawley rats (225-240 g) (Harlan Sprague-Dawley, Houston). Animals were housed on a 12 hour light/dark cycle. Experimental procedures were in accordance with the NIH Guide for the Care and Use of Laboratory Animals as well as the UTMB Institutional Animal Care and Use Committee (IACUC) guidelines. Animals were divided into eight groups with varying injury parameters based on force of injury and dwell time which is the duration of impact (indicated as Force-Dwell time): 150-4 (150 kdyn force impact-4 second dwell time), 150-4, 150-3, 150-2, 150-1, 150-0, 200-0, 90-2 and sham injury (laminectomy only and mounting at the IH frame) controls. In the present study, we have categorized the experimental groups with two points. Firstly, mild to severe injury (between subjects) ranged from 90 kdyn to 200 kdyn injury force are compared. Secondly, 150 kdyn groups (within subject) with dwell times are compared. Previously, we and others commonly have used the 150 kdyn injury force for the spinal cord injury with the IH Impactor device. We have extended the SCI study with more optional parameters; from no dwell time to a 4 second dwell time.
Surgery was performed after intraperitoneal administration of sodium pentobarbital (i.p., 50 mg/kg). Anesthesia was considered sufficient when the flexor-withdrawal reflex to noxious stimulus was absent. After shaving and sterilizing the skin, a #10 blade scalpel was used to make a longitudinal incision along the surface of the back skin. The musculature from the posterior surface of the vertebral bodies on both sides of the vertebral column between thoracic segments 9 and 11 (T9-T11) was cleared and retracted, and a laminectomy was performed exposing the T10 spinal cord segment. Once the spinal cord was exposed, the animal was placed in the IH impactor (Precision Systems and Instruments, LLC, Lexington, KY, USA) by clamping the transverse processes of vertebrae T9 and T11. The IH impactor, uses a 2 mm impact rod tip to contuse the spinal cord and the user determines the force and duration of the impact, the latter being the length of time which the rod compresses the spinal cord at the desired force, or "dwell-time", set in increments of 1 second. The software (PSI IH spinal cord impactor, version 5.0.3) records the actual force of impact (m/sec) and spinal cord displacement (µm). Contusion of the spinal cord was carried out according to the injury parameters for each group. Eight groups of animals were contused: Five groups at 150 kilodynes (kdyn) of force with dwell-times from 0 to 4 seconds (n = 9, n = 9, n = 8, n = 7 and n = 7 respectively); one group was injured at 200 kdyn of force with zero dwell-time (n = 7); and one group was injured at 90 kdyn with a two second dwell-time (n = 8). After impact, the animals were removed from the device, the muscle and fascia were sutured with 5-0 Prolene suture (Ethicon, Somerville, NJ) and the skin incision was closed with autoclips. Animals were allowed to recover on a thermal blanket (Vetko Thermal Barrier, Harvard Apparatus, South Natick, MA) at 37℃. Post-surgical care included food and water ad libitum. Animals had their bladders manually expressed twice daily until two consecutive days of spontaneous bladder control had been demonstrated (minimal to no retained urine at expression time), and were treated with subcutaneous injection of prophylactic antibiotic (Baytril, 30 mg/kg in 0.3 ml injections) during several days following SCI.
Locomotor recovery was tested using the open field test modified by Basso, Beattie and Bresnahan and most commonly known as the BBB Locomotor Rating Scale [22]. The score assesses the locomotor ability following SCI in rodent models and allows validation of the ability of the hindlimbs to locomote and bear weight so that somatosensory testing is feasible. Briefly, Scores 0-7 rank the early phase of recovery indicating movement of the primary 3 joints (hip, knee and ankle), scores 8-13 describe the intermediated phases of recovery from weight bearing stance to coordinated stepping and scores 14-21 rank the late phase with the return of toe clearance, paw position and trunk stability. A score is determined for each hindlimb (left and right) and the combined score is recorded as the daily score for the animal. The BBB scores were measured daily for 14 days and on days post-injury (DPI) 21, 28 and 35.
A blinded observer performed behavioral tests to determine the paw withdrawal force of von Frey filament stimuli. Prior to testing, all animals were environmentally acclimated to the clear Plexiglas testing apparatus (8 × 8 × 18 cm) for 1 hour daily for 3 days. Preoperative testing, consisting of 3 separate days of testing, began 5 days prior to injury to establish presurgical baseline behaviors. Tests were performed postoperatively at day 28 and day 35 after SCI. Since no significant side-to-side differences were found, data from each paw (left and right of both forepaws and hindpaws) were combined for the comparison.
Neuropathic pain-like behaviors induced by SCI were quantified using a paw withdrawal paradigm in both forepaws and hindpaws. Von Frey filaments of a known and calibrated bending force were pressed against the center of the glabrous surface of each paw, and then held for 4 seconds. Each von Frey filament was stepped up until paw withdrawal occurred, accompanied by active attention of the rat to the stimulus by head turning, biting attacks on the stimulus, and whole-body postural changes in response to the mechanical stimuli [7242526]. The calibrated von Frey filament force ranged from undetectable (0.072 mm filament diameter, 0.2 g force) to noxious (1.43 mm filament diameter, 300 g force). In the present study, we have focused the von Frey filament force at which withdrawal firstly occurred and the von Frey filament force was recorded as the withdrawal force value that was displayed by the changes of von Frey filament force. Those withdrawal tests mimic the algometer methods that record the withdrawal force induced by mechanical pressure. To perform these tests, rats were placed inside Plexiglas boxes on an elevated, fine wire mesh screen and acclimated for 60 min prior to testing. The von Frey filament was applied through the mesh to the center of plantar surface of the glabrous skin of each paw. A single trial consisted of the application of the stimuli, applied once every 3 to 4 sec, increasing in intensity. Data were analyzed as a percentage of presurgical baseline values and comparisons were made between the different paw withdrawals. In this method of comparison, post injury scores of 100% indicate no change from presurgical baseline values while scores descending below 100% indicate increased withdrawals to von Frey filaments.
Following SCI, animals showed loss of spontaneous bladder function depending on the severity of the injury. The SCI literature reports that micturition in rats is resumed after a period of several days up to 2 weeks [2327]. We manually expressed the bladder twice daily until the function returned to normal. We used an arbitrary score of "+" (plus), "+/−" (plus/minus) and "−" (minus) for urine retention with plus being very retentive, approximately 1.5 cm in diameter, and minus being normal, 0.5 cm diameter or less. As the animal regains function, there may be times when a minus score is followed by a plus score. Our experience showed that consistent normal function occurred only after three consecutive minus scores. Therefore, an injured animal was considered to have regained normal bladder function on the day of the third consecutive minus score.
Measurements of locomotor recovery were analyzed with a repeated measures analysis of variance. All other tests used a multiple group one way analysis of variance (ANOVA). Where appropriate, groups were compared with Tukey HSD post-hoc statistical tests. Scatter plot with linear regression was used to test the relationship between individual actual force against the actual displacement for each animal. All tests were performed using SPSS software (ver. 14, SPSS Inc., Chicago, IL). Significance was set at P < 0.05. Values are expressed and graphed as mean with standard error of the mean (mean ± S.E.).
Fig. 1 represents the mean actual force (A) and displacement (B) values obtained from the output report of each impact paradigm using the IH impactor. There was no significant difference in force (Fig. 1A, P > 0.6 for all comparisons) or displacement (Fig. 1B, P > 0.8 for all comparisons) between groups with the same injury force parameters (the 150 kdyn groups), independent of any predetermined dwell-time values. However, the actual displacements and forces between groups of differing preset forces were significantly different, independent of dwell-time settings. The 200-0 group (200 kdyn, 0 s dwell time) had a significantly higher actual force (219.5 ± 4.02 kdyn) than any of the 150 kdyn groups (150-0: 165.8 ± 3.84 kdyn; 150-1: 159.7 ± 1.17 kdyn; 150-2: 156.5 ± 2.09 kdyn; 150-3: 161.0 ± 3.04 kdyn; and 150-4: 157.9 ± 2.26 kdyn; *P < 0.05 for all comparisons) and the 90-2 group (93.9 ± 1.01 kdyn; P < 0.05). Each of the 150 kdyn groups had significantly higher actual forces when compared with the 90-2 group as well (#P < 0.05 for all comparisons). Additionally, the 200-0 injury group had a significantly greater spinal cord displacement (1190.2 ± 34.37 µm) than any of the 150 kdyn groups (150-0: 902.2 ± 65.31 µm; 150-1: 926.0 ± 54.42 µm; 150-2: 963.00 ± 78.48 µm; 150-3: 1002.0 ± 30.06 µm; and 150-4: 992.4 ± 27.11 µm; *P < 0.05 for all comparisons) and the 90-2 injury group (534.0 ± 62.95 µm; #P < 0.05). In addition, each of the 150 kdyn injury groups had significantly greater spinal cord displacements than the 90-2 group (P < 0.05 for all comparisons). There was a significant correlation observed, r = 0.813 (Fig. 1C, P < 0.05), for actual spinal cord displacement as a function of actual force of impact. These data indicate the consistency of the impact device, as well as the ability to have a user defined parameter of dwell-time that does not affect other biomechanical properties of the injury, specifically spinal cord displacement or actual force applied in the impact.
Fig. 2A demonstrates the spontaneous recovery of locomotion (BBB scores) among SCI groups. Analysis of variance for repeated measures indicated that 150 kdyn groups (open circle)showed significant differences compared to other groups (150 vs. 200 and 90 kdyn groups) indicating that the locomotor recovery most likely related to the injury parameters assigned to given groups (*P < 0.05 for all comparisons). In addition, the 200-0 (closed diamond) and the 90-2 (open diamond) groups showed more significant recoveries than all the 150-3 (closed square) and 150-4 (open square) groups that included a dwell-time (#P < 0.05 for all comparisons). Interestingly, there was no significant difference between the 200-0 group and the 90-2 group (P > 1.0) indicating a similar locomotor recovery pattern between groups, in spite of one group having an injury force of about one half the intensity but including a 2 second dwell-time. Additionally, there were no significant differences between all 150 kdyn groups that included a dwell-time (P > 0.7 for all comparisons), indicating a similar pattern of locomotor recovery from injuries of the same force that include a dwell-time, irrespective of the duration of dwell-time (within our tested range of 1-4 seconds). To confirm the feasibility of behavior testing, only day 28 post-injury of the BBB scores was analyzed by post hoc tests (28 days post-injury, Fig. 2B). The BBB score of the 150-0 group was 14.63 ± 0.984 and showed significant differences compared to all other groups (*P < 0.05 for all comparisons). The 200-0 and 90-2 groups were 10.36 ± 1.052 and 10.56 ± 0.984, respectively and showed significant differences compared to all 150 kdyn groups (#P < 0.05 for all comparisons). The 150 kdyn with dwell-time groups (150-4: 5.75 ± 1.137; 150-3: 6.14 ± 1.052; 150-2: 6.63 ± 0.984 and 150-1: 6.22 ± 0.982) did not show significant difference between these groups of equal force and varying dwell-times (P > 0.9).
Fig. 3 represents changes of mechanical withdrawal threshold in both forepaws (A) and hindpaws (B) after injury compared to baseline measures. In week 4, percent of baseline threshold scores in forepaws for the 150-4, 150-3 150-2 and 150-1 groups was 20.10 ± 4.7%, 36.07 ± 4.9%, 28.54 ± 5.0% and 30.13 ± 4.1%, respectively and showed significant differences compared to the sham controls (97.12 ± 12.1%, *P < 0.05 for all comparisons). The 150-0 group (69.5 ± 10.9%) and the 200-0 (97.40 ± 7.3%) group showed no significant difference compared with the sham controls; whereas the 90-2 injury group (55.00 ± 10.6%) showed significant difference compared to the sham controls (#P < 0.05, Fig. 3A). By week 5, however, all SCI groups showed significant differences compared to the sham controls ($P < 0.05 for all comparisons, Fig. 3A). In week 4, the percent of baseline threshold scores in hindpaws for the 150-4, 150-3, 150-2 and 150-1 groups was 9.49 ± 3.4%, 9.77 ± 2.1%, 12.36 ± 2.3% and 21.87 ± 3.4%, respectively and showed significant differences compared to the sham controls (101.25 ± 3.5%, *P < 0.05). The 150-0 group (98.69 ± 7.9%) and the 90-2 (93.26 ± 5.6%) group showed no significant difference; whereas the 200-0 injury group (69.33 ± 13.5%) showed significant difference compared to the sham controls (#P < 0.05). In addition, the changes of baseline threshold scores by week 5 showed similar patterns compared to week 4 ($P < 0.05 for all comparisons, Fig. 3B).
Bladder recovery was recorded as the day post-injury on which the third consecutive minus score was recorded (see methods), which is measured at the time of manual bladder expression. In one way ANOVA of the injury groups and post hoc tests for multiple groups showed a statistically significant difference between the groups (Fig. 4). The 150-4 injury group took significantly longer to recover spontaneous bladder function (12.86 ± 0.595 days) than all other groups (*P < 0.05 for all comparisons). The 150-0 (4.88 ± 0.350 days) and the 90-2 (4.75 ± 0.491 days) groups had the fastest recovery times compared to all of the 150 kdyn with dwell-time groups (#P < 0.05 for all comparisons), but the difference compared to the 200-0 group was insignificant (P > 0.7). Additionally, the 200-0 injury group (6.29 ± 0.747 days) recovered more quickly than the 150-1 (9.00 ± 1.143 days) and 150-2 (8.88 ± 0.789 days) groups ($P < 0.05 for each comparison) but not the 150-3 (7.75 ± 0.559 days) group (P > 0.1, Fig. 4).
The design of this study was to examine functional outcomes, such as mechanical allodynia, locomotor recovery and spontaneous bladder recovery, in a rat model of contusive spinal cord injury (SCI) using the IH impactor device. In the present study, we compared functional outcomes by the differential application of injury forces and dwell times. The 150-4 group (150 kdyn - 4 second dwell-time) had the greatest decrease in paw withdrawal forces to mechanical stimuli, which we interpreted to be mechanical allodynia, the longest bladder recovery time and the lowest locomotor recovery score by the end of testing periods. The 150-0 group had the highest BBB scores on all testing days, one of the shortest bladder recovery times, little change in mechanical thresholds in hindpaws. By contrast, the addition of dwell time to the 150 kdyn force resulted in significant mechanical allodynia in forepaws and hindpaws, longer bladder recovery times and lower BBB scores. In addition, the 200-0 group had mechanical allodynia, whereas the 90-2 group, although similar in BBB scores to the 200-0 group, did not display mechanical allodynia to mechanical stimuli.
The differential behavioral outcomes between injury groups could be differently attributed by various mechanical properties of the contusion injury, such as injury force, impact velocity, depth of spinal compression at injury, or duration of compression, respectively. We examined three forces of programmed impact force: 90 (mild), 150 (moderate) and 200 (severe) kdyn and observed significant differences in actual force of injury between rats groups. Additionally, the three groups yielded spinal cord displacements that were significantly different between the groups but not within a group; as impact force increases, the spinal cord displacement also increases. The significant correlations between injury force and tissue displacement using the IH impactor device is also demonstrated in mice SCI [28]. Therefore, our findings suggest that the IH impactor allows for strict and reproducible control over the biomechanical properties of spinal injury to accurately predicted behavioral outcomes.
Of significant note, the addition of any dwell time to the 150 kdyn injury force had no significant effect on either actual force or actual displacement, but had a profound effect on behavioral outcomes. The addition of a dwell time to the 150 kdyn injury significantly reduced the paw withdrawal force for both hindpaws and forepaws; however there were no significant differences between groups for different dwell times. Additionally, an injury with relatively mild impact force (90 kdyn) with two second dwell-time had outcome measures that closely resembled a moderate to severe injury (200 kdyn) with no dwell time. One possible interpretation for this finding is that the increase in compression duration is sufficient to induce an additional ischemic component to the injury. Ischemic injury to the spinal cord induces significant necrosis and apoptosis of neuronal populations [29] and results in increased extracellular concentrations of excitatory amino acids that reach cytotoxic levels and neuropathic pain-like outcome [1030]. Edema, secondary neuronal loss from necrosis, apoptosis and inflammatory cell infiltration result from ischemic events. Ischemia of the spinal cord is the basis of non-contusive models of SCI [13132] where blood flow to the spinal cord is reduced by blocking aortic blood flow or placing a mechanical clamp on the spinal cord for seconds to minutes of durations. The IH impactor with no dwell time setting impacts the cord and quickly retracts from the spinal cord once the programmed force is reached, unlike the NYU device in which the impactor remains for several seconds until the investigator manually withdraws it. We propose that the setting of a dwell time reduces blood flow to surrounding tissue adding ischemic insult to neuronal populations already under stress condition due to SCI. Thus, dwell time exacerbates neural injury that results in worsened outcomes, including mechanical allodynia and delayed recovery of locomotion [28].
Besides mechanical allodynia, other behavioral outcome measures, such as locomotor and bladder function, are important factors to consider in SCI models. Locomotor function allows investigators to evaluate the accuracy and severity of injury, as hindlimb patterns of function are consistent within a given injury profile. One of the common locomotion outcome measures is the open field test with rank scores assigned by trained behaviorists, often referred to as the BBB locomotor score [22]. BBB scores are used to categorize animals into "mild", "moderate" and "severe" categories [415202122]. We confirmed that the injury severity showed significant correlation with the locomotor dysfunction and extended the previous report that the addition of the "dwell time" component worsens the BBB score. Because the force and tissue displacement cause more closed effect on the death of motor neurons, the higher force/displacement causes the lower recovery of locomotion that shows strong correlation to the neuronal damages include the death of motor neurons, myelin sparing, and activation of microglia/macrophages [28].
Another outcome measure that is an important factor to consider in SCI models is lower urinary tract dysfunction after SCI. Bladder dysfunction contributes to morbidity and mortality, and is an important quality of life issue for people with SCI [33]. Literature has shown a strong correlation between severity of trauma and bladder dysfunction after SCI [2327]. We selected measuring the bladder size to test the ability to retain urine. The recovery of bladder function does not show correlation with other behavioral outcomes that were previously reported [3435] suggesting different mechanisms between somatosensory and autonomic pathophysiology following SCI. The emptying/retention of urine is mediated by the activity of the detrusor muscle and external urethral sphincter, which are innervated by sympathetic and parasympathetic tone, respectively [36]. However, SCI interrupts ascending and descending pathways for urination and causes bladder dysfunction that result in increases of urine retention, such as polyuria [34], an important factor of clinical urinary diseases including nephritis and urinary tract infection. It is well documented that the bladder dysfunction following SCI is mediated via the imbalance between descending sympathetic and parasympathetic tones. In the present study, we confirm and extend previous findings, and report that increases of force and displacement significantly affect locomotor abnormality and the duration of bladder dysfunction [343537]. As with mechanical allodynia, the inclusion of a dwell-time in the injury paradigm had a significant negative impact on these outcome measures.
In summary, it is important to characterize the relationship of SCI parameters and behavioral outcomes to suggest putative therapies in animal models on neuropathic pain, recovery of locomotion, and bladder function following SCI [38]. Among the most problematic to people with SCI are bladder, bowel and sexual dysfunction and chronic pain syndromes [33], and the management of those dysfunctions are strongly correlated with perceived quality of life [39]. Because of the consistency and reproducibility of CNP outcomes in the optimized SCI model, the detail studies with molecular, biochemical, anatomical and functional consequences may allow targeted therapeutic interventions. In conclusion, the present study suggest that different parameters of low thoracic spinal contusion injury induced by an IH Imapctor device determine the development and maintenance of mechanical allodynia in both hindpaws, recovery of locomotion on hindlimbs and bladder function, respectively. Therefore, the selection of a SCI model is a critical factor to design the therapeutic strategies for SCI-induced pathophysiological study with neuropathic pain, motor and bladder functions.
ACKNOWLEDGEMENTS
This work was supported by grants from NIH grants NS 11255 and 39161 from United States of America (for Dr. Claire E Hulsebosch) and by the National Research Foundation of Korea (NRF) Grant funded by the Korean Government (MSIP) (NRF-2015R1A5A7037508) and the NRF-2014R1A1A4A01004179.
References
1. Bruce JC, Oatway MA, Weaver LC. Chronic pain after clip-compression injury of the rat spinal cord. Exp Neurol. 2002; 178:33–48. PMID: 12460606.


2. Mills CD, Grady JJ, Hulsebosch CE. Changes in exploratory behavior as a measure of chronic central pain following spinal cord injury. J Neurotrauma. 2001; 18:1091–1105. PMID: 11686495.


3. Vierck CJ Jr, Light AR. Allodynia and hyperalgesia within dermatomes caudal to a spinal cord injury in primates and rodents. Prog Brain Res. 2000; 129:411–428. PMID: 11098708.


4. Lindsey AE, LoVerso RL, Tovar CA, Hill CE, Beattie MS, Bresnahan JC. An analysis of changes in sensory thresholds to mild tactile and cold stimuli after experimental spinal cord injury in the rat. Neurorehabil Neural Repair. 2000; 14:287–300. PMID: 11402879.


5. Hulsebosch CE, Xu GY, Perez-Polo JR, Westlund KN, Taylor CP, McAdoo DJ. Rodent model of chronic central pain after spinal cord contusion injury and effects of gabapentin. J Neurotrauma. 2000; 17:1205–1217. PMID: 11186233.


6. Yezierski RP, Liu S, Ruenes GL, Kajander KJ, Brewer KL. Excitotoxic spinal cord injury: behavioral and morphological characteristics of a central pain model. Pain. 1998; 75:141–155. PMID: 9539683.


7. Christensen MD, Everhart AW, Pickelman JT, Hulsebosch CE. Mechanical and thermal allodynia in chronic central pain following spinal cord injury. Pain. 1996; 68:97–107. PMID: 9252004.


8. Siddall P, Xu CL, Cousins M. Allodynia following traumatic spinal cord injury in the rat. Neuroreport. 1995; 6:1241–1244. PMID: 7669978.


9. Ovelmen-Levitt J, Gorecki J, Nguyen KT, Iskandar B, Nashold BS Jr. Spontaneous and evoked dysesthesias observed in the rat after spinal cordotomies. Stereotact Funct Neurosurg. 1995; 65:157–160. PMID: 8916347.


10. Xu XJ, Hao JX, Aldskogius H, Seiger A, Wiesenfeld-Hallin Z. Chronic pain-related syndrome in rats after ischemic spinal cord lesion: a possible animal model for pain in patients with spinal cord injury. Pain. 1992; 48:279–290. PMID: 1589248.


11. Hao JX, Xu XJ, Aldskogius H, Seiger A, Wiesenfeld-Hallin Z. Allodynia-like effects in rat after ischaemic spinal cord injury photochemically induced by laser irradiation. Pain. 1991; 45:175–185. PMID: 1652116.


12. Bunge RP, Puckett WR, Becerra JL, Marcillo A, Quencer RM. Observations on the pathology of human spinal cord injury. A review and classification of 22 new cases with details from a case of chronic cord compression with extensive focal demyelination. Adv Neurol. 1993; 59:75–89. PMID: 8420126.
13. Kakulas BA, Taylor JR. Pathology of injuries of the vertebral column and spinal cord. In : Vinken PJ, Bruyn GW, Klawans HL, Frankel HL, editors. Handbook of clinical neurology. Vol 17. Amsterdam: Elsevier;1992. p. 21–51.
14. Metz GA, Curt A, van de Meent H, Klusman I, Schwab ME, Dietz V. Validation of the weight-drop contusion model in rats: a comparative study of human spinal cord injury. J Neurotrauma. 2000; 17:1–17. PMID: 10674754.


15. Basso DM, Beattie MS, Bresnahan JC. Graded histological and locomotor outcomes after spinal cord contusion using the NYU weight-drop device versus transection. Exp Neurol. 1996; 139:244–256. PMID: 8654527.


16. Constantini S, Young W. The effects of methylprednisolone and the ganglioside GM1 on acute spinal cord injury in rats. J Neurosurg. 1994; 80:97–111. PMID: 8271028.


17. Gruner JA. A monitored contusion model of spinal cord injury in the rat. J Neurotrauma. 1992; 9:123–126. PMID: 1404425.


18. Wrathall JR, Pettegrew RK, Harvey F. Spinal cord contusion in the rat: production of graded, reproducible, injury groups. Exp Neurol. 1985; 88:108–122. PMID: 3979505.


19. Stokes BT, Noyes DH, Behrmann DL. An electromechanical spinal injury technique with dynamic sensitivity. J Neurotrauma. 1992; 9:187–195. PMID: 1474607.


20. Stokes BT. Experimental spinal cord injury: a dynamic and verifiable injury device. J Neurotrauma. 1992; 9:129–131. PMID: 1404426.


21. Scheff SW, Rabchevsky AG, Fugaccia I, Main JA, Lumpp JE Jr. Experimental modeling of spinal cord injury: characterization of a force-defined injury device. J Neurotrauma. 2003; 20:179–193. PMID: 12675971.


22. Basso DM, Beattie MS, Bresnahan JC. A sensitive and reliable locomotor rating scale for open field testing in rats. J Neurotrauma. 1995; 12:1–21. PMID: 7783230.


23. Pikov V, Wrathall JR. Coordination of the bladder detrusor and the external urethral sphincter in a rat model of spinal cord injury: effect of injury severity. J Neurosci. 2001; 21:559–569. PMID: 11160435.


24. Christensen MD, Hulsebosch CE. Chronic central pain after spinal cord injury. J Neurotrauma. 1997; 14:517–537. PMID: 9300563.


25. Choi Y, Yoon YW, Na HS, Kim SH, Chung JM. Behavioral signs of ongoing pain and cold allodynia in a rat model of neuropathic pain. Pain. 1994; 59:369–376. PMID: 7708411.


26. Kim SH, Chung JM. An experimental model for peripheral neuropathy produced by segmental spinal nerve ligation in the rat. Pain. 1992; 50:355–363. PMID: 1333581.


27. Wrathall JR, Emch GS. Effect of injury severity on lower urinary tract function after experimental spinal cord injury. Prog Brain Res. 2006; 152:117–134. PMID: 16198697.


28. Streijger F, Beernink TM, Lee JH, Bhatnagar T, Park S, Kwon BK, et al. Characterization of a cervical spinal cord hemicontusion injury in mice using the infinite horizon impactor. J Neurotrauma. 2013; 30:869–883. PMID: 23360150.


29. Kato H, Kanellopoulos GK, Matsuo S, Wu YJ, Jacquin MF, Hsu CY, et al. Neuronal apoptosis and necrosis following spinal cord ischemia in the rat. Exp Neurol. 1997; 148:464–474. PMID: 9417826.


30. Rokkas CK, Cronin CS, Nitta T, Helfrich LR Jr, Lobner DC, Choi DW, et al. Profound systemic hypothermia inhibits the release of neurotransmitter amino acids in spinal cord ischemia. J Thorac Cardiovasc Surg. 1995; 110:27–35. PMID: 7609553.


31. Taira Y, Marsala M. Effect of proximal arterial perfusion pressure on function, spinal cord blood flow, and histopathologic changes after increasing intervals of aortic occlusion in the rat. Stroke. 1996; 27:1850–1858. PMID: 8841344.


32. Rivlin AS, Tator CH. Effect of duration of acute spinal cord compression in a new acute cord injury model in the rat. Surg Neurol. 1978; 10:38–43. PMID: 684604.
33. Anderson KD. Targeting recovery: priorities of the spinal cord-injured population. J Neurotrauma. 2004; 21:1371–1383. PMID: 15672628.


34. Ward PJ, Hubscher CH. Persistent polyuria in a rat spinal contusion model. J Neurotrauma. 2012; 29:2490–2498. PMID: 22708983.


35. Sharp K, Yee KM, Steward O. A re-assessment of the effects of treatment with an epidermal growth factor receptor (EGFR) inhibitor on recovery of bladder and locomotor function following thoracic spinal cord injury in rats. Exp Neurol. 2012; 233:649–659. PMID: 22078761.


36. de Groat WC, Yoshimura N. Mechanisms underlying the recovery of lower urinary tract function following spinal cord injury. Prog Brain Res. 2006; 152:59–84. PMID: 16198694.


37. David BT, Steward O. Deficits in bladder function following spinal cord injury vary depending on the level of the injury. Exp Neurol. 2010; 226:128–135. PMID: 20713043.


38. Kennedy P, Frankel H, Gardner B, Nuseibeh I. Factors associated with acute and chronic pain following traumatic spinal cord injuries. Spinal Cord. 1997; 35:814–817. PMID: 9429260.


39. Hicken BL, Putzke JD, Richards JS. Bladder management and quality of life after spinal cord injury. Am J Phys Med Rehabil. 2001; 80:916–922. PMID: 11821674.


Fig. 1
The relationship between actual force of impact and actual spinal cord displacement. All programmed injury mode produced consistent actual injury outcomes. In comparison of injury force (A) and displacement (B), the 200 kdyn group (*P < 0.05) and 90 kydn group (#P < 0.05) showed significant difference compared to 150 kdyn group, respectively. However, the all 150 kdyn groups did not show significant differences, suggesting the programmed injury mode produced consistent outcomes. (C) Scatter plot with linear regression graph revealed significant correlation between the actual force and the tissue displacement. Data are plotted as mean ± S.E.

Fig. 2
BBB rank scores of hindlimb locomotor function after SCI with varying impact forces and dwell-times. (A) The 150 kdyn with no dwell-time (150-0) group (*P < 0.05) showed the most improvement over time. Five days after SCI, 200-0 and 90-2 groups showed significant differences compared to 150-3 group (#P < 0.05). (B) The 150-0 group resulted in a significantly higher BBB score than all other injury groups at this time point (*P < 0.05). The both 200-0 and 90-2 groups showed significant differences compared with 150-0 groups (#P < 0.05). Data are plotted as mean ± S.E.

Fig. 3
Histogram of paws withdrawal responsiveness to von Frey filaments. Mechanical allodynia was evident at both forelimbs (A) and hindlimbs (B), respectively. 150 kdyn with dwell time groups showed mechanical allodynia at both 4 and 5 wks after SCI whereas other groups showed variable patterns. (*P < 0.05 for 4 weeks and $P < 0.05 for 5 weeks compared to sham group, respectively). Data are plotted as mean ± S.E.
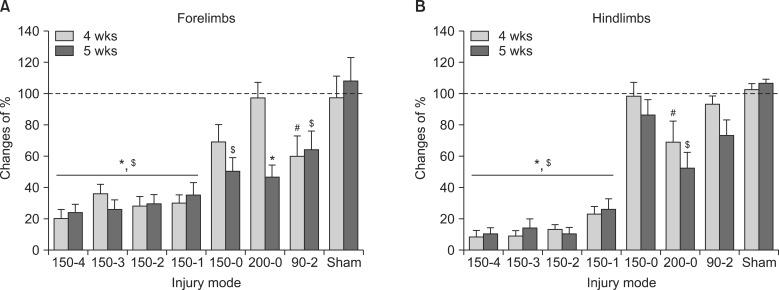
Fig. 4
The duration of bladder dysfunction until onset of spontaneous bladder voiding. Note that the 150-4 group took significantly longer to recover function than all other groups (*P < 0.05). The 150-0 and 90-2 groups showed significant differences compared with 150 kdyn with dwell time groups (#P < 0.05). Additionally, the 200-0 injury group recovered more quickly than the 150-1 and 150-2 groups ($P < 0.05). Data are shown as mean days post-injury for full bladder voiding ± S.E.
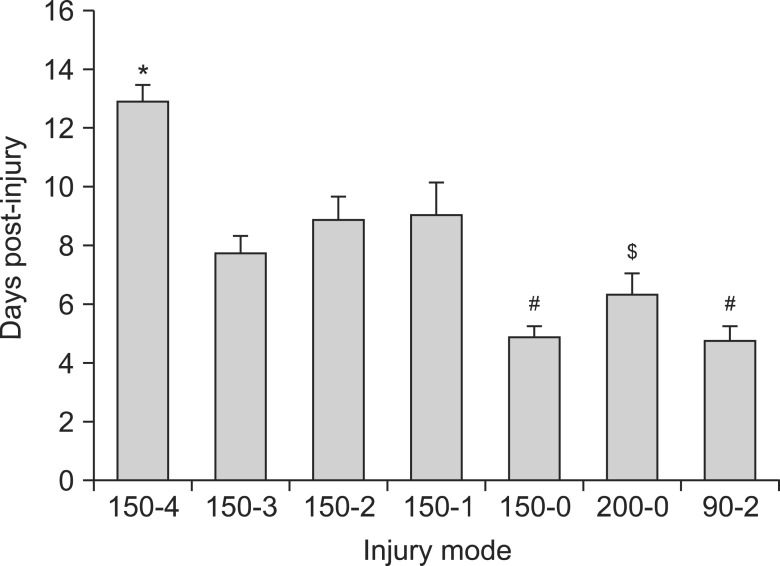