Abstract
Background
It has been demonstrated that the expression of tumor necrosis factor-α (TNF-α) and apoptotic cell death in the dorsal root ganglion (DRG) following spinal nerve constriction injury play a role in the initiation and continuation of hyperalgesia and allodynia. The present study was designed to investigate the effects of ethyl pyruvate (EP) on mechanical and cold allodynia, TNF-α expression, and apoptosis in DRG after spinal nerve ligation injury.
Methods
Rats were divided into 3 groups: control, pre-EP, and post-EP. EP (50 mg/kg) was intraperitoneally injected 30 minutes before (pre-EP) or after (post-EP) surgery. Behavioral tests to determine mechanical and cold allodynia were conducted before surgery and 4 and 7 days after surgery. Seven days after surgery, TNF-α protein levels in DRG were evaluated by enzyme-linked immunosorbent assay, and DRG apoptosis was determined by immunohistochemical detection of activated caspase-3.
Results
Treatment with EP significantly reduced mechanical and cold allodynia following spinal nerve ligation injury. TNF-α protein levels in the pre-EP (4.7 ± 1.2 pg/200 µg; P < 0.001) and post-EP (6.4 ± 1.8 pg/200 µg; P < 0.001) groups were 2-3 times lower than the control group (14.4 ± 1.2 pg/200 µg). The percentages of neurons and satellite cells that co-localized with caspase-3 were also significantly lower in the pre-EP and post-EP groups than the control group.
Neuropathic pain that consists of hyperalgesia, spontaneous pain, and mechanical and thermal allodynia may result from peripheral nerve injury. Unilateral tight ligation of the fifth and sixth lumbar (L5 and L6) spinal nerves reproduces some of the representative features of neuropathic pain [1]. Previous studies have provided evidence of the release of pro-inflammatory cytokines after nerve injury in relation to neuropathic pain, including tumor necrosis factor-α (TNF-α) [2-11]. The increase in TNF-α expression has been observed in the dorsal root ganglion (DRG) after peripheral nerve injury [2-6]. Application of TNF-α to normal or compression-injured DRG results in mechanical allodynia [7-9]. Furthermore, the inhibition of TNF-α by selective antibody therapy attenuates mechanical allodynia in animals with spinal nerve ligation injury [10,11].
Apoptosis is also evident in DRG neurons following peripheral nerve injury, although the mechanism responsible for producing it is uncertain. However, another major factor may be associated with the cytokine signaling pathway of apoptosis secondary to the axonal transport of pro-inflammatory cytokines [2,12] and the activation of neuronal apoptosis [13,14]. Caspase-3, which plays a major role in the proteolytic cleavage of many key proteins, is a key indicator of apoptosis. Previous studies have reported that the inhibition of caspase activity attenuates pain-related behavior [14,15].
Ethyl pyruvate (EP) is a stable, lipophilic derivative of pyruvic acid with known anti-inflammatory and antioxidant properties. EP reduces brain and spinal cord injuries in cerebral and spinal cord ischemic/reperfusion-induced injury models and spinal cord compression injury models [16-18]. In addition, EP inhibits the release of TNF-α in brain and lung injury models [19,20] and reduces apoptosis in various models of organ injury [18,21,22]. Therefore, we hypothesized that EP would have a beneficial effect on neuropathic pain following peripheral nerve injury. The specific aim of this study was to evaluate the preemptive analgesic effects of EP on mechanical and cold allodynia, TNF-α expression, and apoptosis in DRG after spinal nerve ligation injury.
The experimental procedures were approved by the animal care committee of our hospital. Male Sprague-Dawley rats (160-180 g) were housed 3 per cage in a temperature-controlled (21 ± 1℃) vivarium with a 12-hour light/dark cycle. Rats were provided water and food ad libitum and were permitted to acclimate for 3 days prior to use in experiments.
The rats were randomly divided into 3 groups (21 rats per group): 1) the control group, which received only spinal nerve ligation injury; 2) the pre-EP group, which received spinal nerve ligation injury plus an intraperitoneally (ip) administered dose of EP (50 mg/kg) 30 minutes before surgery; and 3) the post-EP group, which received spinal nerve ligation injury plus an ip administered dose of EP (50 mg/kg) 30 minutes after surgery. EP (98% pure; Sigma-Aldrich Co., St. Louis, MO, USA) was dissolved in normal saline to a concentration of 10 mg/ml.
Behavioral tests were performed before and 4 and 7 days after surgery in order to measure behavioral responses to mechanical and cold stimuli. TNF-α protein levels in DRG were determined by enzyme-linked immunosorbent assay (ELISA), and DRG apoptosis was evaluated by immunohistochemical detection of activated caspase-3 on postoperative day 7. All testing was performed by 1 examiner who was blind to the study groups.
The rats were anesthetized by ip injection of zoletil (12.5 mg) and xylazine (3 mg). Spinal nerve ligation injury was performed according to the procedure of a previously published report [1]. Briefly, a midline skin incision to the lower back and deep dissection through the paraspinal muscles was performed, revealing the left L6 transverse process, which was then removed. The left L5/6 spinal nerves were exposed and both nerves were tightly ligated using 6-0 black silk.
Behavioral testing was performed at the same time during the day (10:00 AM-4:00 PM). Rats were positioned in individual plastic cages with wire-mesh bottoms and permitted to acclimate for 20 minutes. Baseline testing was performed before surgery to verify that the animal responses were normal. All rats were tested 4 and 7 days after surgery.
To test for mechanical allodynia, a von Frey filament (Stoelting, Wood Dale, IL, USA) was used to stimulate the plantar surface of the left hind foot. Eight calibrated von Frey filaments (0.41, 0.70, 1.20, 2.00, 3.63, 5.50, 8.50, and 15.10 g) were sequentially applied to the foot (in ascending or descending order) and gently pressed against the foot. Rapid withdrawal or flinching was interpreted as a positive response, in which case the next-lightest filament was tested; a negative response led to the application of the next-heaviest filament. Determination of the 50% withdrawal threshold was made using the up-down method, as previously described [23]. Rats that demonstrated a withdrawal threshold of less than 5.0 g were classified as demonstrating mechanical allodynia.
Response to cold stimuli was examined using the acetone method as previously reported [3]. Briefly, a drop of acetone was lightly placed onto the plantar surface of the left hind foot. Acetone was applied 5 times to the hind foot at 5-minute intervals. Rapid withdrawal in response to the application of acetone was considered a positive sign. The percentage of the withdrawal response was calculated by dividing the number of foot withdrawals by 5 (the total number of trials) and multiplying the result by 100. Rats that showed a withdrawal response greater than 40% were classified as demonstrating cold allodynia.
An ELISA immunoassay was used to analyze the 42 rats used in the behavioral tests (n = 14 rats per group), as previously described [3]. Briefly, rats were rapidly decapitated under anesthesia on postoperative day 7, and the left L5/6 DRGs were removed and frozen in liquid nitrogen. Tissue samples (each sample contained L5/6 DRGs from 2 rats) were homogenized in ice-cold RiPA buffer (R0278; Sigma, St. Louis, MO, USA) that contained a protease inhibitor cocktail (P8340; Sigma), then centrifuged (13,000 rpm for 20 minutes) to obtain protein extracts. Commercially available ELISA kits (Quantikine Rat TNF-α/TNFSF1A Immunoassays, R&D System, Minneapolis, MN, USA; sensitivity: 5 pg/ml) were used to measure the TNF-α protein levels in DRGs. The total protein concentrations of the tissue supernatant were determined using the Bradford protein assay. Microplates were assayed using a Victor3 V Multilabel Counter (Model 1420; Perkin Elmer, Waltham, MA, USA). Data were standardized in terms of picograms of TNF-α per 200 µg of total protein in the supernatant.
The histological findings in the 21 rats used in the behavioral tests were also analyzed (n = 7 rats per group), as previously described [3]. Briefly, under anesthesia, the rats were transcardially perfused with 4% buffered paraformaldehyde on postoperative day 7. The left L5 DRG was collected and subsequently fixed in 4% paraformaldehyde, embedded in paraffin, and sectioned to a thickness of 4 µm. After blocking with 5% goat serum, 0.3% Triton X-100, and 1% bovine serum albumin in phosphate-buffered saline (PBS-T), double-label immunofluorescence was conducted by incubating the paraffin sections overnight at 4℃ with cleaved caspase-3 (Asp 175) antibody (1:200; #9661; Cell Signaling Technology Inc., Danvers, MA, USA) and GFAP antibody (1:200; #3670; Cell Signaling) or NeuN antibody (1:200; MAB377; Chemicon, Temecula, CA, USA), followed by incubation with Alexa Flour 555 goat anti-rabbit IgG (red; 1:500; Invitrogen, Carlsbad, CA, USA) and Alexa Flour 488 goat anti-mouse IgG (green; 1:500; Invitrogen) for 2 hours at 20℃. The immunostained DRG sections were observed using a confocal microscope. Two sections were used in each evaluation to count the number of NeuN-immunoreactive (IR), GFAP-IR, and double-labeled cells at 400× magnification. The percentage of double-labeled cells in each DRG was calculated by dividing the number of double-labeled cells by the total number of NeuN-IR and GFAP-IR cells within the field and multiplying the result by 100.
The data are presented as the means ± standard deviations. Behavioral data (withdrawal threshold to mechanical stimuli and withdrawal response to cold stimuli) were evaluated by 2-way repeated measures analysis of variance. TNF-α protein levels and the percentage of double-labeled cells in the DRGs were analyzed using the Kruskal-Wallis test followed by the Mann-Whitney U-test to evaluate the between-group differences. P values less than 0.05 were considered significant.
Treatment with EP reduced sensitivity to mechanical and cold stimuli after spinal nerve ligation injury (Fig. 1). The withdrawal threshold to mechanical stimuli was significantly increased in both the pre-EP (P < 0.001) and post-EP (P < 0.001) groups on postoperative days 4 and 7 in comparison with the control group. The withdrawal response to cold stimuli was significantly lower in both treatment groups on postoperative days 4 and 7 in comparison with the control group (P < 0.001 vs. both treatment groups).
The TNF-α expression levels in the ipsilateral DRGs on postoperative day 7 are shown in Fig. 2. The levels of TNF-α in the pre-EP (4.7 ± 1.2 pg/200 µg; P < 0.001) and post-EP (6.4 ± 1.8 pg/200 µg; P < 0.001) groups were significantly lower than the control group (14.4 ± 1.2 pg/200 µg).
NeuN-IR cells (DRG neurons, green) and GFAP-IR cells (activated satellite cells, green) were detected in the ipsilateral DRG (Fig. 3A, D). GFAP-IR satellite cells were localized around DRG neurons. In all groups, caspase-3 immunoreactivity (red) was observed in DRG neurons and satellite cells (Fig. 3B, E). Activated caspase-3 was co-localized with NeuN (Fig. 3C) and GFAP (Fig. 3F). The percentages of neurons that co-localized with caspase-3 in the pre-EP (39.6% ± 8.0%; P = 0.002) and post-EP (42.6% ± 6.1%; P = 0.002) groups were significantly lower than those in the control group (73.3% ± 11.0%) (Fig. 4). A similar decrease in the percentage of satellite cells that co-localized with caspase-3 was observed in the pre-EP (36.4% ± 6.5%; P = 0.002) and post-EP (49.9% ± 8.8%; P = 0.002) groups in comparison with the control group (78.7% ± 8.0%).
In the present study, EP alleviated mechanical and cold allodynia and reduced TNF-α expression and DRG apoptosis after spinal nerve ligation injury.
Peripheral nerve injury is associated with molecular and cellular alterations to the peripheral and central nervous systems. Mechanical and thermal allodynia, and ongoing pain-related behaviors in association with peripheral nerve injury have been demonstrated to reflect, at least in part, alteration in the excitability of primary afferent neurons [24,25]. In addition, increased apoptosis in the activated neuronal and satellite cells of DRG may be associated with the induction and maintenance of neuropathic hypersensitivity [26,27].
It is well known that inflammatory cytokines, such as TNF-α, play an important role in the development of neuropathic pain following peripheral nerve injury. Increased expression of TNF-α has been observed in animal models of peripheral nerve induced neuropathic pain [3,5,6,12,26] and nerve biopsies from patients with neuropathies [28]. Exogenous application of TNF-α to normal and compression-injured DRG has been reported to induce mechanical hyperalgesia [7-9]. In addition, treatment with the TNF antagonist has been shown to attenuate mechanical allodynia following peripheral nerve injury [10,11]. In a previous study, we demonstrated that TNF-α expression levels in DRG are significantly increased in rats 7 days after spinal nerve ligation injury (data are not shown) [3].
EP, a stable, lipophilic derivative of the endogenous metabolite pyruvate, has been studied as an effective anti-inflammatory and cytoprotective agent. The proposed mechanisms of the pharmacologic effects of EP include the inhibition of redox-mediated cellular damage, the down-regulation of pro-inflammatory transcription factors, decreased expression of pro-inflammatory proteins, and the inhibition of the up-regulated expression of pro-apoptotic proteins [29]. Ulloa et al. [30] reported that the incubation of lipopolysaccharide-stimulated RAW 264.7 murine macrophage-like cells with EP inhibits the release of TNF and decreases the steady-state levels of TNF mRNA. Cho et al. [19] showed that EP reduces TNF-α expression levels in the murine hippocampus after kainic acid-induced brain injury. Shang et al observed that EP significantly reduces the release of TNF-α in an endotoxin-induced murine model of lung injury [20]. In the present study, pre- and post-treatment with EP reduced the level of TNF-α protein in DRG after spinal nerve ligation injury on day 7 post-injury, and this finding coincided with decreased withdrawal in response to mechanical and cold stimuli throughout the observation period. These results are concordant with previously reported results that demonstrate the anti-inflammatory and cytoprotective effects of EP against excitotoxic neuronal and organ damage.
Additionally, the increased expression of TNF-α is also associated with DRG apoptosis, which plays an important role in neuropathic pain following spinal nerve injury [31,32]. Caspase-3 is known to induce the proteolytic cleavage of many key proteins, and its expression is regarded as a distinct indicator of apoptosis. Caspase signaling pathways are also associated with pain, as evidenced in a report showing that the inhibition of caspases alleviates pain-related behavior; TNF-α, which up-regulates the death receptor ligand, induces pain via this pathway [14]. Treatment with EP has been previously shown to reduce spinal cord apoptosis after spinal cord compression injury [18], myocardial apoptosis after ischemic-reperfusion injury [21], and hepatic apoptosis after liver ischemic-reperfusion injury [22]. The immunohistochemical analysis presented here demonstrates that the percentage of neurons and satellite cells that are co-localized with activated caspase-3 (a potential indicator of progression to apoptosis) was significantly reduced by both pre- and post-operative EP treatment. These results suggest that EP inhibits DRG apoptosis, at least in part, via the caspase signaling pathway following spinal nerve ligation injury.
In this study, we sought to determine whether EP has a beneficial effect on mechanical and cold allodynia, TNF-α expression, and DRG apoptosis following spinal nerve ligation injury. However, we did not examine the behavioral effects of EP treatment after postoperative day 7, which is a possible limitation of the current study. Thus, it is not clear whether increases in TNF-α and apoptosis affect the recurrence of allodynia after 7 days. Therefore, further studies are needed to evaluate the anti-allodynic effect of EP beyond this point. Another potential limitation is that ELISA, which was used to determine the role of TNF-α, and the histological studies, which were used to determine the effect of apoptosis on DRG neurons, were only performed on postoperative day 7. This time point was chosen because previous studies have shown that the peak time for TNF-α expression and apoptosis is about 7 days after nerve injury [5,32,33].
In conclusion, treatment with EP alleviated mechanical and cold allodynia through the inhibition of TNF-α expression and apoptosis in DRG after spinal nerve ligation injury. Therefore, the present study suggests that EP may be an effective new drug for reducing allodynia after peripheral nerve injury.
References
1. Kim SH, Chung JM. An experimental model for peripheral neuropathy produced by segmental spinal nerve ligation in the rat. Pain. 1992; 50:355–363. PMID: 1333581.


2. Schäfers M, Geis C, Brors D, Yaksh TL, Sommer C. Anterograde transport of tumor necrosis factor-alpha in the intact and injured rat sciatic nerve. J Neurosci. 2002; 22:536–545. PMID: 11784800.


3. Kim SH, Nam JS, Choi DK, Koh WW, Suh JH, Song JG, et al. Tumor necrosis factor-alpha and apoptosis following spinal nerve ligation injury in rats. Korean J Pain. 2011; 24:185–190. PMID: 22220239.


4. Ohtori S, Takahashi K, Moriya H, Myers RR. TNF-alpha and TNF-alpha receptor type 1 upregulation in glia and neurons after peripheral nerve injury: studies in murine DRG and spinal cord. Spine (Phila Pa 1976). 2004; 29:1082–1088. PMID: 15131433.


5. Dubový P, Jancálek R, Klusáková I, Svízenská I, Pejchalová K. Intra- and extraneuronal changes of immunofluorescence staining for TNF-alpha and TNFR1 in the dorsal root ganglia of rat peripheral neuropathic pain models. Cell Mol Neurobiol. 2006; 26:1205–1217. PMID: 16705482.
6. Miao P, Madec K, Gong Y, Shen H, Eisenstat D, Melanson M, et al. Axotomy-induced up-regulation of tumor necrosis factor-alpha in the dorsal root ganglia. Neurol Res. 2008; 30:623–631. PMID: 18489817.


7. Homma Y, Brull SJ, Zhang JM. A comparison of chronic pain behavior following local application of tumor necrosis factor alpha to the normal and mechanically compressed lumbar ganglia in the rat. Pain. 2002; 95:239–246. PMID: 11839423.


8. Wagner R, Myers RR. Endoneurial injection of TNF-alpha produces neuropathic pain behaviors. Neuroreport. 1996; 7:2897–2901. PMID: 9116205.


9. Wei XH, Zang Y, Wu CY, Xu JT, Xin WJ, Liu XG. Peri-sciatic administration of recombinant rat TNF-alpha induces mechanical allodynia via upregulation of TNF-alpha in dorsal root ganglia and in spinal dorsal horn: the role of NF-kappa B pathway. Exp Neurol. 2007; 205:471–484. PMID: 17459378.


10. Sweitzer S, Martin D, DeLeo JA. Intrathecal interleukin-1 receptor antagonist in combination with soluble tumor necrosis factor receptor exhibits an anti-allodynic action in a rat model of neuropathic pain. Neuroscience. 2001; 103:529–539. PMID: 11246166.


11. Schäfers M, Lee DH, Brors D, Yaksh TL, Sorkin LS. Increased sensitivity of injured and adjacent uninjured rat primary sensory neurons to exogenous tumor necrosis factor-alpha after spinal nerve ligation. J Neurosci. 2003; 23:3028–3038. PMID: 12684490.


12. Shubayev VI, Myers RR. Axonal transport of TNF-alpha in painful neuropathy: distribution of ligand tracer and TNF receptors. J Neuroimmunol. 2001; 114:48–56. PMID: 11240015.


13. Chen M, Wang J. Initiator caspases in apoptosis signaling pathways. Apoptosis. 2002; 7:313–319. PMID: 12101390.
14. Joseph EK, Levine JD. Caspase signalling in neuropathic and inflammatory pain in the rat. Eur J Neurosci. 2004; 20:2896–2902. PMID: 15579143.


15. Scholz J, Broom DC, Youn DH, Mills CD, Kohno T, Suter MR, et al. Blocking caspase activity prevents transsynaptic neuronal apoptosis and the loss of inhibition in lamina II of the dorsal horn after peripheral nerve injury. J Neurosci. 2005; 25:7317–7323. PMID: 16093381.


16. Yu YM, Kim JB, Lee KW, Kim SY, Han PL, Lee JK. Inhibition of the cerebral ischemic injury by ethyl pyruvate with a wide therapeutic window. Stroke. 2005; 36:2238–2243. PMID: 16141417.


17. Wang Q, Ding Q, Zhou Y, Gou X, Hou L, Chen S, et al. Ethyl pyruvate attenuates spinal cord ischemic injury with a wide therapeutic window through inhibiting high-mobility group box 1 release in rabbits. Anesthesiology. 2009; 110:1279–1286. PMID: 19417608.


18. Genovese T, Esposito E, Mazzon E, Di Paola R, Meli R, Caminiti R, et al. Beneficial effects of ethyl pyruvate in a mouse model of spinal cord injury. Shock. 2009; 32:217–227. PMID: 18948848.


19. Cho IH, Kim SW, Kim JB, Kim TK, Lee KW, Han PL, et al. Ethyl pyruvate attenuates kainic acid-induced neuronal cell death in the mouse hippocampus. J Neurosci Res. 2006; 84:1505–1511. PMID: 16958132.


20. Shang GH, Lin DJ, Xiao W, Jia CQ, Li Y, Wang AH, et al. Ethyl pyruvate reduces mortality in an endotoxin-induced severe acute lung injury mouse model. Respir Res. 2009; 10:91. PMID: 19799777.


21. Guo J, Zhang K, Ji Y, Jiang X, Zuo S. Effects of ethyl pyruvate on myocardial apoptosis and expression of Bcl-2 and Bax proteins after ischemia-reperfusion in rats. J Huazhong Univ Sci Technolog Med Sci. 2008; 28:281–283. PMID: 18563323.


22. Tsung A, Kaizu T, Nakao A, Shao L, Bucher B, Fink MP, et al. Ethyl pyruvate ameliorates liver ischemia-reperfusion injury by decreasing hepatic necrosis and apoptosis. Transplantation. 2005; 79:196–204. PMID: 15665768.


23. Li Y, Dorsi MJ, Meyer RA, Belzberg AJ. Mechanical hyperalgesia after an L5 spinal nerve lesion in the rat is not dependent on input from injured nerve fibers. Pain. 2000; 85:493–502. PMID: 10781924.


24. Suzuki R, Dickenson AH. Neuropathic pain: nerves bursting with excitement. Neuroreport. 2000; 11:R17–R21. PMID: 10976929.
25. Woolf CJ, Salter MW. Neuronal plasticity: increasing the gain in pain. Science. 2000; 288:1765–1769. PMID: 10846153.


26. Sekiguchi M, Sekiguchi Y, Konno S, Kobayashi H, Homma Y, Kikuchi S. Comparison of neuropathic pain and neuronal apoptosis following nerve root or spinal nerve compression. Eur Spine J. 2009; 18:1978–1985. PMID: 19543754.


27. Schaeffer V, Meyer L, Patte-Mensah C, Eckert A, Mensah-Nyagan AG. Sciatic nerve injury induces apoptosis of dorsal root ganglion satellite glial cells and selectively modifies neurosteroidogenesis in sensory neurons. Glia. 2010; 58:169–180. PMID: 19565659.


28. Empl M, Renaud S, Erne B, Fuhr P, Straube A, Schaeren-Wiemers N, et al. TNF-alpha expression in painful and nonpainful neuropathies. Neurology. 2001; 56:1371–1377. PMID: 11376190.


29. Kao KK, Fink MP. The biochemical basis for the anti-inflammatory and cytoprotective actions of ethyl pyruvate and related compounds. Biochem Pharmacol. 2010; 80:151–159. PMID: 20230800.


30. Ulloa L, Ochani M, Yang H, Tanovic M, Halperin D, Yang R, et al. Ethyl pyruvate prevents lethality in mice with established lethal sepsis and systemic inflammation. Proc Natl Acad Sci U S A. 2002; 99:12351–12356. PMID: 12209006.


31. Campana WM, Myers RR. Exogenous erythropoietin protects against dorsal root ganglion apoptosis and pain following peripheral nerve injury. Eur J Neurosci. 2003; 18:1497–1506. PMID: 14511329.


32. Sekiguchi M, Kobayashi H, Sekiguchi Y, Konno S, Kikuchi S. Sympathectomy reduces mechanical allodynia, tumor necrosis factor-alpha expression, and dorsal root ganglion apoptosis following nerve root crush injury. Spine (Phila Pa 1976). 2008; 33:1163–1169. PMID: 18469688.


33. Jancálek R, Dubový P, Svízenská I, Klusáková I. Bilateral changes of TNF-alpha and IL-10 protein in the lumbar and cervical dorsal root ganglia following a unilateral chronic constriction injury of the sciatic nerve. J Neuroinflammation. 2010; 7:11. PMID: 20146792.
Fig. 1
Behavioral tests for (A) mechanical and (B) cold allodynia (n = 21 rats per group). Mechanical allodynia was tested by the application of von Frey filaments to the plantar surface of the hind paw ipsilateral to the spinal nerve ligation injury. Cold allodynia was tested by the application of acetone to the same site. POD, postoperative day. *P < 0.001 compared with the control group.
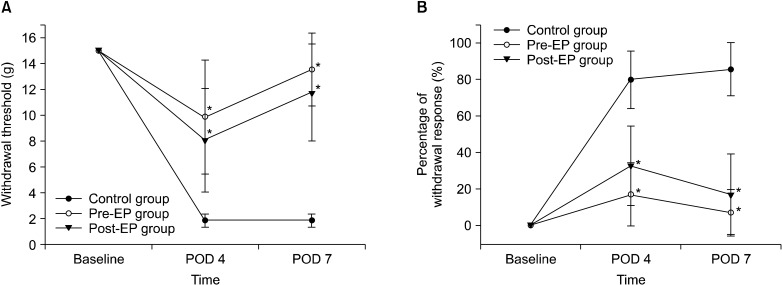
Fig. 2
TNF-α expression levels in the dorsal root ganglion (DRG). The TNF-α protein level in the ipsilateral (left) DRGs of the L5/6 spinal segments was measured using ELISA. *P < 0.001 compared with the control group.
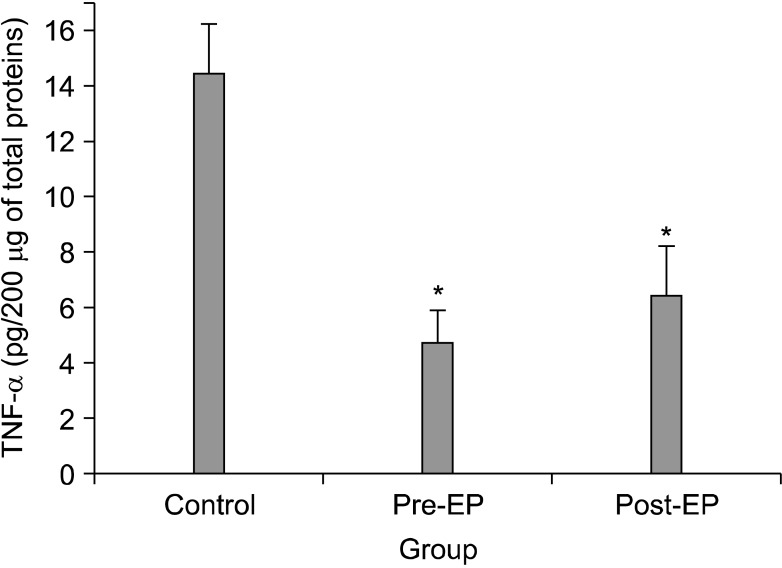
Fig. 3
Activated capsase-3 fluorescence in the dorsal root ganglion (DRG) of the control group. (A) NeuN (green) is a marker of neurons. (D) GFAP (green) is a marker of activated satellite cells. (B, E) Activated caspase-3 (red) is an indicator of apoptosis and was observed by fluorescent staining. Activated caspase-3 was co-localized in (C) DRG neurons and (F) satellite cells. The black arrows indicate where activated caspase-3 is co-localized with NeuN, and the white arrows indicate where activated caspase-3 is colocalized with GFAP. Bar indicates 50 µm. POD, postoperative day.
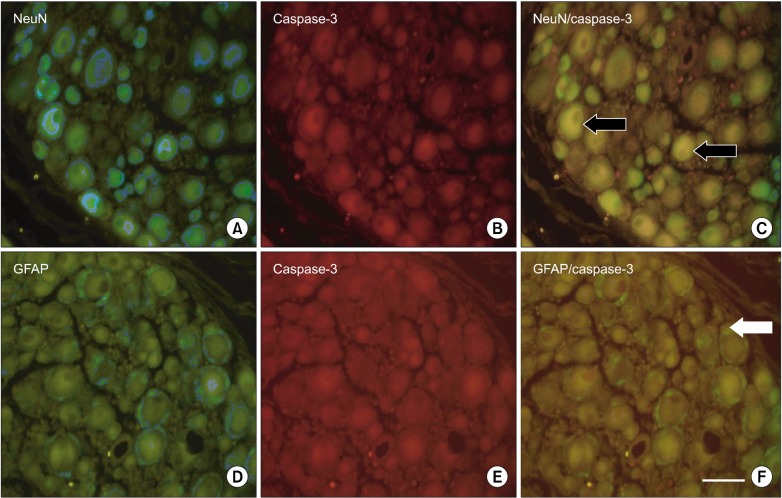
Fig. 4
Percentage of neurons and satellite cells that colocalized with caspase-3 in the dorsal root ganglion (n = 7 rats per group). NeuN is a marker of neurons. GFAP is a marker of activated satellite cells. The neurons and satellite cells that co-localized with caspase-3 were counted and are expressed as a percentage of the total neurons or activated satellite cells per section. *P = 0.002 compared with the control group.
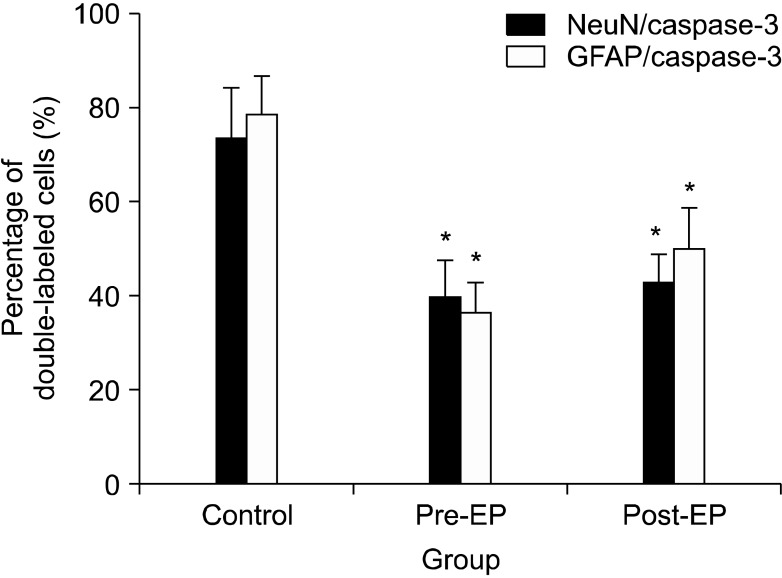