Abstract
Background
Nefopam is a centrally acting non-opioid analgesic agent. Its analgesic properties may be related to the inhibitions of monoamine reuptake and the N-methyl-D-aspartate (NMDA) receptor. The antinociceptive effect of nefopam has been shown in animal models of acute and chronic pain and in humans. However, the effect of nefopam on diabetic neuropathic pain is unclear. Therefore, we investigated the preventive effect of nefopam on diabetic neuropathic pain induced by streptozotocin (STZ) in rats.
Methods
Pretreatment with nefopam (30 mg/kg) was performed intraperitoneally 30 min prior to an intraperitoneal injection of STZ (60 mg/kg). Mechanical and cold allodynia were tested before, and 1 to 4 weeks after drug administration. Thermal hyperalgesia was also investigated. In addition, the transient receptor potential ankyrin 1 (TRPA1) and TRP melastatin 8 (TRPM8) expression levels in the dorsal root ganglion (DRG) were evaluated.
Nefopam is a centrally acting non-opioid analgesic drug of the benzoxazocine chemical class [1]. It has been reported that its analgesic mechanisms may be related to the inhibition of monoamine reuptake [2] and the N-methyl-D-aspartate (NMDA) receptor [3]. The antinociceptive effect of nefopam has been shown in various animal models of acute and chronic pain. For example, its antinociceptive properties have been revealed using noxious heat stimuli (hot-plate) either in intact or in injured rats after skin and muscular incisions [4]. Nefopam can also inhibit nociceptive behaviors in acetic acid-induced writhing and formalin tests in the mouse [2]. Moreover, nefopam has shown a protective analgesic effect on allodynia induced by the chronic constriction of the sciatic nerve [5,6]. However, the effect of nefopam on diabetic neuropathic pain remains unclear.
It is well established that diabetic neuropathic pain can easily induced by a systemic administration of streptozotocin (STZ). Systemically administered STZ has cytotoxic effect to the pancreatic β-cell [7]. Although STZ-induced diabetic rodents are hypoinsulinemic, they generally do not require an exogenous insulin treatment to survive. Diabetic neuropathic pain occurs mainly due to damage in the nervous system [8].
Since the characterization of the first nociceptive ion channel transient receptor potential (TRP) vanilloid 1 (TRPV1) [9], many other TRP channels on nociceptive neurons have been characterized and targeted for pain relief [10]. Among them, it has been observed that the inhibition of the TRP ankyrin 1 (TRPA1) ion channel reduced mechanical hypersensitivity [11] and the loss of the cutaneous nerve fiber function in diabetic animals [12] implying a contribution of the TRPA1 channel to the pathogenesis of diabetic neuropathic pain [13]. In addition, TRP melastatin 8 (TRPM8), which is known to be activated by cool stimuli and menthol, may be involved in the development of cold allodynia in some neuropathic pain models [14,15]. However, the effects of nefopam on the diabetic neuropathic pain and relationships with TRP proteins expression in the DRG are not well known.
Therefore, the present study investigated the preventive effect of nefopam on diabetic neuropathic pain induced by STZ in rats. Furthermore, the levels of TRPM8 and TRPA1 expressions in the dorsal root ganglion (DRG) were examined.
This study was reviewed and approved by the Institutional Animal Care and Use Committee (IACUC) of the Asan Institute for Life Sciences, Asan Medical Center. The committee follows the Institute of Laboratory Animal Resources (ILAR) guide.
Male Sprague-Dawley rats (160-180 g) were housed three per cage in a room maintained at 21 ± 1.0℃ with an alternating 12 h light-dark cycle. The rats were provided with water and food ad libitum and were permitted to acclimate for at least three days prior to their use in experiments. The animals were allowed to adapt to the laboratory for at least 2 h before testing, and they were used only once. To reduce variation, all experiments were performed during the light phase of the cycle (10:00-17:00).
The rats were randomly divided into three groups (seven rats per group): 1) the control group, which received only the vehicle; 2) the STZ group, which received STZ; and 3) the nefopam group, which received nefopam before the administration of STZ. After overnight fasting, diabetes was induced by an intraperitoneal injection of STZ (Sigma-Aldrich Co., St. Louis, MO, USA). The STZ solution was prepared freshly by dissolving normal saline and was injected intraperitoneally at a dose of 60 mg/kg, as previously described [16]. Age-matched control rats received a vehicle alone. The induction of diabetes was assessed by weekly measurement of the tail vein blood glucose level using a commercial blood glucose meter (Optium Xceed®, Abbott Diabetes Care, Alameda, CA, USA). Body weight was also monitored. Diabetic rats with hyperglycemia (blood glucose levels of more than 300 mg/dL from 1 to 4 weeks) were included in the study. Nefopam (Acupan®, Pharmbio Korea Co., Seoul, Korea) was injected intraperitoneally at a dose of 30 mg/kg 30 min before the STZ administration. The dose of nefopam was chosen based on previous publications [4,6]. During the administration of nefopam, the control and STZ groups were treated with the vehicle for nefopam (normal saline).
Behavioral tests were performed before, and 1 to 4 weeks after the final administration of the drugs in order to measure behavioral responses to mechanical and thermal stimuli. To evaluate the expressions of the TRPM8 and TRPA1 proteins in the DRG, the DRG was isolated 4 weeks after the final drug administration.
Behavioral tests were performed during the same time period on each testing day (10:00 AM to 4:00 PM). Rats were positioned in individual plastic cages with wire-mesh bottoms and were permitted to acclimate for 20 minutes. Baseline testing was performed before drug administration to verify that the animal responses were normal. To test for mechanical allodynia, a von Frey filament (Stoelting, Wood Dale, IL, USA) was used to stimulate the plantar surface of the left hind foot. Eight calibrated von Frey filaments (0.41, 0.70, 1.20, 2.00, 3.63, 5.50, 8.50, and 15.10 g) were sequentially applied to the plantar surface of the foot (in an ascending or a descending order), and gently pressed against the foot. Rapid withdrawal or flinching was interpreted as a positive response, in which case the next-lightest filament was tested; a negative response led to the application of the next heaviest filament. Determination of the 50% withdrawal threshold was made using the up-down method, as previously described [17,18,19].
Responses to cold stimuli were examined using the acetone method, as previously reported [19]. Briefly, a drop of acetone was lightly placed onto the plantar surface of the left hind foot. Acetone was applied 5 times to the hind foot at 5 min intervals. A rapid withdrawal in response to the application of acetone was considered as a positive sign. The number of withdrawal responses was counted. Rats that showed a withdrawal response in more than 2 of 5 applications (40%) were classified as demonstrating cold allodynia.
For the hot-plate test, rats were individually placed on a 55℃ hot-plate apparatus (Ugo-Basile, Varese, Italy) as previously reported [5], after which the reaction time starting from the placement of the rat on the hot-plate to the time the rat licked its hind paw was measured. The cut-off time of the hot-plate test was approximately 12 sec.
After dissecting the L4-L6 DRG, corresponding to the afferent nervous pathway from the hind paw, the tissue was washed two times with cold Tris-buffered saline (TBS; 20 mM Trizma base and 137 mM NaCl, pH 7.5). Immediate after washing, cells were lysed with SDS lysis buffer (62.5 mM Trizma base, 2% w/v SDS, 10% glycerol) containing 0.1 mM Na3VO4, 3 mg/mL of aprotinin, and 20 mM NaF. After brief sonication to shear DNA and reduce the viscosity of this mixture, the protein concentration was determined with a detergent-compatible protein assay reagent (Bio-Rad Laboratories, Richmond, CA, USA) using bovine serum albumin as the standard. After the addition of dithiothreitol (5 mM) and bromophenol blue (0.1% w/v) and boiling, the total proteins (40 µg) were separated by electrophoresis in 15% polyacrylamide gels and transferred onto a polyvinylidene difluoride membrane (Amersham Pharmacia Bioscience, Little Chalfont, Buckinghamshire, UK). The membranes were immunoblotted with antibodies against TRPA1 (1:1000; Abcam, Cambridge, MA, USA), TRPM8 (1:1000; Abcam) and β-actin (1:1000; Bethyl Laboratories INC., Montgomery, TX, USA) and visualized with the ECL-plus solution (Amersham Pharmacia Bioscience). The membranes were then exposed to Hyperfilm-MP (Amersham Pharmacia Bioscience) to detect the light emissions. The specific signals for TRPM8, TRPA1 and β-actin were quantified using ImageJ freeware (NIH, USA).
The histological findings in the three rats used in the behavioral tests were also analyzed (n = 3 rats per group) [18,20]. Briefly, under anesthesia, the rats were transcardially perfused with 4% buffered paraformaldehyde four weeks after the administration of STZ. The L5 DRG was collected and subsequently fixed in 4% paraformaldehyde, embedded in paraffin, and sectioned to a thickness of 4 µm. After blocking with 5% goat serum, 0.3% Triton X-100, and 1% bovine serum albumin in phosphate-buffered saline (PBS-T), double-label immunofluorescence was conducted by incubating the paraffin sections overnight at 4℃ with rabbit polyclonal antibodies against TRPM8 (1:1000, Abcam), with the IgG fraction of the mouse polyclonal antibody against the N52 clone of neurofilament 200 (NF200, 1:200; Sigma-Aldrich), followed by incubation with Alexa Flour 555 goat anti-rabbit IgG (red; 1:500; Invitrogen, Carlsbad, CA, USA) and Alexa Flour 488 goat anti-mouse IgG (green; 1:500; Invitrogen) for 2 hours at 20℃. The immunostained DRG sections were observed using a confocal microscope.
The data are presented as the means ± standard error. Behavioral data (withdrawal threshold to mechanical and thermal stimuli) were evaluated by two-way repeated measures analysis of variance (ANOVA). The statistical significance of the differences for the quantified specific signals for TRPM8 and TRPA1 was assessed with a one-way ANOVA followed by the Bonferroni's post hoc test. P values less than 0.05 were considered significant.
The baseline threshold to evoke a withdrawal response to mechanical stimuli in all rats before drug administration was 15.10 g (maximal mechanical stimulus). the paw withdrawal threshold to mechanical stimuli was reduced significantly after the STZ treatment in a time-dependent manner (Fig. 2A). A systemic pretreatment with 30 mg/kg of nefopam inhibited STZ-induced mechanical allodynia significantly during the testing period (up to 4 weeks). STZ produced significant cold allodynia after 1 week, which was sustained until 4 weeks after the STZ administration. The withdrawal response to cold stimuli was significantly increased in the STZ-treated rats. Pretreatment with nefopam inhibited STZ-induced cold allodynia significantly in a time-dependent manner (Fig. 2B). In the hot-plate test, the paw withdrawal latency to noxious thermal stimuli (55℃) was significantly decreased in STZ-treated rats from 2 to 4 weeks after administration. A pretreatment with the same dose of nefopam did not significantly affect STZ-induced noxious thermal hyperalgesia (Fig. 2C).
As shown in Fig. 3A, an STZ injection did not affect the basal level of the TRPA1 protein in the DRG. On the other hand, an immunoblot analysis revealed that the TRPM8 protein level in the DRG significantly increased 4 weeks after STZ injection, which was reduced by the nefopam pretreatment (Fig. 3B). The β-actin expressions in the DRG were not altered in any of the experimental groups. An immunohistochemical analysis 4 weeks after the STZ injection revealed an increase in TRPM8 immunoreactivity (IR) in the DRG cells, which was co-localized with NF200-positive neurons (Fig. 4). Moreover, the nefopam pretreatment decreased the STZ-induced TRPM8-IR, as shown Fig. 4.
In this study, pretreatment with nefopam significantly inhibited STZ-induced mechanical and cold allodynia, but not thermal hyperalgesia. The STZ injection increased the TRPM8, but not the TRPA1, protein expression levels in the DRG. The nefopam pretreatment decreased the STZ-induced TRPM8 expression level in the DRG.
Antiallodynic or antihyperalgesic effects of nefopam pretreatment were previously evaluated in other models of neuropathic pain. For example, a single intraperitoneal pretreatment, but not a post-treatment, of nefopam significantly increased the withdrawal threshold to mechanical stimuli and the paw licking latency to noxious thermal stimuli (51℃) in a rat model of chronic constriction injury of the sciatic nerve [5]. Laboureyras et al. [21] reported that a preemptive treatment of nefopam prevented the development of long-term pain hypersensitivity induced by a surgical incision. Similarly, in the STZ-induced diabetic neuropathic pain model used in the present study, a nefopam pretreatment significantly inhibited STZ-induced mechanical and cold allodynia.
However, in contrast to previous reports which used constriction injuries of the sciatic nerve [5], a nefopam pretreatment did not attenuate thermal hyperalgesia in the present study. This discrepancy may be at least partially explained by the different progression rates of the development of hyperalgesia in diabetic neuropathic pain. In the STZ-induced diabetic rat, this type of neuropathy progression is not very rapid, as vascular dysfunction developed after 1 week and functional abnormalities within 1 month [22]. Given the relative short action duration of nefopam compared to the development of diabetic neuropathy, only a single pretreatment of nefopam before an STZ injection may be less effective at blocking central sensitization than before a sciatic nerve constriction injury [23].
It has been proposed that the TRPA1 channel may be involved in the development as well as the maintenance of diabetic mechanical hypersensitivity [11,12,13] and cold allodynia [24,25]. Wei and colleagues [11] demonstrated that a TRPA1 channel antagonist attenuated mechanical hypersensitivity in diabetic animals. Furthermore, the TRPA1 gene expression level in the DRG 14 days after a STZ injection was enhanced by 45% in diabetic rats [24]. In the present study, the TRPA1 protein level in DRG was not affected 4 weeks after the STZ injection. The characterization of the exact time course of TRPA1 protein expression in diabetic rats remains to be elucidated.
On the other hand, the TRPM8 protein level in DRG neurons was increased after the STZ injection and decreased by the nefopam pretreatment in the present study. To the best of our knowledge, there is no report of a relationship between TRPM8 and cold allodynia induced by STZ. Our results appear to be similar to those with other neuropathic pain models. That is, the level of the TRPM8 protein in the L5 DRG in chronic constriction-injured rats increased significantly [14,15]. TRPM8 is well known as a cold sensation receptor, and several studies have indicated a relationship between TRPM8 expression and cold hyperalgesia and allodynia. For example, a study using TRPM8 and TRPA1 knockout animals revealed that noxious cold signaling may be exclusive to TRPM8, mediating neural and behavioral responses to cold and cold-mimetics [26]. It was shown that nocifensive behaviors to cold stimuli were absent in TRPM8 knockout and TRPM8/TRPA1 double-knockout mice but were retained in TRPA1 null mice. Similarly, cold allodynia induced by the application of icilin or acetone was attenuated in TRPM8 knockout mice [27]. Therefore, given the present results, the preventive effects of nefopam on STZ-induced cold allodynia appear to be related to TRPM8 located in DRG neurons. In contrast, the role of the TRPM8 receptor with regard to sensations of mechanical stimuli is not well understood. There are conflicting results about association between TRPM8 and mechanical hypersensitivity indicating that a complex mechanism between the TRPM8 receptor and mechanical allodynia may exist [28,29,30,31]. Therefore, the preventive effects of nefopam on STZ-induced mechanical allodynia cannot solely be explained by the TRPM8 expression levels in DRG neurons. This remains to be elucidated in a further study.
The present experiment has some limitations. First, diabetic neuropathy does not always manifest in STZ-induced diabetic rats. Kim et al. [32] reported that the incidence of diabetic neuropathy in STZ-induced diabetic rats is 85.7% with regard to both mechanical allodynia and cold allodynia. Because nefopam was given before the STZ injection, we could not determine that the incidence of diabetic neuropathy was equivalent in both groups. Therefore, our result may be influenced by the incidence of neuropathic pain development. In addition, STZ-induced diabetic rats can show certain structural changes of the peripheral nerves, such as axonal atrophy, axonal disjunction, and fiber demyelination, despite the fact that these changes evolve slowly [8]. However, the effects of a nefopam pretreatment on pathologic structural changes during STZ-induced diabetic neuropathy were not evaluated in the current study. This can be explored in future work which exploits the effect of a nefopam pretreatment on the peripheral nerve structure in a diabetic rat model.
In conclusion, a pretreatment with nefopam was shown to inhibit STZ-induced mechanical and cold allodynia significantly, but this was not the case for noxious thermal hyperalgesia. Pretreatment with nefopam also decreased the STZ-induced TRPM8 expression in the DRG neurons.
References
1. Durrieu G, Olivier P, Bagheri H, Montastruc JL. French Network of Pharmacovigilance Centers. Overview of adverse reactions to nefopam: an analysis of the French Pharmacovigilance database. Fundam Clin Pharmacol. 2007; 21:555–558. PMID: 17868209.


2. Girard P, Coppé MC, Verniers D, Pansart Y, Gillardin JM. Role of catecholamines and serotonin receptor subtypes in nefopam-induced antinociception. Pharmacol Res. 2006; 54:195–202. PMID: 16750379.


3. Verleye M, André N, Heulard I, Gillardin JM. Nefopam blocks voltage-sensitive sodium channels and modulates glutamatergic transmission in rodents. Brain Res. 2004; 1013:249–255. PMID: 15193535.


4. Girard P, Pansart Y, Coppe MC, Gillardin JM. Nefopam reduces thermal hypersensitivity in acute and postoperative pain models in the rat. Pharmacol Res. 2001; 44:541–545. PMID: 11735363.


5. Biella GE, Groppetti A, Novelli A, Fernández-Sánchez MT, Manfredi B, Sotgiu ML. Neuronal sensitization and its behavioral correlates in a rat model of neuropathy are prevented by a cyclic analog of orphenadrine. J Neurotrauma. 2003; 20:593–601. PMID: 12906743.


6. Saghaei E, Moini Zanjani T, Sabetkasaei M, Naseri K. Enhancement of antinociception by co-administrations of nefopam, morphine, and nimesulide in a rat model of neuropathic pain. Korean J Pain. 2012; 25:7–15. PMID: 22259710.


7. Schnedl WJ, Ferber S, Johnson JH, Newgard CB. STZ transport and cytotoxicity. Specific enhancement in GLUT2-expressing cells. Diabetes. 1994; 43:1326–1333. PMID: 7926307.


8. Sima AA, Sugimoto K. Experimental diabetic neuropathy: an update. Diabetologia. 1999; 42:773–788. PMID: 10440118.


9. Caterina MJ, Schumacher MA, Tominaga M, Rosen TA, Levine JD, Julius D. The capsaicin receptor: a heat-activated ion channel in the pain pathway. Nature. 1997; 389:816–824. PMID: 9349813.


10. Brederson JD, Kym PR, Szallasi A. Targeting TRP channels for pain relief. Eur J Pharmacol. 2013; 716:61–76. PMID: 23500195.


11. Wei H, Hämäläinen MM, Saarnilehto M, Koivisto A, Pertovaara A. Attenuation of mechanical hypersensitivity by an antagonist of the TRPA1 ion channel in diabetic animals. Anesthesiology. 2009; 111:147–154. PMID: 19512877.


12. Koivisto A, Hukkanen M, Saarnilehto M, Chapman H, Kuokkanen K, Wei H, et al. Inhibiting TRPA1 ion channel reduces loss of cutaneous nerve fiber function in diabetic animals: sustained activation of the TRPA1 channel contributes to the pathogenesis of peripheral diabetic neuropathy. Pharmacol Res. 2012; 65:149–158. PMID: 22133672.


13. Wei H, Chapman H, Saarnilehto M, Kuokkanen K, Koivisto A, Pertovaara A. Roles of cutaneous versus spinal TRPA1 channels in mechanical hypersensitivity in the diabetic or mustard oil-treated non-diabetic rat. Neuropharmacology. 2010; 58:578–584. PMID: 20004676.


14. Su L, Wang C, Yu YH, Ren YY, Xie KL, Wang GL. Role of TRPM8 in dorsal root ganglion in nerve injury-induced chronic pain. BMC Neurosci. 2011; 12:120. PMID: 22111979.


15. Xing H, Chen M, Ling J, Tan W, Gu JG. TRPM8 mechanism of cold allodynia after chronic nerve injury. J Neurosci. 2007; 27:13680–13690. PMID: 18077679.


16. Talbot S, Chahmi E, Dias JP, Couture R. Key role for spinal dorsal horn microglial kinin B1 receptor in early diabetic pain neuropathy. J Neuroinflammation. 2010; 7:36. PMID: 20587056.
17. Chaplan SR, Bach FW, Pogrel JW, Chung JM, Yaksh TL. Quantitative assessment of tactile allodynia in the rat paw. J Neurosci Methods. 1994; 53:55–63. PMID: 7990513.


18. Kim SH, Nam JS, Choi DK, Koh WW, Suh JH, Song JG, et al. Tumor necrosis factor-alpha and apoptosis following spinal nerve ligation injury in rats. Korean J Pain. 2011; 24:185–190. PMID: 22220239.


19. Li Y, Dorsi MJ, Meyer RA, Belzberg AJ. Mechanical hyperalgesia after an L5 spinal nerve lesion in the rat is not dependent on input from injured nerve fibers. Pain. 2000; 85:493–502. PMID: 10781924.


20. Choi SS, Koh WU, Nam JS, Shin JW, Leem JG, Suh JH. Effect of ethyl pyruvate on Paclitaxel-induced neuropathic pain in rats. Korean J Pain. 2013; 26:135–141. PMID: 23614074.


21. Laboureyras E, Chateauraynaud J, Richebé P, Simonnet G. Long-term pain vulnerability after surgery in rats: prevention by nefopam, an analgesic with antihyperalgesic properties. Anesth Analg. 2009; 109:623–631. PMID: 19608840.


22. Sigaudo-Roussel D, Fromy B, Saumet JL. Diabetic neuropathy in animal models. Drug Discov Today Dis Models. 2007; 4:39–44.


23. Leurs R, Donnell D, Timmerman H, Bast A. Cytochrome P450 metabolic intermediate complex of nefopam. J Pharm Pharmacol. 1987; 39:835–837. PMID: 2891822.


24. Barrière DA, Rieusset J, Chanteranne D, Busserolles J, Chauvin MA, Chapuis L, et al. Paclitaxel therapy potentiates cold hyperalgesia in streptozotocin-induced diabetic rats through enhanced mitochondrial reactive oxygen species production and TRPA1 sensitization. Pain. 2012; 153:553–561. PMID: 22177224.


25. Wrigley PJ, Jeong HJ, Vaughan CW. Primary afferents with TRPM8 and TRPA1 profiles target distinct subpopulations of rat superficial dorsal horn neurones. Br J Pharmacol. 2009; 157:371–380. PMID: 19371346.


26. Knowlton WM, Bifolck-Fisher A, Bautista DM, McKemy DD. TRPM8, but not TRPA1, is required for neural and behavioral responses to acute noxious cold temperatures and cold-mimetics in vivo. Pain. 2010; 150:340–350. PMID: 20542379.


27. Colburn RW, Lubin ML, Stone DJ Jr, Wang Y, Lawrence D, D'Andrea MR, et al. Attenuated cold sensitivity in TRPM8 null mice. Neuron. 2007; 54:379–386. PMID: 17481392.


28. Klein AH, Sawyer CM, Carstens MI, Tsagareli MG, Tsiklauri N, Carstens E. Topical application of L-menthol induces heat analgesia, mechanical allodynia, and a biphasic effect on cold sensitivity in rats. Behav Brain Res. 2010; 212:179–186. PMID: 20398704.


29. Namer B, Seifert F, Handwerker HO, Maihöfner C. TRPA1 and TRPM8 activation in humans: effects of cinnamaldehyde and menthol. Neuroreport. 2005; 16:955–959. PMID: 15931068.


30. Calcutt NA. Potential mechanisms of neuropathic pain in diabetes. Int Rev Neurobiol. 2002; 50:205–228. PMID: 12198811.


31. Proudfoot CJ, Garry EM, Cottrell DF, Rosie R, Anderson H, Robertson DC, et al. Analgesia mediated by the TRPM8 cold receptor in chronic neuropathic pain. Curr Biol. 2006; 16:1591–1605. PMID: 16920620.


32. Kim SH, Kwon JK, Kwon YB. Pain modality and spinal glia expression by streptozotocin induced diabetic peripheral neuropathy in rats. Lab Anim Res. 2012; 28:131–136. PMID: 22787487.


Fig. 1
Changes of blood glucose levels and body weights after streptozotocin (STZ) treatment in rats. After overnight fasting, an intraperitoneal injection of a vehicle (Control) or STZ (60 mg/kg) was performed. Nefopam was injected intraperitoneally at a dose of 30 mg/kg 30 min before the administration of STZ (Nefo-STZ). The induction of diabetes was assessed by a serial measurement of the tail vein blood glucose level using a blood glucose meter (A). Body weight was also measured (B). The data are presented as the means ± standard error. *P < 0.05, **P < 0.01 and ***P < 0.001 compared to the control group.
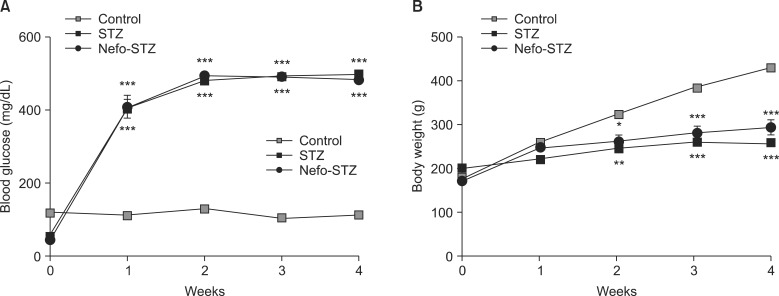
Fig. 2
Effect of a nefopam pretreatment on the withdrawal response to mechanical and thermal stimuli in streptozotocin (STZ)-induced diabetic rats. Pretreatment with nefopam (30 mg/kg) was given intraperitoneally 30 min prior to an intraperitoneal injection of STZ (60 mg/kg). Behavioral tests were performed before, and 1 to 4 weeks after the final administration of drugs. Systemic pretreatment with 30 mg/kg of nefopam significantly inhibited STZ-induced mechanical allodynia at 4 weeks (A). Pretreatment with nefopam significantly inhibited STZ-induced cold allodynia from 2 weeks after the nefopam treatment (B). However, a nefopam pretreatment did not attenuate STZ-induced thermal hyperalgesia (C). The data are presented as the means ± standard error. *P < 0.05, **P < 0.01 and ***P < 0.001 compared to the control group. †P < 0.05 and ††P < 0.01 compared to the STZ group.
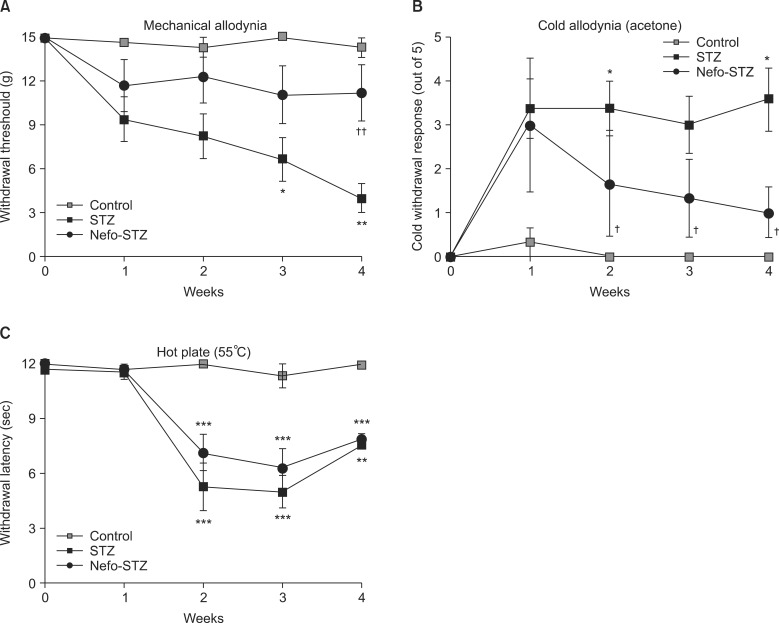
Fig. 3
Effect of a nefopam pretreatment on the transient receptor potential ankyrin 1 (TRPA1) and melastatin 8 (TRPM8) protein expression levels in the dorsal root ganglion (DRG) of streptozotocin (STZ)-induced diabetic rats. Pretreatment with nefopam (30 mg/kg) was performed intraperitoneally 30 min prior to an intraperitoneal injection of STZ (60 mg/kg). To evaluate the expressions of TRPA1 (A) and TRPM8 (B) proteins located in the L4-L6 DRG, a Western blot analysis was performed 4 weeks after the final drug administration. The specific signals for TRPA1 and TRPM8 were quantified and plotted (lower panel). β-actin was used as an internal loading control. STZ injections did not significantly affect the basal level of the TRPA1 protein in the DRG, which was not altered by the nefopam pretreatment. The TRPM8 protein level in the DRG significantly increased 4 weeks after the STZ injection, which was reduced by the nefopam pretreatment. ***P < 0.001 compared to the control group. †††P < 0.001 compared to the STZ group.
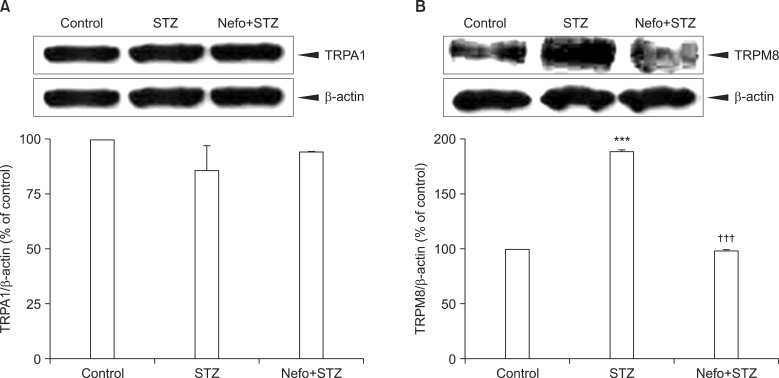
Fig. 4
Typical double immunofluorescence staining of transient receptor potential melastatin 8 (TRPM8) and neurofilament 200 (NF200) in the rat dorsal root ganglion (DRG) of streptozotocin (STZ)-induced diabetic rats. Pretreatment with nefopam (30 mg/kg) was performed intraperitoneally 30 min prior to an intraperitoneal injection of STZ (60 mg/kg). Four weeks after the final drug treatment, L5 DRG samples were immunostained with TRPM8 and neurofilament 200 (NF200), a marker of myelinated neurons. The colocalization of TRPM8 was visualized in a merged image.
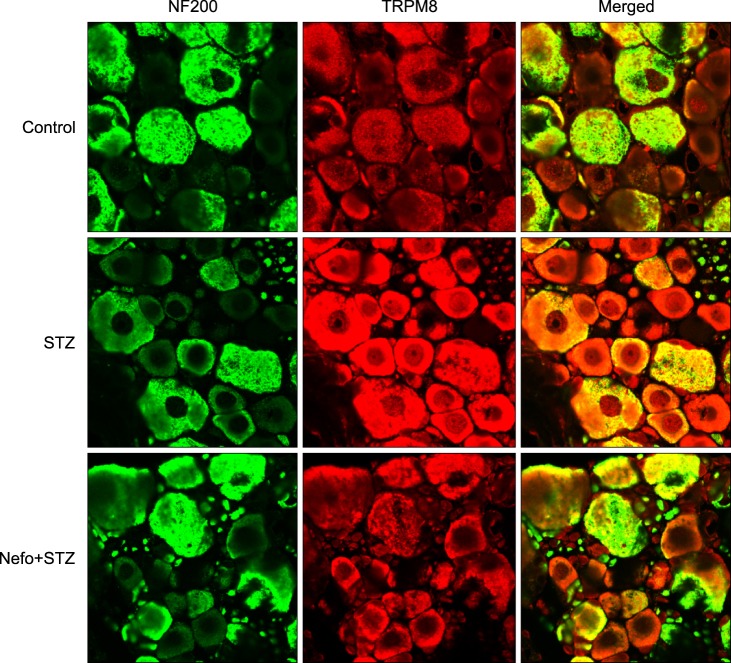