Abstract
Stem cells are attracting attention as a key element in future medicine, satisfying the desire to live a healthier life with the possibility that they can regenerate tissue damaged or degenerated by disease or aging. Stem cells are defined as undifferentiated cells that have the ability to replicate and differentiate themselves into various tissues cells. Stem cells, commonly encountered in clinical or preclinical stages, are largely classified into embryonic, adult, and induced pluripotent stem cells. Recently, stem cell transplantation has been frequently applied to the treatment of pain as an alternative or promising approach for the treatment of severe osteoarthritis, neuropathic pain, and intractable musculoskeletal pain which do not respond to conventional medicine. The main idea of applying stem cells to neuropathic pain is based on the ability of stem cells to release neurotrophic factors, along with providing a cellular source for replacing the injured neural cells, making them ideal candidates for modulating and possibly reversing intractable neuropathic pain. Even though various differentiation capacities of stem cells are reported, there is not enough knowledge and technique to control the differentiation into desired tissues in vivo. Even though the use of stem cells is still in the very early stages of clinical use and raises complicated ethical problems, the future of stem cells therapies is very bright with the help of accumulating evidence and technology.
One of the major achievements in the development in modern medicine is the discovery of stem cells. Stem cells are attracting attention as a key element in future medicine, satisfying the desire to live a healthier life with the possibility that they can regenerate tissue damaged or degenerated by disease or aging. Development of cell therapy and regenerative medicine using stem cells is expanding the medical industry and businesses as well as increasing the understanding of the nature of the cell itself. Stem cell medicine brings a new paradigm to modern medicine which has relied heavily on medicine or surgery.
Intravenous infusions of bone marrow cells in 1957 from a healthy donor to a leukemia patient following radiation and chemotherapy would come to be considered a kind of hematopoietic stem cell therapy [1]. Since this historical event, leukemia has been successfully treated with stem cell therapy. Today, treatment with stem cells has received increasing attention as a solution to overcome the limitations of conventional treatment and medicine for intractable diseases. Stem cell therapy has been tried for various diseases, such as Lou Gehrig’s disease, Burger’s disease, spinal cord injury, Parkinson’s disease, and intractable osteoarthritis [2–6]. Recently, stem cell therapy has been introduced in the field of treatment for chronic intractable pain syndromes. The number of clinical or preclinical reports in which stem cell therapy was applied for the treatment of chronic pain has been growing [7,8].
In this review, the emerging opportunities of stem cell therapy for pain treatment are discussed as well as the limitations of stem cell treatments, and ethical and legal issues as well.
Stem cells are defined as undifferentiated cells that have the ability to replicate and differentiate themselves into various tissues cells. Stem cells play an important role in forming organs at the stage of embryonic development and restoring organs and renewing tissue functions in fully developed adults as well. Stem cells generate their daughter cells through symmetrical or asymmetrical cell division. Symmetrical divisions are defined as the generation of undifferentiated daughter cells like the parent cell, and unsymmetrical divisions are defined as the generation of differentiated daughter cells [9,10].
Since the first successful cultivation of human embryonic stem cells in 1998 [11], interest in stem cells has been increasing. Stem cells that are often encountered in clinical or preclinical stages are largely classified into embryonic, adult, and induced pluripotent stem cells (iPSCs). Embryonic stem cells can be obtained from the inner cell mass of blastocysts, one of the embryonic stages following the morula stage which is produced by a series of cleavage divisions of a fertilized ovum. The inner mass of embryonic stem cells is capable of differentiating into the endoderm, mesoderm, and ectoderm, which means it is capable of differentiating into all organs. Adult stem cells are present in all tissues or organs of the adult body. Even though they are small in number, these cells help repair and renew tissues or organs when the tissues are damaged or degraded. Recently, it was discovered that adult stem cells could be differentiated into various cells as well. Purified hematopoietic stem cells can differentiate into the hepatocytes, or bone marrow-derived mesenchymal cell can differentiate into angioblasts [12,13].
Adult stem cells can be categorized into placenta and umbilical cord stem cells, hematopoietic stem cells, bone marrow-derived mesenchymal stem cells (MSCs), and adipose-derived MSCs (AMSCs), according to their origin. MSCs originate from the mesoderm of the embryo. Most of the adult stem cell are MSCs, the origin of which are distributed between the organs and tissues developed from the mesoderm and umbilical cord, amniotic fluid, and even peripheral blood. The iPSCs are induced from already differentiated fibroblast cells. They are reprogrammed to an embryonic-like state by transfer of nuclear contents into oocytes, or by fusion with embryonic stem cells [14].
MSCs are known to be suitable for allografts due to not having major histocompatibility molecule class 2 and having only a small amount of class 1. This means that MSCs are not immunologically privileged, and could be considered merely ‘immune evasive’ [15]. However, many citations have been made in which allogenic MSCs are not immunogenic and do not stimulate alloreactivity, and in which they escape lysis by cytotoxic T-cells and natural killer-cells [16].
The differentiation ability of stem cells can be labeled with terms such as totipotent, pluripotent, and multipotent [17]. Totipotent stem cells can differentiate into any form of cells and even build organs. Pluripotent stem cells differentiate into all necessary cells but do not form complete organs. Multipotent stem cell can differentiate into several limited forms of cells. Totipotent stem cells can be found when the fertilized ovum starts to divide. Pluripotent stem cells are generally witnessed as embryonic stem cells which can be found in the inner mass cells of blastocysts or as iPSCs. Multipotent stem cells can be found as adult stem cells in the organs of the developed body, placenta or umbilical cord, and bone marrow (Fig. 1).
The genuine properties of stem cells that are attributable to regenerating damaged or degenerative tissue are the self-reproduction or self-renewal and plasticity of differentiation. Self-renewal refers to the ability to multiply themselves through the generations of daughter cells that have the same characteristics, as undifferentiated stem cells. The plasticity of differentiation refers to the ability to differentiate into various types of cells, which is sometimes influenced by the surrounding tissue environment. Pluripotent embryonic stem cells and iPSCs are excellent in their ability to reproduce and differentiate into various tissues, while multipotent adult stem cells can have a limited ability of reproduction or differentiation compared to embryonic stem cells or iPSCs [17–19].
Embryonic stem cells have complicated ethical problems to be used for clinical applications, which includes the unethical donation of ovum and abuse of embryos as life itself. Those ethical issues would limit the research on stem cells and clinical applications. On the other hand, adult stem cells and iPSCs would be free from these ethical issues. Embryonic stem cells and iPSCs, however, are more likely to differentiate into undesired tissues such as teratoma or cancer than the adult stem cells are [17].
To be defined as MSCs, three conditions must be met: first, they must be attached to plastic tissue culture ware, second, they must be able to differentiate into osteoblasts, adipose cells, and chondrocytes in vitro, and third, a specific cell surface antigen must be expressed [20].
Stem cells can be harvested from liposuction or excised adipose tissue [21–24]. Tissue samples obtained from liposuction are digested in a buffer solution containing collagenase with intermittent shaking. The digested solution is centrifuged and separated into the extracellular matrix with the oil in the upper layer and the cell layer precipitated at the bottom. The precipitated layer is called the stromal vascular fraction (SVF), which contains adipose stem cells. The SVF includes vascular endothelial cells, muscle cells, interstitial cells, fibroblasts, and hematopoietic cells. The entire unpurified SVF is often used in experimental or clinical fields, which obscure the pure effect of AMSCs. To screen AMSCs out of the SVF, serial tissue culture passages have been done on the basis that the MSCs adhere to the plastic tissue culture dish [25]. However, it is still controversial that some fibroblasts also adhere to the plastic tissue culture ware and proliferate [26]. Therefore, some researchers have separated stem cells by flow cytometry using specific cell surface antigens.
Among the cells in the bone marrow, cells which are non-adherent to plastic culture dishes are hematopoietic stem cells, while adherent cells are MSCs like adipose stem cells, and constitute the stromal cells of bone marrow [27]. So far, there is no single marker molecule that can define MSCs, so stem cells obtained by plastic dish adhesion are still used prevalently [28,29].
Recently, stem cells transplantation has been frequently applied to the treatment of pain as an alternative or promising approach for the treatment of severe osteoarthritis, neuropathic pain and intractable musculoskeletal pain which does not respond to conventional medicine. Stem cell-based therapies have been realized to be a potential treatment option for articular cartilage repair in patients with knee osteoarthritis [6,30], neuropathic pain [31,32], and intervertebral disc disease [33].
Degeneration and inflammation of the cartilage that covers the joint surface is the main cause of pain in osteoarthritis. The cartilage of the articular surface reduces the friction of the joint motion and acts as a cushion against weight loading. While chondrocytes occupy only 1% to 5% of cartilage volume, they produce collagen, proteoglycans, and hyaluronan, which are components of the extracellular matrix, and maintain cartilage structure and physical properties [34]. However, as the cartilage has no blood vessels and nerves, cartilage regeneration is difficult once the cartilage has been damaged or undergone degenerative changes.
Some that limit the regeneration of, and recovery from, damaged cartilage. Chondrocytes within the cartilage can migrate very slowly to the adjacent lesion. Even if bone marrow-derived stem cells can help cartilage regenerate, it is only possible when the bone marrow is exposed, or, in other words, when the entire layer of cartilage and chondral bone is damaged. The evidence for the contribution of stem cells, which have migrated from the synovial membrane or fluid, for cartilage regeneration, is not definitive.
For one of the attempts to overcome the limitation of the natural regeneration of cartilage in osteoarthritis, autologous chondrocyte transplantation has been reported in 1994 [35]. The implantation of MSCs has also been reported. Repaired tissues treated with MSCs appeared to have better cell arrangement, subchondral bone remodeling, and integration with surrounding cartilage than did repaired tissues generated by chondrocyte implantation [36]. Various sources of MSCs, which have the ability to differentiate into chondrocytes and regenerate cartilage, have been reported [37,38]. While adipose tissues, the synovial membrane, and the umbilical cord would be one of the sources, bone marrow-derived MSCs have been known as the standard sources of adult stem cells for the treatment of knee osteoarthritis. They are generally harvested from the iliac crest and easily differentiate into cartilage tissues under specific conditions [39–41].
The therapeutic modalities applied for osteoarthritis include surgical intervention or arthroscopy, tissue engineering, and intra-articular injection of cultured stem cells. These modalities would be applied individually or in combination. Microfractures are made artificially under arthroscopy by awls which are used to make holes through the subchondral bone plate to become focal full-thickness cartilage defects. This procedure is intended to allow the migration of stem cells in the bone marrow to reach the cartilage defect site. In this case, the cartilage produced by this procedure tends to become less durable fibrous cartilage in comparison to the innate hyaline cartilage [42]. The scaffolds that provide mechanical support for cells and the extracellular matrix can be used for culturing stem cells. This scaffold also needs surgery through which the cultured scaffold could be implanted in the joint [43,44].
For the convenience of the clinical use of stem cells, intra-articular injection of cultured cell therapy would be a minimally invasive and potentially efficient method for knee osteoarthritis. Intra-articular-injected autologous MSCs increased the knee cartilage volume and improved the pain scores [45]. MSCs, as an intra-articular injection adjuvant to the arthroscopic debridement procedure, resulted in better outcomes than the debridement alone [46]. Both increased interleukin 6 (IL-6) and tumor necrosis factor-alpha (TNF-α) in the synovial membrane and increased TNF-α and IL-1β in the vastus lateralis were closely related with the pain and muscular atrophy of osteoarthritis patients [47]. A precursor of inflammatory cytokines in the serum or synovial fluid was associated with osteochondritis [48]. Due to MSCs having immunomodulatory functions and the characteristic of homing to injured sites, it is effective in treating trauma or inflammatory pain.
Intra-articular cell therapy for osteoarthritis with autologous culture-expanded stem cells showed only four serious adverse events in 844 procedure reviews including one case of infection on the bone marrow aspiration site and one pulmonary embolism; two tumors, not at the site of injection, were reported as unrelated. The main adverse events related to the procedure were increased pain, swelling, and dehydration after bone marrow aspiration [49]. Safety reports on 227 cases of intra-articular MSC injection showed 7 cases of probable procedure-related complications and 3 cases of possible stem cell-related complications, all of which were either self-limited or were remedied with simple therapeutic measures. There was no cancer-related adverse event reported during the two years of follow-up [50].
Many of the systemic reviews have reported that the treatment of knee osteoarthritis with intra-articular injections of stem cells showed favorable results in which the therapy can reduce knee pain and improve physical function and cartilage quality [34,51,52]. However, there have been reports which did not have optimistic or promising point of views [53,54]. Some reports emphasized that evidence of efficacy remains limited because of poor study design, a high risk of bias, large heterogeneity, and a wide confidence interval in the estimate of the effects. In addition, rehabilitation programs followed by stem cell injections played an important role in reducing pain [55]. To date, there have been no reliable and convincing clinical human studies with a high level of evidence conducted on the application of intra-articular stem cell injection to knee arthritis. Intra-articular injection therapy using stem cells suffers from a lack of evidence of efficacy in both functional improvements and cartilage repair.
Treatment of neuropathic pain is a clinical challenge, as the pathogenesis is very complicated. The pathology of neuropathic pain involves the entire nervous systems, including the peripheral nerve, dorsal root ganglion, spinal cord, and brain.
The main idea of applying stem cells to neuropathic pain is based on the ability of stem cells to release neurotrophic factors, along with providing a cellular source for replacing the injured neural cells, which make them ideal candidates for modulating and possibly reversing intractable neuropathic pain.
Hofstetter et al. [56] and Fischer et al. [57] confirmed the relief of pain and recovery of motor function by directly administering neural stem cells to a spinal cord injury model. Stem cells would migrate to the injured site, which is called the homing of stem cells [58–60]. Thanks to the homing properties of stem cells, relieving neuropathic pain can be achieved even by intravenous injection [31].
When human bone marrow-derived stem cells were directly administered to the cerebral ventricle of rats or intravenously, they were found to settle in the spinal cord or prefrontal cortex [31,61]. The stem cells given intravenously were apt to be trapped in the lung when passing through; therefore, only a small amount of the stem cells can survive and move to the injured site [57]. From such a point of view, selecting the intrathecal route and targeting the pain pathway of the dorsal root ganglion or spinal cord directly looks like the obvious method for stem cell delivery in a spinal cord injury model [62].
During the early phase in the research of stem cells, the focus has been directed toward the regeneration of tissues. Recently, the focus has been more on the side of the paracrine effect, which is known to participate in tissue repair by stimulating surrounding cells to be recruited and by suppress the inflammation responses [63]. Stem cells do not need to make direct contact with the injured cells to have a neuroprotective effect as is revealed in vitro studies [64,65].
Both the neurotrophic factors and neuroinflammatory cascades caused by immune and glial cells also play an important role in the development of neuropathic pain [31,66–69]. When the balance between both factors is destroyed, and the inflammatory side becomes dominant, neuropathic pain is more likely to occur. Significant increases in IL-1β and IL-6, but not TNF-α, in the cerebrospinal fluid of complex regional pain syndrome patients, which indicates the activation of the neuroimmune system, as compared to controls, was reported [70].
Various stem cells including human mesenchymal stem or stromal cells, are known to secrete neurotrophic factors and anti-neuroinflammatory cytokines which have neuroprotective and even regenerative effect [64,71–75]. With these paracrine effects, stem cells inhibit the hazard of the inflammatory cytokines [76]. Neurotrophic factors, especially nerve growth factor (NGF) and glial cell line-derived neurotrophic factor help the injured nerve restore itself in maintaining the function of a nerve, promoting regeneration, and regulating neural plasticity in response to injury [66]. MSCs reduce the secretion of inflammatory cytokine in T-cells such as IL-1β or TNF-α [77]. In addition to the paracrine effects, intrathecal administration of MSCs reduces the reactive oxygen species and pain behavior in neuropathic rats [78].
The pathology of diabetic peripheral neuropathy initiates from the destruction or obstruction of peripheral vessels. Consequently, decreased blood flow ends up causing nerve damage. The stem cells that secrete neurotrophic factors and paracrine inducing neovascularization should be an effective therapy for diabetic peripheral neuropathy [79–82]. In a diabetic neuropathic pain animal model, transplantation of MSCs improved the blood circulation and nerve conduction velocity. Neurotrophic factors such as NGF, neurotrophin-3 protein, vascular endothelial growth factor, and basic fibroblast growth factor are reported to be involved as attributable factors [83,84].
There have been three reports on diabetic neuropathy in an animal model. Stem cells were administered intramuscularly to the hind leg. Subjects were observed for 2 to 16 weeks and showed improvement in nerve conduction velocity through the paracrine actions of growth factors secreted by MSCs [80,83,84]. MSCs, differentiated into anti-inflammatory cells, attenuated pain behaviors of streptozotocin-induced diabetes in a rat model [85,86]. A report said that patients with type I diabetes who received MSCs did not need analgesics after the dramatic pain reduction at two months, blood flow was recovered after six months, painlessness after nine months, and all tissues with infection and necrosis were recovered [87].
Patients with spinal cord injury suffer from desperate and intractable pain. Reduced neurotrophic factors caused by disrupted inhibitory pathways and the production of proinflammatory cytokines would be attributable to neuropathic pain [88–90].
In an animal model of spinal cord injury, stem cell therapy reduced pain by differentiating into glial cells and releasing trophic factors. That is, stem cells contribute pain medicine as small analgesic biopumps in addition to supplying cellular sources of tissue regeneration. When the neural stem cells were injected intrathecally into the spinal cord injury rat model, they would have an analgesic effect as small biopumps releasing inhibitory neurotransmitters, such as gamma-aminobutyric acid or glycine [91]. Other animal studies reported that the transplantation of MSCs for the treatment of spinal cord injury produced gait improvement and evidence of histological regeneration of the nerve [92,93]. In a meta-analysis of an animal model [94], the efficacy of neural stem/progenitor cell transplantation was higher in transection and contusion models than in compression ones. The shorter the interval between injury and treatment, the better the functional recovery and sensory condition. Immunosuppressive drugs used for avoiding rejection negatively affected motor function recovery. Scaffold use could boost efficacy on motor function recovery. However, other reports said that the neural stem cells rather increase the pain of spinal cord injury. Neural stem cells survived and differentiated into a predominately astrocytic population; however, the locomotor function was not improved and significant forelimb thermal and mechanical allodynia were observed [95].
A clinical case of a patient with an incomplete spinal cord injury at the T12-L1 level and a crush fracture in the L1 vertebral body was administered several doses of allogeneic MSCs intrathecally and intravenously. The patient reported a marked decrease of neuropathic pain, an improvement in muscle strength, an increased dermatomal sensation, and a recovery of urological and sexual functions [5].
Intravenous administration of bone marrow-derived mononuclear cells reduced neuropathic pain in a sciatic nerve CCI model [96]. Systemic administration of human MSCs attenuated the neuropathic pain in a spared nerve injury mouse model. The human MSCs were mainly able to home in on the spinal cord and pre-frontal cortex of neuropathic mice. It reduced the level of IL-1β and IL-17 and increased the anti-inflammatory cytokine IL-10 and the activity of macrophages [31].
Seven studies involving a sciatic nerve injury model were reviewed. In 4 of them, stem cells were given intravenously. For the other 3, stem cells were placed directly on the sciatic nerve, the L4 dorsal root ganglion, and the lateral ventricle of the cerebrum, respectively. Administration to the lateral ventricle was intended to allow observation of the influence of supraspinal regulation of neuropathic pain. Observations were made for 1 to 90 days after stem cell implantation; reports said that most of the allodynia and hyperalgesia had decreased [31,61,97–101].
The expression of TNF-α and IL-8 in the nucleus pulposus of degenerated discs was much higher than that of herniated discs. That would be a reason why the level of pain is more severe in patients with a degenerated disc [102]. When patients with degenerative disc disease were treated with autologous expanded bone marrow MSCs injected into the nucleus pulposus, the pain and disability were improved, and were comparable to spinal fusion surgery, although disc height was not recovered [103].
A systemic review of autologous MSC injections for the regeneration of intervertebral discs was conducted on a total of 98 patients and 122 treated levels in seven studies [33]. Bone marrow-derived MSCs harvested from the iliac crest represented the most common type of injected cell. Patients with fractures of the trabecular bone and intervertebral discs with a complete radial fissure were excluded because the non-integrity of the annulus may allow the injected stem cells to escape. Patients with low back pain due to initial intervertebral disc degeneration and low-stage radiological degeneration were eligible for the stem cell infiltration. The average Oswestry Disability Index and visual analogue scale scores improved at the one-year follow-up. Quantitative improvements, such as T2-weighted magnetic resonance image scans, protrusion sizes, and disc bulges also improved.
Adequate dosages of stem cells have not been well established. For clinical applications, however, more research on stem cell types, doses, safety, and implantation rates is needed. When the neuronal or adipose stem cells are given in a mouse CCI model repetitively, they have an analgesic effect in a dose-dependent way [104].
In high concentration of human MSCs, dopaminergic neurons in a rodent Parkinson’s disease model were well preserved, and neurogenesis was enhanced [71]. In a study with a Parkinson’s disease animal model, both mechanisms of human MSCs, mediated by neurotrophic paracrine effects and differentiation to neuronal cells, may work in the neuroprotective process even though only 1.7% of injected human MSCs survive [74].
To compare and discuss the effect of a lot of clinical studies, it is important to develop internationally standardized methods for MSC production. To be accepted as a standard treatment, stem cell therapy should be evidence-based, legally compliant, and cost-effective treatment and should have excellent clinical outcomes [105].
Even though various differentiation capacities of stem cells are reported, there is not enough knowledge nor sufficient technique to control the differentiation into desired tissues in vivo [106–108]. However, differentiation techniques to targeted cells by biological factors or physical stimuli are being developed along with the discovery of stem cell populations and the advancement of culture technology. Even though stem cells are still in the very early stages of clinical use, the future of stem cells is very bright with the help of accumulating evidence and technologies.
Notes
REFERENCES
1. Thomas ED, Lochte HL Jr, Lu WC, Ferrebee JW. Intravenous infusion of bone marrow in patients receiving radiation and chemotherapy. N Engl J Med. 1957; 257:491–6. DOI: 10.1056/NEJM195709122571102. PMID: 13464965.


2. Ciervo Y, Ning K, Jun X, Shaw PJ, Mead RJ. Advances, challenges and future directions for stem cell therapy in amyotrophic lateral sclerosis. Mol Neurodegener. 2017; 12:85. DOI: 10.1186/s13024-017-0227-3. PMID: 29132389. PMCID: PMC5683324.


3. Cacione DG, do Carmo Novaes F, Moreno DH. Stem cell therapy for treatment of thromboangiitis obliterans (Buerger’s disease). Cochrane Database Syst Rev. 2018; 10:CD012794.


4. Venkatesh K, Sen D. Mesenchymal stem cells as a source of dopaminergic neurons: a potential cell based therapy for parkinson’s disease. Curr Stem Cell Res Ther. 2017; 12:326–47. DOI: 10.2174/1574888X12666161114122059. PMID: 27842480.


5. Ichim TE, Solano F, Lara F, Paris E, Ugalde F, Rodriguez JP, et al. Feasibility of combination allogeneic stem cell therapy for spinal cord injury: a case report. Int Arch Med. 2010; 3:30. DOI: 10.1186/1755-7682-3-30. PMID: 21070647. PMCID: PMC2989319.


6. Pers YM, Ruiz M, Noël D, Jorgensen C. Mesenchymal stem cells for the management of inflammation in osteoarthritis: state of the art and perspectives. Osteoarthritis Cartilage. 2015; 23:2027–35. DOI: 10.1016/j.joca.2015.07.004. PMID: 26521749.


7. Chakravarthy K, Chen Y, He C, Christo PJ. Stem cell therapy for chronic pain management: review of uses, advances, and adverse effects. Pain Physician. 2017; 20:293–305. PMID: 28535552.
8. Hosseini M, Yousefifard M, Aziznejad H, Nasirinezhad F. The effect of bone marrow-derived mesenchymal stem cell transplantation on allodynia and hyperalgesia in neuropathic animals: a systematic review with meta-analysis. Biol Blood Marrow Transplant. 2015; 21:1537–44. DOI: 10.1016/j.bbmt.2015.05.008. PMID: 25985918.


9. Morrison SJ, Shah NM, Anderson DJ. Regulatory mechanisms in stem cell biology. Cell. 1997; 88:287–98. DOI: 10.1016/S0092-8674(00)81867-X. PMID: 9039255.


10. Blank U, Karlsson G, Karlsson S. Signaling pathways governing stem-cell fate. Blood. 2008; 111:492–503. DOI: 10.1182/blood-2007-07-075168. PMID: 17914027.


11. Thomson JA, Itskovitz-Eldor J, Shapiro SS, Waknitz MA, Swiergiel JJ, Marshall VS, et al. Embryonic stem cell lines derived from human blastocysts. Science. 1998; 282:1145–7. DOI: 10.1126/science.282.5391.1145. PMID: 9804556.


12. Lagasse E, Connors H, Al-Dhalimy M, Reitsma M, Dohse M, Osborne L, et al. Purified hematopoietic stem cells can differentiate into hepatocytes in vivo. Nat Med. 2000; 6:1229–34. DOI: 10.1038/81326. PMID: 11062533.


13. Kocher AA, Schuster MD, Szabolcs MJ, Takuma S, Burkhoff D, Wang J, et al. Neovascularization of ischemic myocardium by human bone-marrow-derived angioblasts prevents cardiomyocyte apoptosis, reduces remodeling and improves cardiac function. Nat Med. 2001; 7:430–6. DOI: 10.1038/86498. PMID: 11283669.


14. Takahashi K, Yamanaka S. Induction of pluripotent stem cells from mouse embryonic and adult fibroblast cultures by defined factors. Cell. 2006; 126:663–76. DOI: 10.1016/j.cell.2006.07.024. PMID: 16904174.


15. Ankrum JA, Ong JF, Karp JM. Mesenchymal stem cells: immune evasive, not immune privileged. Nat Biotechnol. 2014; 32:252–60. DOI: 10.1038/nbt.2816. PMID: 24561556. PMCID: PMC4320647.


16. Le Blanc K. Immunomodulatory effects of fetal and adult mesenchymal stem cells. Cytotherapy. 2003; 5:485–9. DOI: 10.1080/14653240310003611. PMID: 14660044.


17. Zakrzewski W, Dobrzyński M, Szymonowicz M, Rybak Z. Stem cells: past, present, and future. Stem Cell Res Ther. 2019; 10:68. DOI: 10.1186/s13287-019-1165-5. PMID: 30808416. PMCID: PMC6390367.


18. Chagastelles PC, Nardi NB. Biology of stem cells: an overview. Kidney Int Suppl (2011). 2011; 1:63–7. DOI: 10.1038/kisup.2011.15. PMID: 25028627. PMCID: PMC4089750.


19. Oh IH, Kim DW. Three-dimensional approach to stem cell therapy. J Korean Med Sci. 2002; 17:151–60. DOI: 10.3346/jkms.2002.17.2.151. PMID: 11961296. PMCID: PMC3054865.


20. Dominici M, Le Blanc K, Mueller I, Slaper-Cortenbach I, Marini F, Krause D, et al. Minimal criteria for defining multipotent mesenchymal stromal cells. The International Society for Cellular Therapy position statement. Cytotherapy. 2006; 8:315–7. DOI: 10.1080/14653240600855905. PMID: 16923606.


21. Zuk PA, Zhu M, Mizuno H, Huang J, Futrell JW, Katz AJ, et al. Multilineage cells from human adipose tissue: implications for cell-based therapies. Tissue Eng. 2001; 7:211–28. DOI: 10.1089/107632701300062859. PMID: 11304456.


22. Gronthos S, Franklin DM, Leddy HA, Robey PG, Storms RW, Gimble JM. Surface protein characterization of human adipose tissue-derived stromal cells. J Cell Physiol. 2001; 189:54–63. DOI: 10.1002/jcp.1138. PMID: 11573204.


23. Aust L, Devlin B, Foster SJ, Halvorsen YD, Hicok K, du Laney T, et al. Yield of human adipose-derived adult stem cells from liposuction aspirates. Cytotherapy. 2004; 6:7–14. DOI: 10.1080/14653240310004539. PMID: 14985162.


24. Boquest AC, Shahdadfar A, Brinchmann JE, Collas P. Isolation of stromal stem cells from human adipose tissue. Methods Mol Biol. 2006; 325:35–46. PMID: 16761717.


25. Locke M, Windsor J, Dunbar PR. Human adipose-derived stem cells: isolation, characterization and applications in surgery. ANZ J Surg. 2009; 79:235–44. DOI: 10.1111/j.1445-2197.2009.04852.x. PMID: 19432707.


26. Safford KM, Rice HE. Stem cell therapy for neurologic disorders: therapeutic potential of adipose-derived stem cells. Curr Drug Targets. 2005; 6:57–62. DOI: 10.2174/1389450053345028. PMID: 15720213.


27. Prockop DJ. Marrow stromal cells as stem cells for nonhematopoietic tissues. Science. 1997; 276:71–4. DOI: 10.1126/science.276.5309.71. PMID: 9082988.


28. Majumdar MK, Banks V, Peluso DP, Morris EA. Isolation, characterization, and chondrogenic potential of human bone marrow-derived multipotential stromal cells. J Cell Physiol. 2000; 185:98–106. DOI: 10.1002/1097-4652(200010)185:1<98::AID-JCP9>3.0.CO;2-1. PMID: 10942523.


29. Johnstone B, Hering TM, Caplan AI, Goldberg VM, Yoo JU. In vitro chondrogenesis of bone marrow-derived mesenchymal progenitor cells. Exp Cell Res. 1998; 238:265–72. DOI: 10.1006/excr.1997.3858. PMID: 9457080.
30. Pers YM, Rackwitz L, Ferreira R, Pullig O, Delfour C, Barry F, et al. Adipose mesenchymal stromal cell-based therapy for severe osteoarthritis of the knee: a phase i dose-escalation trial. Stem Cells Transl Med. 2016; 5:847–56. DOI: 10.5966/sctm.2015-0245. PMID: 27217345. PMCID: PMC4922848.


31. Siniscalco D, Giordano C, Galderisi U, Luongo L, de Novellis V, Rossi F, et al. Long-lasting effects of human mesenchymal stem cell systemic administration on pain-like behaviors, cellular, and biomolecular modifications in neuropathic mice. Front Integr Neurosci. 2011; 5:79. DOI: 10.3389/fnint.2011.00079. PMID: 22164136. PMCID: PMC3230031.


32. Richardson SM, Kalamegam G, Pushparaj PN, Matta C, Memic A, Khademhosseini A, et al. Mesenchymal stem cells in regenerative medicine: focus on articular cartilage and intervertebral disc regeneration. Methods. 2016; 99:69–80. DOI: 10.1016/j.ymeth.2015.09.015. PMID: 26384579.


33. Migliorini F, Rath B, Tingart M, Baroncini A, Quack V, Eschweiler J. Autogenic mesenchymal stem cells for intervertebral disc regeneration. Int Orthop. 2019; 43:1027–36. DOI: 10.1007/s00264-018-4218-y. PMID: 30415465.


34. Li MH, Xiao R, Li JB, Zhu Q. Regenerative approaches for cartilage repair in the treatment of osteoarthritis. Osteoarthritis Cartilage. 2017; 25:1577–87. DOI: 10.1016/j.joca.2017.07.004. PMID: 28705606.


35. Brittberg M, Lindahl A, Nilsson A, Ohlsson C, Isaksson O, Peterson L. Treatment of deep cartilage defects in the knee with autologous chondrocyte transplantation. N Engl J Med. 1994; 331:889–95. DOI: 10.1056/NEJM199410063311401. PMID: 8078550.


36. Yan H, Yu C. Repair of full-thickness cartilage defects with cells of different origin in a rabbit model. Arthroscopy. 2007; 23:178–87. DOI: 10.1016/j.arthro.2006.09.005. PMID: 17276226.


37. Goessler UR, Bugert P, Bieback K, Stern-Straeter J, Bran G, Hörmann K, et al. Integrin expression in stem cells from bone marrow and adipose tissue during chondrogenic differentiation. Int J Mol Med. 2008; 21:271–9. PMID: 18288373.


38. Kern S, Eichler H, Stoeve J, Klüter H, Bieback K. Comparative analysis of mesenchymal stem cells from bone marrow, umbilical cord blood, or adipose tissue. Stem Cells. 2006; 24:1294–301. DOI: 10.1634/stemcells.2005-0342. PMID: 16410387.


39. Wakitani S, Imoto K, Yamamoto T, Saito M, Murata N, Yoneda M. Human autologous culture expanded bone marrow mesenchymal cell transplantation for repair of cartilage defects in osteoarthritic knees. Osteoarthritis Cartilage. 2002; 10:199–206. DOI: 10.1053/joca.2001.0504. PMID: 11869080.


40. Wakitani S, Mitsuoka T, Nakamura N, Toritsuka Y, Nakamura Y, Horibe S. Autologous bone marrow stromal cell transplantation for repair of full-thickness articular cartilage defects in human patellae: two case reports. Cell Transplant. 2004; 13:595–600. DOI: 10.3727/000000004783983747. PMID: 15565871.


41. Wakitani S, Nawata M, Tensho K, Okabe T, Machida H, Ohgushi H. Repair of articular cartilage defects in the patellofemoral joint with autologous bone marrow mesenchymal cell transplantation: three case reports involving nine defects in five knees. J Tissue Eng Regen Med. 2007; 1:74–9. DOI: 10.1002/term.8. PMID: 18038395.


42. Erggelet C, Vavken P. Microfracture for the treatment of cartilage defects in the knee joint - a golden standard? J Clin Orthop Trauma. 2016; 7:145–52. DOI: 10.1016/j.jcot.2016.06.015. PMID: 27489408. PMCID: PMC4949407.


43. Kramer J, Böhrnsen F, Lindner U, Behrens P, Schlenke P, Rohwedel J. In vivo matrix-guided human mesenchymal stem cells. Cell Mol Life Sci. 2006; 63:616–26. DOI: 10.1007/s00018-005-5527-z. PMID: 16482398.


44. Tseng WJ, Huang SW, Fang CH, Hsu LT, Chen CY, Shen HH, et al. Treatment of osteoarthritis with collagen-based scaffold: a porcine animal model with xenograft mesenchymal stem cells. Histol Histopathol. 2018; 33:1271–86. PMID: 29905361.
45. Centeno CJ, Busse D, Kisiday J, Keohan C, Freeman M, Karli D. Increased knee cartilage volume in degenerative joint disease using percutaneously implanted, autologous mesenchymal stem cells. Pain Physician. 2008; 11:343–53. PMID: 18523506.
46. Varma HS, Dadarya B, Vidyarthi A. The new avenues in the management of osteo-arthritis of knee--stem cells. J Indian Med Assoc. 2010; 108:583–5. PMID: 21510531.
47. Levinger I, Levinger P, Trenerry MK, Feller JA, Bartlett JR, Bergman N, et al. Increased inflammatory cytokine expression in the vastus lateralis of patients with knee osteoarthritis. Arthritis Rheum. 2011; 63:1343–8. DOI: 10.1002/art.30287. PMID: 21538317.


48. Orita S, Koshi T, Mitsuka T, Miyagi M, Inoue G, Arai G, et al. Associations between proinflammatory cytokines in the synovial fluid and radiographic grading and pain-related scores in 47 consecutive patients with osteoarthritis of the knee. BMC Musculoskelet Disord. 2011; 12:144. DOI: 10.1186/1471-2474-12-144. PMID: 21714933. PMCID: PMC3144455.


49. Peeters CM, Leijs MJ, Reijman M, van Osch GJ, Bos PK. Safety of intra-articular cell-therapy with culture-expanded stem cells in humans: a systematic literature review. Osteoarthritis Cartilage. 2013; 21:1465–73. DOI: 10.1016/j.joca.2013.06.025. PMID: 23831631.


50. Centeno CJ, Schultz JR, Cheever M, Robinson B, Freeman M, Marasco W. Safety and complications reporting on the re-implantation of culture-expanded mesenchymal stem cells using autologous platelet lysate technique. Curr Stem Cell Res Ther. 2010; 5:81–93. DOI: 10.2174/157488810790442796. PMID: 19951252.


51. Kim SH, Ha CW, Park YB, Nam E, Lee JE, Lee HJ. Intraarticular injection of mesenchymal stem cells for clinical outcomes and cartilage repair in osteoarthritis of the knee: a meta-analysis of randomized controlled trials. Arch Orthop Trauma Surg. 2019; 139:971–80. DOI: 10.1007/s00402-019-03140-8. PMID: 30756165.


52. Ha CW, Park YB, Kim SH, Lee HJ. Intra-articular mesenchymal stem cells in osteoarthritis of the knee: a systematic review of clinical outcomes and evidence of cartilage repair. Arthroscopy. 2019; 35:277–88.e2. DOI: 10.1016/j.arthro.2018.07.028. PMID: 30455086.


53. Rodriguez-Merchan EC. Intra-articular injections of fatderived mesenchymal stem cells in knee osteoarthritis: are they recommended? Hosp Pract (1995). 2018; 46:172–4. DOI: 10.1080/21548331.2018.1505181. PMID: 30052101.


54. Pas HI, Winters M, Haisma HJ, Koenis MJ, Tol JL, Moen MH. Stem cell injections in knee osteoarthritis: a systematic review of the literature. Br J Sports Med. 2017; 51:1125–33. DOI: 10.1136/bjsports-2016-096793. PMID: 28258177.


55. Iijima H, Isho T, Kuroki H, Takahashi M, Aoyama T. Effectiveness of mesenchymal stem cells for treating patients with knee osteoarthritis: a meta-analysis toward the establishment of effective regenerative rehabilitation. NPJ Regen Med. 2018; 3:15. DOI: 10.1038/s41536-018-0041-8. PMID: 30245848. PMCID: PMC6141619.


56. Hofstetter CP, Holmström NA, Lilja JA, Schweinhardt P, Hao J, Spenger C, et al. Allodynia limits the usefulness of intraspinal neural stem cell grafts; directed differentiation improves outcome. Nat Neurosci. 2005; 8:346–53. DOI: 10.1038/nn1405. PMID: 15711542.


57. Fischer UM, Harting MT, Jimenez F, Monzon-Posadas WO, Xue H, Savitz SI, et al. Pulmonary passage is a major obstacle for intravenous stem cell delivery: the pulmonary firstpass effect. Stem Cells Dev. 2009; 18:683–92. DOI: 10.1089/scd.2008.0253. PMID: 19099374. PMCID: PMC3190292.


58. Lu D, Mahmood A, Wang L, Li Y, Lu M, Chopp M. Adult bone marrow stromal cells administered intravenously to rats after traumatic brain injury migrate into brain and improve neurological outcome. Neuroreport. 2001; 12:559–63. DOI: 10.1097/00001756-200103050-00025. PMID: 11234763.


59. Zhang J, Shi XQ, Echeverry S, Mogil JS, De Koninck Y, Rivest S. Expression of CCR2 in both resident and bone marrow-derived microglia plays a critical role in neuropathic pain. J Neurosci. 2007; 27:12396–406. DOI: 10.1523/JNEUROSCI.3016-07.2007. PMID: 17989304. PMCID: PMC6673247.


60. Mahmood A, Lu D, Chopp M. Intravenous administration of marrow stromal cells (MSCs) increases the expression of growth factors in rat brain after traumatic brain injury. J Neurotrauma. 2004; 21:33–9. DOI: 10.1089/089771504772695922. PMID: 14987463.


61. Siniscalco D, Giordano C, Galderisi U, Luongo L, Alessio N, Di Bernardo G, et al. Intra-brain microinjection of human mesenchymal stem cells decreases allodynia in neuropathic mice. Cell Mol Life Sci. 2010; 67:655–69. DOI: 10.1007/s00018-009-0202-4. PMID: 19937263.


62. Chen G, Park CK, Xie RG, Ji RR. Intrathecal bone marrow stromal cells inhibit neuropathic pain via TGF-β secretion. J Clin Invest. 2015; 125:3226–40. DOI: 10.1172/JCI80883. PMID: 26168219. PMCID: PMC4563753.


63. Mariani E, Facchini A. Clinical applications and biosafety of human adult mesenchymal stem cells. Curr Pharm Des. 2012; 18:1821–45. DOI: 10.2174/138161212799859666. PMID: 22352750.


64. Nesti C, Pardini C, Barachini S, D’Alessandro D, Siciliano G, Murri L, et al. Human dental pulp stem cells protect mouse dopaminergic neurons against MPP+ or rotenone. Brain Res. 2011; 1367:94–102. DOI: 10.1016/j.brainres.2010.09.042. PMID: 20854799.


65. Sarnowska A, Braun H, Sauerzweig S, Reymann KG. The neuroprotective effect of bone marrow stem cells is not dependent on direct cell contact with hypoxic injured tissue. Exp Neurol. 2009; 215:317–27. DOI: 10.1016/j.expneurol.2008.10.023. PMID: 19063882.


66. Ossipov MH. Growth factors and neuropathic pain. Curr Pain Headache Rep. 2011; 15:185–92. DOI: 10.1007/s11916-011-0183-5. PMID: 21327569.


67. Scholz J, Woolf CJ. The neuropathic pain triad: neurons, immune cells and glia. Nat Neurosci. 2007; 10:1361–8. DOI: 10.1038/nn1992. PMID: 17965656.


68. Martucci C, Trovato AE, Costa B, Borsani E, Franchi S, Magnaghi V, et al. The purinergic antagonist PPADS reduces pain related behaviours and interleukin-1 beta, interleukin-6, iNOS and nNOS overproduction in central and peripheral nervous system after peripheral neuropathy in mice. Pain. 2008; 137:81–95. DOI: 10.1016/j.pain.2007.08.017. PMID: 17900807.


69. Apfel SC. Neurotrophic factors in peripheral neuropathies: therapeutic implications. Brain Pathol. 1999; 9:393–413. DOI: 10.1111/j.1750-3639.1999.tb00234.x. PMID: 10219753.


70. Alexander GM, van Rijn MA, van Hilten JJ, Perreault MJ, Schwartzman RJ. Changes in cerebrospinal fluid levels of pro-inflammatory cytokines in CRPS. Pain. 2005; 116:213–9. DOI: 10.1016/j.pain.2005.04.013. PMID: 15964681.


71. Cova L, Armentero MT, Zennaro E, Calzarossa C, Bossolasco P, Busca G, et al. Multiple neurogenic and neurorescue effects of human mesenchymal stem cell after transplantation in an experimental model of Parkinson’s disease. Brain Res. 2010; 1311:12–27. DOI: 10.1016/j.brainres.2009.11.041. PMID: 19945443.


72. Koh SH, Kim KS, Choi MR, Jung KH, Park KS, Chai YG, et al. Implantation of human umbilical cord-derived mesenchymal stem cells as a neuroprotective therapy for ischemic stroke in rats. Brain Res. 2008; 1229:233–48. DOI: 10.1016/j.brainres.2008.06.087. PMID: 18634757.


73. Reid AJ, Sun M, Wiberg M, Downes S, Terenghi G, Kingham PJ. Nerve repair with adipose-derived stem cells protects dorsal root ganglia neurons from apoptosis. Neuroscience. 2011; 199:515–22. DOI: 10.1016/j.neuroscience.2011.09.064. PMID: 22020320.


74. Park HJ, Lee PH, Bang OY, Lee G, Ahn YH. Mesenchymal stem cells therapy exerts neuroprotection in a progressive animal model of Parkinson’s disease. J Neurochem. 2008; 107:141–51. DOI: 10.1111/j.1471-4159.2008.05589.x. PMID: 18665911.


75. Edalatmanesh MA, Bahrami AR, Hosseini E, Hosseini M, Khatamsaz S. Neuroprotective effects of mesenchymal stem cell transplantation in animal model of cerebellar degeneration. Neurol Res. 2011; 33:913–20. DOI: 10.1179/1743132811Y.0000000036. PMID: 22080991.


76. Meirelles Lda S, Fontes AM, Covas DT, Caplan AI. Mechanisms involved in the therapeutic properties of mesenchymal stem cells. Cytokine Growth Factor Rev. 2009; 20:419–27. DOI: 10.1016/j.cytogfr.2009.10.002. PMID: 19926330.


77. Ghannam S, Bouffi C, Djouad F, Jorgensen C, Noël D. Immunosuppression by mesenchymal stem cells: mechanisms and clinical applications. Stem Cell Res Ther. 2010; 1:2. DOI: 10.1186/scrt2. PMID: 20504283. PMCID: PMC2873698.


78. Zhang EJ, Song CH, Ko YK, Lee WH. Intrathecal administration of mesenchymal stem cells reduces the reactive oxygen species and pain behavior in neuropathic rats. Korean J Pain. 2014; 27:239–45. DOI: 10.3344/kjp.2014.27.3.239. PMID: 25031809. PMCID: PMC4099236.


79. Jeong JO, Kim MO, Kim H, Lee MY, Kim SW, Ii M, et al. Dual angiogenic and neurotrophic effects of bone marrow-derived endothelial progenitor cells on diabetic neuropathy. Circulation. 2009; 119:699–708. DOI: 10.1161/CIRCULATIONAHA.108.789297. PMID: 19171856. PMCID: PMC2746559.


80. Naruse K, Sato J, Funakubo M, Hata M, Nakamura N, Kobayashi Y, et al. Transplantation of bone marrow-derived mononuclear cells improves mechanical hyperalgesia, cold allodynia and nerve function in diabetic neuropathy. PLoS One. 2011; 6:e27458. DOI: 10.1371/journal.pone.0027458. PMID: 22125614. PMCID: PMC3220696.


81. Anitha M, Gondha C, Sutliff R, Parsadanian A, Mwangi S, Sitaraman SV, et al. GDNF rescues hyperglycemia-induced diabetic enteric neuropathy through activation of the PI3K/ Akt pathway. J Clin Invest. 2006; 116:344–56. DOI: 10.1172/JCI26295. PMID: 16453021. PMCID: PMC1359053.
82. Tse HF, Siu CW, Zhu SG, Songyan L, Zhang QY, Lai WH, et al. Paracrine effects of direct intramyocardial implantation of bone marrow derived cells to enhance neovascularization in chronic ischaemic myocardium. Eur J Heart Fail. 2007; 9:747–53. DOI: 10.1016/j.ejheart.2007.03.008. PMID: 17481945.


83. Shibata T, Naruse K, Kamiya H, Kozakae M, Kondo M, Yasuda Y, et al. Transplantation of bone marrow-derived mesenchymal stem cells improves diabetic polyneuropathy in rats. Diabetes. 2008; 57:3099–107. DOI: 10.2337/db08-0031. PMID: 18728233. PMCID: PMC2570407.


84. Kim BJ, Jin HK, Bae JS. Bone marrow-derived mesenchymal stem cells improve the functioning of neurotrophic factors in a mouse model of diabetic neuropathy. Lab Anim Res. 2011; 27:171–6. DOI: 10.5625/lar.2011.27.2.171. PMID: 21826178. PMCID: PMC3146005.


85. Waterman RS, Tomchuck SL, Henkle SL, Betancourt AM. A new mesenchymal stem cell (MSC) paradigm: polarization into a pro-inflammatory MSC1 or an immunosuppressive MSC2 phenotype. PLoS One. 2010; 5:e10088. DOI: 10.1371/journal.pone.0010088. PMID: 20436665. PMCID: PMC2859930.


86. Waterman RS, Morgenweck J, Nossaman BD, Scandurro AE, Scandurro SA, Betancourt AM. Anti-inflammatory mesenchymal stem cells (MSC2) attenuate symptoms of painful diabetic peripheral neuropathy. Stem Cells Transl Med. 2012; 1:557–65. DOI: 10.5966/sctm.2012-0025. PMID: 23197860. PMCID: PMC3659725.


87. Comerota AJ, Link A, Douville J, Burchardt ER. Upper extremity ischemia treated with tissue repair cells from adult bone marrow. J Vasc Surg. 2010; 52:723–9. DOI: 10.1016/j.jvs.2010.04.020. PMID: 20576396.


88. Yezierski RP. Pain following spinal cord injury: the clinical problem and experimental studies. Pain. 1996; 68:185–94. DOI: 10.1016/S0304-3959(96)03178-8. PMID: 9121805.


89. Satake K, Matsuyama Y, Kamiya M, Kawakami H, Iwata H, Adachi K, et al. Up-regulation of glial cell line-derived neurotrophic factor (GDNF) following traumatic spinal cord injury. Neuroreport. 2000; 11:3877–81. DOI: 10.1097/00001756-200011270-00054. PMID: 11117507.


90. Schnell L, Schneider R, Kolbeck R, Barde YA, Schwab ME. Neurotrophin-3 enhances sprouting of corticospinal tract during development and after adult spinal cord lesion. Nature. 1994; 367:170–3. DOI: 10.1038/367170a0. PMID: 8114912.


91. Eaton MJ, Wolfe SQ, Martinez M, Hernandez M, Furst C, Huang J, et al. Subarachnoid transplant of a human neuronal cell line attenuates chronic allodynia and hyperalgesia after excitotoxic spinal cord injury in the rat. J Pain. 2007; 8:33–50. DOI: 10.1016/j.jpain.2006.05.013. PMID: 17207742.


92. Hofstetter CP, Schwarz EJ, Hess D, Widenfalk J, El Manira A, Prockop DJ, et al. Marrow stromal cells form guiding strands in the injured spinal cord and promote recovery. Proc Natl Acad Sci U S A. 2002; 99:2199–204. DOI: 10.1073/pnas.042678299. PMID: 11854516. PMCID: PMC122342.


93. Yang CC, Shih YH, Ko MH, Hsu SY, Cheng H, Fu YS. Transplantation of human umbilical mesenchymal stem cells from Wharton’s jelly after complete transection of the rat spinal cord. PLoS One. 2008; 3:e3336. DOI: 10.1371/journal.pone.0003336. PMID: 18852872. PMCID: PMC2566594.


94. Yousefifard M, Rahimi-Movaghar V, Nasirinezhad F, Baikpour M, Safari S, Saadat S, et al. Neural stem/progenitor cell transplantation for spinal cord injury treatment; a systematic review and meta-analysis. Neuroscience. 2016; 322:377–97. DOI: 10.1016/j.neuroscience.2016.02.034. PMID: 26917272.


95. Macias MY, Syring MB, Pizzi MA, Crowe MJ, Alexanian AR, Kurpad SN. Pain with no gain: allodynia following neural stem cell transplantation in spinal cord injury. Exp Neurol. 2006; 201:335–48. DOI: 10.1016/j.expneurol.2006.04.035. PMID: 16839548.


96. Klass M, Gavrikov V, Drury D, Stewart B, Hunter S, Denson DD, et al. Intravenous mononuclear marrow cells reverse neuropathic pain from experimental mononeuropathy. Anesth Analg. 2007; 104:944–8. DOI: 10.1213/01.ane.0000258021.03211.d0. PMID: 17377111.


97. Vadivelu S, Willsey M, Curry DJ, McDonald JW 3rd. Potential role of stem cells for neuropathic pain disorders. Neurosurg Focus. 2013; 35:E11. DOI: 10.3171/2013.6.FOCUS13235. PMID: 23991814.


98. Choi JI, Cho HT, Jee MK, Kang SK. Core-shell nanoparticle controlled hATSCs neurogenesis for neuropathic pain therapy. Biomaterials. 2013; 34:4956–70. DOI: 10.1016/j.biomaterials.2013.02.037. PMID: 23582861.


99. Franchi S, Valsecchi AE, Borsani E, Procacci P, Ferrari D, Zalfa C, et al. Intravenous neural stem cells abolish nociceptive hypersensitivity and trigger nerve regeneration in experimental neuropathy. Pain. 2012; 153:850–61. DOI: 10.1016/j.pain.2012.01.008. PMID: 22321918.


100. Sacerdote P, Niada S, Franchi S, Arrigoni E, Rossi A, Yenagi V, et al. Systemic administration of human adipose-derived stem cells reverts nociceptive hypersensitivity in an experimental model of neuropathy. Stem Cells Dev. 2013; 22:1252–63. DOI: 10.1089/scd.2012.0398. PMID: 23190263. PMCID: PMC3613970.


101. Coronel MF, Musolino PL, Brumovsky PR, Hökfelt T, Villar MJ. Bone marrow stromal cells attenuate injury-induced changes in galanin, NPY and NPY Y1-receptor expression after a sciatic nerve constriction. Neuropeptides. 2009; 43:125–32. DOI: 10.1016/j.npep.2008.12.003. PMID: 19168218.


102. Lee S, Moon CS, Sul D, Lee J, Bae M, Hong Y, et al. Comparison of growth factor and cytokine expression in patients with degenerated disc disease and herniated nucleus pulposus. Clin Biochem. 2009; 42:1504–11. DOI: 10.1016/j.clinbiochem.2009.06.017. PMID: 19563795.


103. Orozco L, Soler R, Morera C, Alberca M, Sánchez A, García-Sancho J. Intervertebral disc repair by autologous mesenchymal bone marrow cells: a pilot study. Transplantation. 2011; 92:822–8. DOI: 10.1097/TP.0b013e3182298a15. PMID: 21792091.


104. Franchi S, Castelli M, Amodeo G, Niada S, Ferrari D, Vescovi A, et al. Adult stem cell as new advanced therapy for experimental neuropathic pain treatment. Biomed Res Int. 2014; 2014:470983. DOI: 10.1155/2014/470983. PMID: 25197647. PMCID: PMC4147203.


105. Ikebe C, Suzuki K. Mesenchymal stem cells for regenerative therapy: optimization of cell preparation protocols. Biomed Res Int. 2014; 2014:951512. DOI: 10.1155/2014/951512.


106. Koga H, Engebretsen L, Brinchmann JE, Muneta T, Sekiya I. Mesenchymal stem cell-based therapy for cartilage repair: a review. Knee Surg Sports Traumatol Arthrosc. 2009; 17:1289–97. DOI: 10.1007/s00167-009-0782-4. PMID: 19333576.


107. Van Osch GJ, Van Der Veen SW, Burger EH, Verwoerd-Verhoef HL. Chondrogenic potential of in vitro multiplied rabbit perichondrium cells cultured in alginate beads in defined medium. Tissue Eng. 2000; 6:321–30. DOI: 10.1089/107632700418047. PMID: 10992429.


108. Wiesmann A, Bühring HJ, Mentrup C, Wiesmann HP. Decreased CD90 expression in human mesenchymal stem cells by applying mechanical stimulation. Head Face Med. 2006; 2:8. DOI: 10.1186/1746-160X-2-8. PMID: 16573842. PMCID: PMC1483821.


Fig. 1
Three types of stem cells. After the sperm enters the ovum using the acrosome head to break the zona pellucida, the fertilized egg is called as a zygote before it divides into 16 cells (the morula). The blastocyst formation begins 5 days after fertilization. The blastocyst has 2 types of blastomere cells: the inner cell mass (embryoblast) and trophoblast. The inner cell mass becomes the embryo (till 8 wk), and then the fetus (from 9 wk). The embryonic stem cells are derived from the inner cell mass of the blastocyst. The induced pluripotent stem cells are made from the differentiated somatic cells of the adult, affected by Yamanaka factor. The adult stem cells vary from 1) the hematopoietic stem cells, derived from the umbilical cord of the fetus, 2) the bone marrow mesenchymal stem cells and hematopoietic stem cells, harvested from the bone marrow of an adult, 3) the adipose mesenchymal stem cells, derived from adipose tissue, and 4) the organ mesenchymal stem cells, harvested from the adult body. The embryonic and induced pluripotent stem cells have pluripotent potential; the adult stem cells have multipotent properties. Self-renewal ability, the malignancy rate, and the possibility of ethical problems are increasingly higher in the adult stem cells, induced pluripotent stem cells, and embryonic stem cells, respectively.
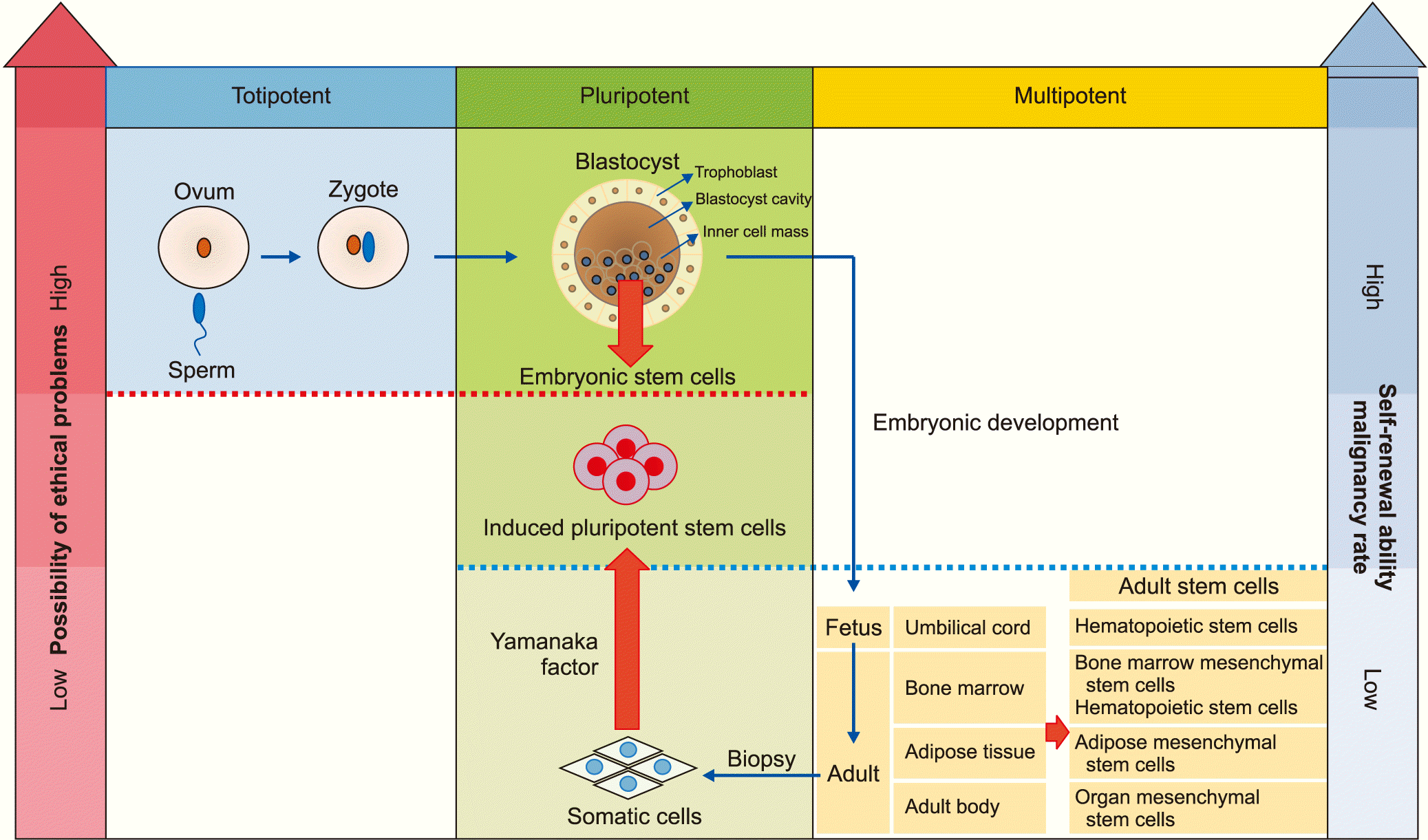