Abstract
Background
It has been reported that curcumin, the main active compound of Curcuma longa, also known as turmeric, exhibits antinociceptive properties. The aim of this study was to examine the participation of ATP-sensitive potassium channels (KATP channels) and, in particular, that of the L-arginine-nitric oxide-cyclic GMP-KATP channel pathway, in the antinociceptive effect of curcumin.
Methods
Pain was induced by the intraplantar injection of 1% formalin in the right hind paw of Wistar rats. Formalin-induced flinching behavior was interpreted as an expression of nociception. The antinociceptive effect of oral curcumin was explored in the presence and absence of local pretreatment with L-NAME, an inhibitor of nitric oxide synthase, ODQ, an inhibitor of soluble guanylyl cyclase, and glibenclamide, a blocker of KATP channels.
Results
Oral curcumin produced a dose-dependent antinociceptive effect in the 1% formalin test. Curcumin-induced antinociception was not altered by local L-NAME or ODQ, but was significantly impaired by glibenclamide.
Conclusions
Our results confirm that curcumin is an effective antinociceptive agent. Curcumin-induced antinociception appears to involve the participation of KATP channels at the peripheral level, as local injection of glibenclamide prevented its effect. Activation of KATP channels, however, does not occur by activation of the L-arginine-nitric oxide-cGMP-KATP channel pathway.
Curcumin is the main compound obtained from the rhizoma of Curcuma longa, also known as turmeric [1]. Curcumin displays a vibrant yellow color and thus is widely used as an additive in Indian food [2]. Curcuma longa has been used in Asian traditional medicine in the treatment of a variety of inflammatory disorders [3]. Furthermore, it has also been reported that curcumin exhibits antitumor, antioxidant, and antimicrobial activities [4]. It has been observed that curcumin is well tolerated after long-term use and at high doses [3,5]. However, adequately designed clinical studies specifically addressing curcumin safety are still lacking [6].
There is evidence that curcumin also appears to be an effective analgesic agent [7]. It has recently been reported that curcumin reduces pain and fatigue in patients undergoing laparoscopic cholecystectomy [8]. Animal studies have confirmed the efficacy of curcumin in pain relief. Systemic administration of curcumin induces significant antinociception in experimental models of neuropathic pain [9,10], acetic acid-induced visceral nociception [11], capsaicin-induced thermal hyperalgesia [12], and the formalin-induced orofacial pain test [13]. Several mechanisms of action have been proposed for the antinociceptive effects of curcumin, including suppression of brain nitrite and TNFα levels [9], activation of the descending monoamine system [10], blockade of TRPV1 activation [12], and desensitization of TRPA1 [14]. These hypotheses notwithstanding, however, the mechanism of antinociceptive action of curcumin has not yet been fully elucidated.
It has been reported that activation of ATP-sensitive potassium channels (KATP channels) antagonizes nociceptive responses evoked by noxious stimuli, dampening the hyperexcitability of the nociceptors [15]. There is evidence that the opening of KATP channels participates in the analgesic effect of the non-steroidal anti-inflammatory drugs ketorolac [16] and diclofenac [17, 18], but not in that of indomethacin [17]. There is also evidence that KATP channels play a role in the analgesic effect of a variety of agents such as morphine [19], clonidine [20] and 5-HT1 agonists [21], atrial natriuretic peptide (ANP) [17], and pregabalin [22]. Therefore, it appears that the opening of KATP channels is a common mechanism of antinociception.
There are several intracellular pathways that lead to the opening of KATP channels and to analgesia. Nitric oxide donors, such as sodium nitroprusside, activate the soluble form of guanylyl cyclase, resulting in the synthesis of cyclic GMP (cGMP), which in turn results in the opening of KATP channels and analgesia [23]. Ketorolac [16] and diclofenac [17] are able to produce antinociception by activation of the L-arginine-nitric oxide-cGMP-KATP channel pathway. On the other hand, ANP activates the particulate form of guanylyl cyclase [24], resulting in cGMP synthesis, which leads to KATP channel opening and antinociception [17]. There is also evidence that compounds that are able to directly open KATP channels, such as cromakalim [25] and pinacidil [17], result in pain relief. It is therefore possible that KATP channels are involved in the antinociceptive effect of curcumin. Hence, the purpose of this study was to examine whether KATP channel opening, and, in particular, the activation of the L-arginine-nitric oxide-cGMP-KATP channel pathway, is involved in curcumin-induced pain-relief.
Female Wistar rats aged 8-9 weeks (weight range 200-220 g) from our own breeding facilities were used in this study [17,18]. The animals had free access to drinking water and were fasted for 18-20 hours before experiments. Efforts were made to minimize animal suffering and to reduce the number of animals used. Rats were used one time only. At the end of the experiments, the rats were sacrificed in a CO2 chamber. All experiments followed the Guidelines on Ethical Standards for Investigation of Experimental Pain in Animals [26] and the protocol was approved by the Institutional Animal Care and Use Committee (Center for Research and Advanced Studies of the National Polytechnic Institute, Mexico, DF).
Curcumin, NG-nitro-L-arginine methyl ester (L-NAME), 1H-[1,2,4]oxadiazolo[4,3-a]quinoxaline-1-one (ODQ) and glibenclamide were purchased from Sigma Aldrich (St. Louis, MO, USA). Curcumin was suspended in carboxymethylcellulose (0.5% CMC). Glibenclamide and ODQ were dissolved in 20% dimethyl sulfoxide and L-NAME in isotonic saline.
Antinociception was assessed using the formalin test. Rats were placed in open Plexiglas observation chambers for 30 min. to allow them to accommodate to their surroundings; they were then removed for formalin administration. Fifty microliters of diluted formalin (1%) were injected subcutaneously into the dorsal surface of the right hind paw with a 30-gauge needle. Animals were then returned to the chambers and nociceptive behavior was observed immediately after formalin injection. Mirrors were placed behind each chamber to enable unhindered observation of the formalin-injected paw. Nociceptive behavior was quantified as the numbers of flinches of the injected paw during 1 min. periods every 5 min. for up to 60 min. after injection [25-28]. Flinching was readily identified and characterized as a rapid and brief withdrawal or flexing of the injected paw. Formalin-induced flinching behavior is biphasic [17,27-29]. The initial acute phase (0-10 min.) is followed by a relatively short quiescent period, which is then followed by a prolonged tonic response (15-60 min.). At the end of the experiment, the animals were sacrificed in a CO2 chamber.
Rats received vehicle or increasing oral doses of curcumin (3.1-100 mg/kg) by gavage 60 min. before formalin injection. In order to examine the participation of the NO-cGMP-KATP channel pathway in the antinociceptive effect of oral curcumin, rats were pretreated locally with L-NAME, an inhibitor of nitric oxide synthase, ODQ, an inhibitor of soluble guanylyl cyclase, or glibenclamide, a blocker of KATP channels [17,28,29]. Blockers dissolved in a volume of 50 µl were injected in the paw that was to be injured 10 min. before the insult, as described previously [17].
All results are presented as mean ± SEM. Curves were made by plotting the number of flinches against time. The area under the number of flinches against time curves (AUC) was calculated by the trapezoidal rule [17,28-30]. AUC was calculated for the two phases of the assay, and the percent of antinociception for each phase was calculated according to the following equation [30]:
Percent of antinociception = [(AUCvehicle - AUCpost compound)/AUCvehicle] × 100.
Analysis of variance followed by Tukey's test was used to compare the differences between treatments. Differences were considered statistically significant when P < 0.05.
Local injection of 1% formalin produced a typical biphasic flinching behavior. The first phase was characterized by an immediate increase in flinching followed by a gradual decrease, to reach near-zero values after 10 min. A second flinching phase then occurred, being observed from 15 min. to 60 min. Oral curcumin administration did not have any significant effect on formalin-induced nociception during phase 1, but significantly reduced flinching during phase 2 (Fig. 1A). Therefore, only data from phase 2 were submitted for further analysis. Curcumin produced a dose-dependent reduction in the AUC of phase 2 (Fig. 1B). The effect of curcumin on the second phase of the formalin test can also be expressed as a dose-dependent increase in the percent of antinociception (Fig. 1C).
In an additional experimental series, it was observed that pretreatment with local injection of L-NAME or ODQ did not produce any significant change in oral curcumin-induced antinociception (Figs. 2A and 2B), while pretreatment with glibenclamide resulted in a dose-dependent reduction in the antinociceptive effect of 100 mg/kg oral curcumin (Fig. 2C).
Curcumin, the main active ingredient of turmeric, exhibits several pharmacological actions [1-5]. It has been documented that curcumin exhibits a significant antinociceptive effect in a variety of experimental pain models [9-13]. Moreover, it has recently been reported that curcumin is effective in postsurgical pain [8] and therefore exhibits true potential as an analgesic agent in clinical practice [7]. Several mechanisms have been proposed to explain the antinociceptive effect of curcumin, including suppression of brain nitrite and TNFα levels [9], activation of the descending monoamine system [10], blockade of TRPV1 activation [12], and desensitization of TRPA1 [14]. However, the exact mechanism of action remains controversial [12]. Moreover, it is possible that the overall antinociceptive effect of curcumin involves multiple mechanisms of action, as is the case for several analgesic agents, such as diclofenac [31].
It is now well documented that the opening of KATP channels plays a role in the antinociceptive effect of a number of agents, including ketorolac [16], diclofenac [17,18], morphine [19], clonidine [20], 5-HT1 agonists [21], ANP [17], and pregabalin [22]. It is known that activation of KATP channels antagonizes nociceptive responses evoked by noxious stimuli, dampening the hyperexcitability of the nociceptors [15]. However, KATP channel opening is not a universal mechanism of antinociception. Several agents, such as indomethacin [17], acemetacin [32], and kappa opioid receptor agonists [33] induce a significant antinociceptive effect which does not involve the participation of KATP channels. In this study, we demonstrate that the activation of KATP channels is involved in the antinociceptive effect of curcumin.
KATP channels can be activated by several pathways. Compounds that directly open the channels, such as diazoxide [34], pinacidil [17], and cromakalim [33] produce a significant antinociceptive effect. KATP channel opening also occurs as a consequence of activation of Gi/o proteins, as is the case for µ- and δ-opioid agonists [33]. Moreover, an increase in intracellular cGMP also results in KATP channel opening and analgesia. This is observed after administration of dibutyryl-cGMP [34,35] or by activation of cGMP-generating pathways. ANP, which directly activates the particulate form of guanylyl cyclase, results in significant antinociception [17]. It is also well documented that activation of the L-arginine-nitric oxide-cGMP-KATP channel pathway results in antinociception. Administration of nitric oxide donors, such as sodium nitroprusside [23], induces a significant antinociceptive effect. KATP channel opening and antinociception is also induced by hydrogen sulfide [36].
Local administration of L-NAME, ODQ, or glibenclamide does not induce any significant alteration in formalin-induced nociception, suggesting that these compounds are devoid of any pro- or antinociceptive effects by themselves, although they can interfere with the antinociceptive action of several drugs [17,18,29]. In the present study, we observed that glibenclamide, but not L-NAME or ODQ, impairs the antinociceptive effect of oral curcumin. These results suggest that activation of KATP channels, but not by the L-arginine-nitric oxide-cGMP-KATP channel pathway, is involved in the antinociceptive effect of curcumin. Hence, curcumin may be directly stimulating KATP channels, activating Gi/o proteins, stimulating the particulate form of guanylyl cyclase, or acting through the hydrogen sulfide-KATP channel pathway. Further research is needed to fully elucidate this issue.
The local injection of glibenclamide in the same site as the formalin insult was able to significantly reduce the antinociceptive effect of oral curcumin. There is evidence that glibenclamide is able to block drug-induced antinociception after local and spinal administration [17,29], but also after systemic administration [18]. Hence, our results provide evidence that curcumin-induced antinociception involves the participation of KATP channels at the peripheral level, although additional systemic mechanisms of action may also be involved.
Recently, Han and coworkers reported that intrathecal administration of curcumin produces antinociception in the formalin test [37], demonstrating that this agent is also able to produce antinociception by central mechanisms. There is evidence that curcumin is able to pass through the blood-brain barrier [38]. Therefore, it is likely that the overall antinociceptive effect of curcumin after systemic administration involves both peripheral and central actions, as is the case for non-steroidal anti-inflammatory drugs and opioids [31]. Furthermore, for several agents, such as diclofenac [28] and lumiracoxib [29], we have shown that both the peripheral and central actions include the participation of KATP channels.
In summary, the present results confirm that curcumin is an effective antinociceptive agent. Our data provide evidence for the participation of peripheral mechanisms, including KATP channel opening, in addition to other mechanisms of action. The complete elucidation of the mechanisms of action of the antinociceptive effect of curcumin will certainly bolster its potential as an analgesic agent in clinical practice.
ACKNOWLEDGEMENTS
This study was supported by Cinvestav and by the National Polytechnic Institute, grant SIP20121164. Marco Antonio De Paz Campos y a fellow of the National Council for Science and Technology (CONACYT).
References
1. Rivera-Espinoza Y, Muriel P. Pharmacological actions of curcumin in liver diseases or damage. Liver Int. 2009; 29:1457–1466. PMID: 19811613.


2. Basnet P, Skalko-Basnet N. Curcumin: an anti-inflammatory molecule from a curry spice on the path to cancer treatment. Molecules. 2011; 16:4567–4598. PMID: 21642934.


3. Goel A, Kunnumakkara AB, Aggarwal BB. Curcumin as "Curecumin": from kitchen to clinic. Biochem Pharmacol. 2008; 75:787–809. PMID: 17900536.


4. Aggarwal BB, Sung B. Pharmacological basis for the role of curcumin in chronic diseases: an age-old spice with modern targets. Trends Pharmacol Sci. 2009; 30:85–94. PMID: 19110321.


5. Chainani-Wu N. Safety and anti-inflammatory activity of curcumin: a component of tumeric (Curcuma longa). J Altern Complement Med. 2003; 9:161–168. PMID: 12676044.


6. Burgos-Morón E, Calderón-Montaño JM, Salvador J, Robles A, López-Lázaro M. The dark side of curcumin. Int J Cancer. 2010; 126:1771–1775. PMID: 19830693.


7. Kapoor S. Curcumin and its emerging role in pain modulation and pain management. Korean J Pain. 2012; 25:202–203. PMID: 22787555.


8. Agarwal KA, Tripathi CD, Agarwal BB, Saluja S. Efficacy of turmeric (curcumin) in pain and postoperative fatigue after laparoscopic cholecystectomy: a double-blind, randomized placebo-controlled study. Surg Endosc. 2011; 25:3805–3810. PMID: 21671126.


9. Sharma S, Kulkarni SK, Agrewala JN, Chopra K. Curcumin attenuates thermal hyperalgesia in a diabetic mouse model of neuropathic pain. Eur J Pharmacol. 2006; 536:256–261. PMID: 16584726.


10. Zhao X, Xu Y, Zhao Q, Chen CR, Liu AM, Huang ZL. Curcumin exerts antinociceptive effects in a mouse model of neuropathic pain: descending monoamine system and opioid receptors are differentially involved. Neuropharmacology. 2012; 62:843–854. PMID: 21945716.


11. Tajik H, Tamaddonfard E, Hamzeh-Gooshchi N. The effect of curcumin (active substance of turmeric) on the acetic acid-induced visceral nociception in rats. Pak J Biol Sci. 2008; 11:312–314. PMID: 18817212.


12. Yeon KY, Kim SA, Kim YH, Lee MK, Ahn DK, Kim HJ, et al. Curcumin produces an antihyperalgesic effect via antagonism of TRPV1. J Dent Res. 2010; 89:170–174. PMID: 20040737.


13. Mittal N, Joshi R, Hota D, Chakrabarti A. Evaluation of antihyperalgesic effect of curcumin on formalin-induced orofacial pain in rat. Phytother Res. 2009; 23:507–512. PMID: 19051211.


14. Leamy AW, Shukla P, McAlexander MA, Carr MJ, Ghatta S. Curcumin ((E,E)-1,7-bis(4-hydroxy-3-methoxyphenyl)-1,6-heptadiene-3,5-dione) activates and desensitizes the nociceptor ion channel TRPA1. Neurosci Lett. 2011; 503:157–162. PMID: 21855605.


15. Du X, Wang C, Zhang H. Activation of ATP-sensitive potassium channels antagonize nociceptive behavior and hyperexcitability of DRG neurons from rats. Mol Pain. 2011; 7:35. PMID: 21569593.


16. Lázaro-Ibáñez GG, Torres-López JE, Granados-Soto V. Participation of the nitric oxide-cyclic GMP-ATP-sensitive K(+) channel pathway in the antinociceptive action of ketorolac. Eur J Pharmacol. 2001; 426:39–44. PMID: 11525769.


17. Ortiz MI, Granados-Soto V, Castañeda-Hernández G. The NO-cGMP-K+ channel pathway participates in the antinociceptive effect of diclofenac, but not of indomethacin. Pharmacol Biochem Behav. 2003; 76:187–195. PMID: 13679232.


18. León-Reyes MR, Castañeda-Hernández G, Ortiz MI. Pharmacokinetics and pharmacodynamics of diclofenac in the presence and absence of glibenclamide in the rat. J Pharm Pharm Sci. 2008; 11:68–76.


19. Ocaña M, Del Pozo E, Barrios M, Robles LI, Baeyens JM. An ATP-dependent potassium channel blocker antagonizes morphine analgesia. Eur J Pharmacol. 1990; 186:377–378. PMID: 2127024.


20. Ocaña M, Baeyens JM. Differential effects of K+ channel blockers on antinociception induced by alpha 2-adrenoceptor, GABAB and kappa-opioid receptor agonists. Br J Pharmacol. 1993; 110:1049–1054. PMID: 7905339.


21. Robles LI, Barrios M, Del Pozo E, Dordal A, Baeyens JM. Effects of K+ channel blockers and openers on antinociception induced by agonists of 5-HT1A receptors. Eur J Pharmacol. 1996; 295:181–188. PMID: 8720582.


22. Kweon TD, Kim JY, Kwon IW, Choi JB, Lee YW. Participation of K(ATP) channels in the antinociceptive effect of pregabalin in rat formalin test. Korean J Pain. 2011; 24:131–136. PMID: 21935490.


23. Soares AC, Leite R, Tatsuo MA, Duarte ID. Activation of ATP-sensitive K(+) channels: mechanism of peripheral antinociceptive action of the nitric oxide donor, sodium nitroprusside. Eur J Pharmacol. 2000; 400:67–71. PMID: 10913586.


24. White RE, Lee AB, Shcherbatko AD, Lincoln TM, Schonbrunn A, Armstrong DL. Potassium channel stimulation by natriuretic peptides through cGMP-dependent dephosphorylation. Nature. 1993; 361:263–266. PMID: 7678699.


25. Ocaña M, Barrios M, Baeyens JM. Cromakalim differentially enhances antinociception induced by agonists of alpha(2)-adrenoceptors, gamma-aminobutyric acid(B), mu and kappa opioid receptors. J Pharmacol Exp Ther. 1996; 276:1136–1142. PMID: 8786544.
26. Zimmermann M. Ethical guidelines for investigations of experimental pain in conscious animals. Pain. 1983; 16:109–110. PMID: 6877845.


27. Jeong HJ, Lee SH, Cho SY, Lee CS, Jeong CW, Yoon MH, et al. Roles of serotonergic and adrenergic receptors in the antinociception of selective cyclooxygenase-2 inhibitor in the rat spinal cord. Korean J Pain. 2011; 24:179–184. PMID: 22220238.


28. Ortiz MI, Lozano-Cuenca J, Granados-Soto V, Castañeda-Hernández G. Additive interaction between peripheral and central mechanisms involved in the antinociceptive effect of diclofenac in the formalin test in rats. Pharmacol Biochem Behav. 2008; 91:32–37. PMID: 18602417.


29. Lozano-Cuenca J, Castañeda-Hernández G, Granados-Soto V. Peripheral and spinal mechanisms of antinociceptive action of lumiracoxib. Eur J Pharmacol. 2005; 513:81–91. PMID: 15878712.


30. Ortiz MI, Ramírez-Montiel ML, González-García MP, Ponce-Monter HA, Castañeda-Hernández G, Cariño-Cortés R. The combination of naproxen and citral reduces nociception and gastric damage in rats. Arch Pharm Res. 2010; 33:1691–1697. PMID: 21052946.


31. Jiménez-Andrade JM, Ortiz MI, Pérez-Urizar J, Aguirre-Bañuelos P, Granados-Soto V, Castañeda-Hernández G. Synergistic effects between codeine and diclofenac after local, spinal and systemic administration. Pharmacol Biochem Behav. 2003; 76:463–471. PMID: 14643845.


32. Gil-Flores M, Ortiz MI, Castañeda-Hernández G, Chávez-Piña AE. Acemetacin antinociceptive mechanism is not related to NO or K+ channel pathways. Methods Find Exp Clin Pharmacol. 2010; 32:101–105. PMID: 20401346.


33. Ocaña M, Cendán CM, Cobos EJ, Entrena JM, Baeyens JM. Potassium channels and pain: present realities and future opportunities. Eur J Pharmacol. 2004; 500:203–219. PMID: 15464034.


34. Alves DP, Soares AC, Francischi JN, Castro MS, Perez AC, Duarte ID. Additive antinociceptive effect of the combination of diazoxide, an activator of ATP-sensitive K+ channels, and sodium nitroprusside and dibutyryl-cGMP. Eur J Pharmacol. 2004; 489:59–65. PMID: 15063156.


35. Soares AC, Duarte ID. Dibutyryl-cyclic GMP induces peripheral antinociception via activation of ATP-sensitive K(+) channels in the rat PGE2-induced hyperalgesic paw. Br J Pharmacol. 2001; 134:127–131. PMID: 11522604.


36. Distrutti E, Sediari L, Mencarelli A, Renga B, Orlandi S, Antonelli E, et al. Evidence that hydrogen sulfide exerts antinociceptive effects in the gastrointestinal tract by activating KATP channels. J Pharmacol Exp Ther. 2006; 316:325–335. PMID: 16192316.


37. Han YK, Lee SH, Jeong HJ, Kim MS, Yoon MH, Kim WM. Analgesic effects of intrathecal curcumin in the rat formalin test. Korean J Pain. 2012; 25:1–6. PMID: 22259709.


38. Tsai YM, Chien CF, Lin LC, Tsai TH. Curcumin and its nano-formulation: the kinetics of tissue distribution and blood-brain barrier penetration. Int J Pharm. 2011; 416:331–338. PMID: 21729743.


Fig. 1
(A) Flinching behavior observed after the intraplantar injection of 1% formalin in the right hind paw of Wistar rats in absence (black symbols) of in presence (open symbols) of 100 mg/kg oral curcumin. (B) Area under the number of flinches-against-time curve of the second phase of the formalin test observed in Wistar rats in presence of increasing doses of oral curcumin. (C) Percent of antinociception observed in the second phase of the formalin test in presence of increasing doses of oral curcumin. Data are presented as mean ± SEM of six animals. *Denotes a statistically significant difference (P < 0.05) with respect to the effect observed in absence of curcumin.
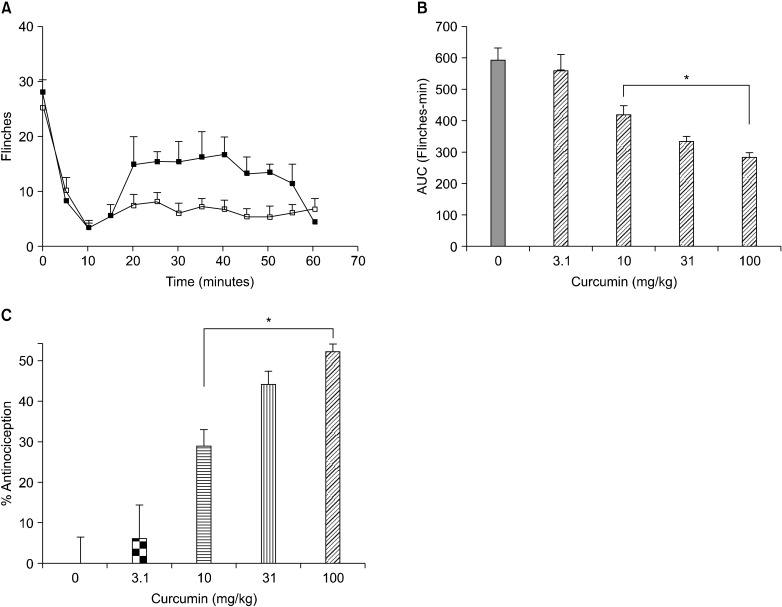
Fig. 2
Antinociceptive effect of 100 mg/kg oral curcumin in the second phase of the formalin test in Wistar rats submitted to local pretreatment with increasing doses of L-NAME (A), ODQ (B) and glibenclamide (C). Data are presented as mean ± SEM of six animals. *Denotes a statistically significant difference (P < 0.05) with respect to the effect observed in absence of glibenclamide.
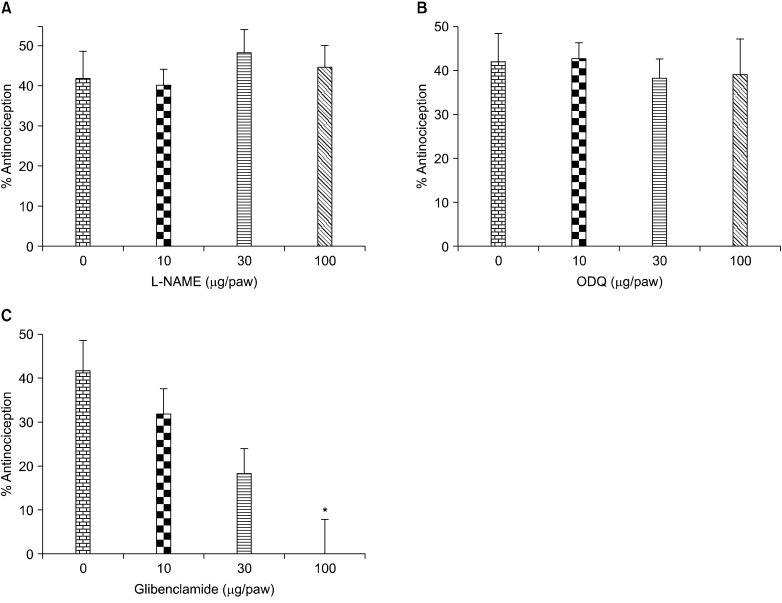