INTRODUCTION
Lumbosacral epidural injection is commonly performed in patients with lower back pain or radiating pain in the lower extremities. The long-term effect of lumbosacral epidural injection remains controversial, but many studies have shown a favorable trend regarding various effects including short-term benefits [
1-
4]. Among the several epidural approaches, caudal epidural injection (CEI) is preferred by many clinicians because it can be implemented easily. CEI is possible using a blind technique, which is less invasive and requires a short procedure time. However, the failure rate of blind techniques in adults is as high as 25%, even for experienced clinicians, because of variations in caudal anatomy [
5-
7].
Recently, ultrasound guidance has increased the success rate of CEI, with several studies showing that ultrasound is effective in guiding needle placement during CEI [
8,
9]. Ultrasound can be used to accurately assess caudal anatomical structures such as the sacral hiatus and sacral cornu before inserting the needle and to evaluate needle placement in real time [
10,
11]. Nevertheless, fluoroscopy-guided caudal epidural injection (FG-CEI) is still considered the gold standard, given the need to confirm the distribution of the solution in the epidural space [
5,
7,
12,
13]. It has been considered impossible to confirm the distribution of the injected solution at the lumbosacral level when using ultrasound-guided CEI (USG-CEI), although it is effective in guiding needle placement.
Compared to FG-CEI, USG-CEI has advantages such as ease of use, short procedural time, and contrast-free and radiation-free application. In rare cases, however, USG-CEI may result in the injected solution not reaching the desired target level, which is usually the lumbosacral epidural space. When performing a CEI with ultrasound alone, it is difficult to predict the area over which the injected solution has spread. Therefore, CEI—which is commonly recommended in clinical practice—is performed as follows. First, the sacral area is scanned using ultrasound, the needle entry point is checked, and the needle is inserted. After needle placement, fluoroscopy is used to examine the distribution of the contrast medium, following which the drug solution is injected. The identification of contrast medium via fluoroscopy is considered essential for predicting whether a drug has reached the target site [
14].
The paramedian sagittal oblique view of the lumbosacral spine (LS-PSOV) is one of the ultrasonographic views introduced for neuraxial anesthesia [
15,
16]. In the LS-PSOV, one can observe the laminae of each lumbar spine, as well as the anterior and posterior complexes containing the dura mata (
Fig. 1). Therefore, we speculated that the use of color Doppler ultrasonography would allow us to confirm the distribution of the injected solution in the epidural space at the lumbosacral level without the need for fluoroscopy. In other words, we hypothesized that color Doppler flow in the LS-PSOV during ultrasound could replace identification of contrast medium via fluoroscopy. Thus, the present study aimed to evaluate the validity and reliability of CEI using color Doppler ultrasonography in the LS-PSOV by comparing the technique with fluoroscopy.
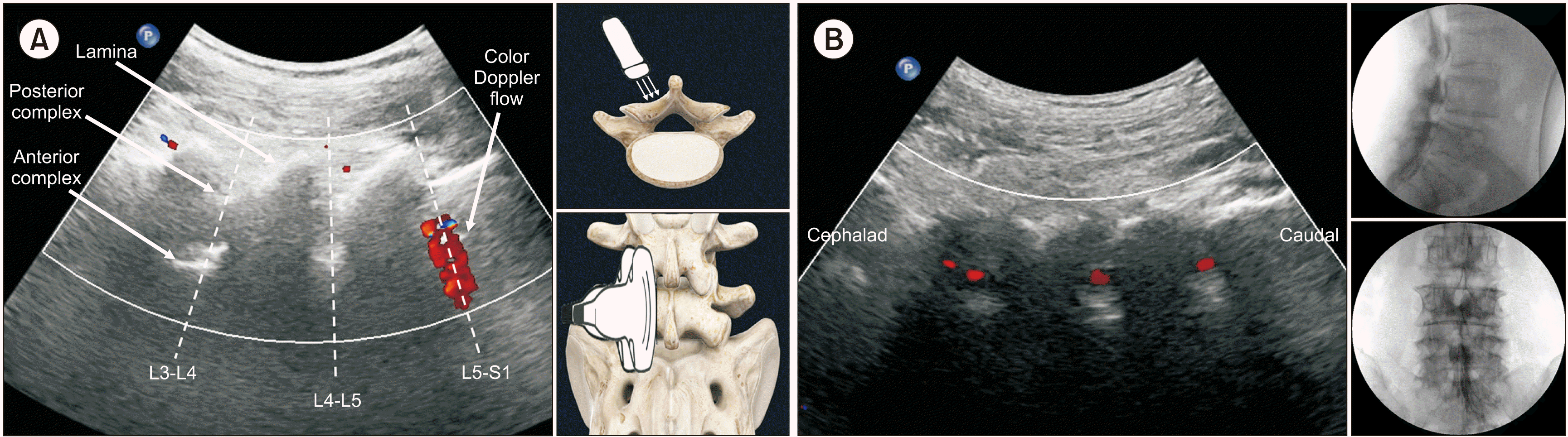 | Fig. 1Ultrasound paramedian sagittal oblique view (PSOV) of the lumbosacral level (A). The patient was in the prone position, and the convex ultrasound probe was placed on the patient’s back aligned to the sagittal plane (median sagittal view). The sacrum can be recognized as a horizontal high-echo curve structure, and the L5 layer has a typical “serrated” shape. After checking the sacrum, the sacrum is placed on the right side of the ultrasound window through the cephalad shift of the probe, and the L3 spinous process is visible on the left side. The probe was then shifted by 1-2 fingers in the left lateral direction to ensure that the lamina was visible (paramedian sagittal view). The probe was then tilted slightly toward the midline of the spine (PSOV). In the image presented here, a serrated laminar shape is visible, and hyperechoic shadows of the anterior complex and posterior complex including the dura mater can be observed between the lamina of each level. The color Doppler in (A) shows that the injection flow has reached L5-S1. (B) is a color Doppler image of another patient performed in the same way. The drug solution rises from the caudal region to the cephalad, and Doppler flow can be observed between each lamina. Doppler flow can be observed at L5-S1, L4-L5, and L3-L4, and the anteroposterior and lateral fluoroscopy images show that the contrast medium is distributed at the corresponding levels. 
|
Go to :

RESULTS
Sixty-five patients were enrolled, and all 65 patients were analyzed without exclusion. The baseline characteristics of the patients are described in
Table 1. The mean patient age was 61 years, the pre-CEI visual analogue scale (VAS) score was 6.5, and the post-CEI VAS score was 3.5 (
P < 0.001).
Table 1
Characteristic |
Patients (n = 65) |
Sex (male:female) |
32:33 |
Age (yr) |
61 (46-69) |
Height (cm) |
162.0 (154.5-170.0) |
Weight (kg) |
62 (53-70) |
Pre-CEI pain score (VAS) |
6.5 ± 2.2 |
Post-CEI pain score (VAS) |
3.5 ± 2.2 |

In 62 of 65 patients, the contrast image was observed in L5/S1 on fluoroscopy after USG-CEI, and the probability of “successful CEI” was 95.4% (
Tables 2,
3). The prediction accuracy of CEI using Doppler ultrasonography in the LS-PSOV, the primary outcome of this study, was 96.9%. That is, in all 60 patients with Doppler flow observed in the LS-PSOV, contrast images were also observed at L5-S1 on fluoroscopy, and in all three patients without Doppler flow, no contrast images were observed. Two of 65 patients exhibited no Doppler flow, although contrast images were observed at L5-S1 on fluoroscopy; consequently, the prediction of color Doppler ultrasonography was incorrect. On the other hand, none of the patients showed both positive Doppler flow and negative contrast image. The sensitivity, specificity, positive predictive value, and negative predictive value of color Doppler ultrasonography in the LS-PSOV were 96.7%, 100%, 100%, and 60.0%, respectively. In addition,
Fig. 3 shows how the level of injected drug distribution predicted by color Doppler ultrasonography match that confirmed by fluoroscopy.
Table 2
The contrast image in fluoroscopy at L5-S1 after CEI using color Doppler ultrasound in LS-PSOV
Doppler flow of ultrasonography in the LS-PSOV |
The contrast image of fluoroscopy at L5-S1 |
Positive |
Negative |
Positive |
60 |
0 |
Negative |
2 |
3 |

Table 3
Prediction accuracy of USG-CEI vs. FG-CEI (n = 65)
Result |
USG-CEI |
FG-CEI |
Prediction accuracy |
63/65 (96.9%) |
65/65 (100%) |
Absolute difference vs. FG-CEI (95% CI) |
−3.1% (−7.4% to 1.2%) |
|

In the non-inferiority test, CEI performed under Doppler ultrasonography in the LS-PSOV was not inferior to that performed under FG, since the lower limit of the confidence interval for the absolute difference versus FG-CEI was greater than the pre-specified non-inferiority margin of −10%. Among the 65 patients, there were no unexpected adverse reactions or unintended injections (e.g., intrathecal or intravascular).
Go to :

DISCUSSION
Fluoroscopy is currently the standard for predicting drug distribution in the epidural space before drug injection. Although ultrasound has many advantages over fluoroscopy and is widely used, the visualization of solution spread has only been possible using fluoroscopy [
19]. In the present study, we investigated whether ultrasound could confirm drug spread during CEI. Ultrasound has many advantages over fluoroscopy for CEI: it is radiation-free, contrast-free, and easy to use and requires a short procedure time [
9]. Above all, USG-CEI provides a more accurate route for needle insertion than FG-CEI because it can provide information regarding anatomical structures such as the sacral hiatus, sacral cornu, and sacrococcygeal ligament in real time [
20].
Our study introduced and verified a novel ultrasonic method for predicting the range of drug spread and confirming the success of CEI, with a very high prediction accuracy of 96.9%. Among the 65 patients, failure to predict the success of CEI occurred in only two patients. The statistical significance obtained from prediction accuracy was also not inferior to that of FG-CEI. In particular, the 100% specificity is very impressive. This means that the probability of a type 1 error is almost zero. In addition, if color Doppler flow is observed at L5-S1, the probability that the actual drug solution reaches above L5-S1 is 100%. If it is observed at L4-L5, the probability that the drug solution reaches above L4-L5 is also 100%.
Failure to predict successful epidural spread of the injected solution occurred in two patients. In these two patients, injection flow was not observed on color Doppler ultrasonography, but the epidural spread of the contrast solution was observed at L5-S1 under fluoroscopy. One patient had morbid obesity (body mass index: 35.2), and the other patient exhibited severe degenerative changes. These factors are thought to have influenced color Doppler detection [
21,
22]. One of the limitations of ultrasound is that it remains difficult to check for unintended intravascular injections and dura puncture [
5]. However, injecting immediately after penetrating the sacrococcygeal ligament is associated with a very low risk of intravascular injection or dura puncture [
23]. In addition, a previous study identified that intravascular injection can be distinguished even by color Doppler ultrasound flow in the longitudinal view of the sacral hiatus [
11]. In our study, none of the 65 patients received any unintended injection (
e.g., intrathecal or intravascular).
Our findings indicated that color Doppler ultrasonography in the LS-PSOV can predict the level to which the injected solution has spread in real time and in 80% of patients. Indeed, the level was exactly consistent with the fluoroscopic confirmation in 52 of 65 patients (
Fig. 3). These findings suggest that it is possible to determine whether the injected solution has reached the target level in real time, and that this method can help determine the volume of solution injected, which may in turn prevent overuse of the injected drug. When Doppler artifacts such as noise or blood vessels are suspected, the operator can distinguish them from the actual solution flow by repeatedly injecting and stopping. As in our study, this is simplified when a single operator holds the probe and injects the solution simultaneously. For consistency, all procedures were performed in the left PSOV in the present study. However, we recommend selecting the left or right PSOV based on the desired target side.
This study has several limitations. First, we did not examine solution spread above level L2-L3 on ultrasound. Since issues at L2-L3 or higher are less likely to result in lower back pain and the distance from the CEI insertion site is far, there are relatively few cases in which CEI is indicated at L2-L3. In addition, we were unable to capture L5-S1 through L2-L3 on a single ultrasound screen, making it difficult to evaluate the spread of contrast solution in these regions at the same time. Even within one ultrasound window, as the level of the lumbar spine increased, it became more difficult to observe the color Doppler flow. In particular, the amount of color Doppler flow was quite small at L3-L4, necessitating more careful examination. A larger solution volume may have allowed for easier visualization of Doppler flow. However, we thought that high doses of lidocaine pose a risk of local anesthetic systemic toxicity because ultrasound cannot complet rule out intravascular injection [
24]. In addition, according to our pilot study and our own retrospective data, even the 10 mL dose reached the level of L4-5 or L5-S1 in most patients, and it was thought that an excessive dose could rather obscure the comparison between the ultrasound and fluoroscopy in the lower lumbar level, one of the objectives of this study.
Second, when there is vascular uptake during the epidurogram, it cannot be excluded by ultrasound alone. This is thought to be a phenomenon caused by the vein in the epidural space and is distinguished from vascular injection. In our study, several vascular uptakes were identified on fluoroscopy during the epidurogram, but there was no local anesthetic systemic toxicity, including neurological symptoms. Third, the possibility that the vertebra, which is expected to be L5 on ultrasound, is actually S1, due to lumbarization of S1, cannot be excluded. Although accurate lumbar vertebra numbering is possible through whole spine images [
25], preoperative whole spine images of all patients were not obtained uniformly.
Finally, color Doppler flow was not easily observed in patients with narrow interlaminar spaces or severe scoliosis due to advanced degenerative changes. Furthermore, the ultrasound window was obscured depending on the degree of obesity, further complicating evaluation. Further studies are required to determine whether color Doppler ultrasonography in the LS-PSOV can be used to evaluate CEI of contrast material in various patient groups.
In conclusion, our findings suggest that CEI using color Doppler ultrasonography in the LS-PSOV has good validity, reliability, and feasibility. Our findings indicated that this technique was associated with high prediction accuracy and was not inferior to FG-CEI. Moreover, our study suggests that color Doppler ultrasonography in the LS-PSOV is easy to apply in clinical practice, allowing for visual confirmation that the CEI solution has reached the target level of the lumbosacral spine without the need for fluoroscopy.
Go to :
