Abstract
The microRNAs (miRNAs) are small, non-coding RNAs that modulate protein expression by interfering with target mRNA translation or stability. miRNAs play crucial roles in various functions such as cellular, developmental, and physiological processes. The spatial expression patterns of miRNAs are very essential for identifying their functions. The expressions of miR-302 and miR-367 are critical in maintaining stemness of pluripotent stem cells, including embryonic stem cells (ESCs) and induced pluripotent stem cells (iPSCs) but their functions in early development are not fully elucidated. So, we used Locked Nucleic Acid (LNA) probes to perform in situ hybridization and confirmed the temporal and spatial distribution patterns during early chick development. As a result, we found that miR-302 and miR-367 were expressed in various tissues such as primitive steak, neural ectoderm, neural plate, neural fold, neural tube, notochord, and oral cavity. Specially, we confirmed that miR-302 and miR-367 were strongly expressed in neural folds in HH8 to HH10. miR-302 was expressed on dorsal part of the neural tube but miR-367 was expressed on lateral and ventral parts of the neural tube. And also we performed quantitative stem-loop real-time PCR to analyze global expression level of miR-302 and miR-367. miR-302 and miR-367 expression was sustained before Hamburger and Hamilton stage (HH) 14. Thus, the temporal and spatial expression patterns of miR-302 and miR-367 may provide us information of the role of these miRNAs on tissue formation during early chick development.
The microRNAs (miRNAs) are small non-coding RNAs of 18–25 nucleotides in length that modulate the expressions of genes by inhibiting translation or cleaving complementary target mRNAs (1). The silencing mechanism of miRNAs are mediated by the complementary base-pairing sequence to the 3′ untranslated region (UTR) of the targeted mRNAs, resulting in mRNA cleavage, deadenylation, or translational repression (2). The miRNAs are involved in the regulation of various cellular functions such as cell proliferation, apoptosis, differentiation, tumorigenesis, and vertebrate development (1, 3). Since miRNAs were initially discovered in 1993 in Caenorhabditis elegans (4), Currently, more than 17,000 miRNAs have been identified (5).
In vertebrates, most miRNAs are expressed in a tissue-specific manner (6). A variety of miRNAs are expressed during developmental process and in a stage-specific manner (7). Unique expression of miRNA in any cell type explains its role on tissue specification. miR-302-367 known as downstream effectors of Oct4, Sox2, and Nanog (8) are the embryonic stem cell (ESC)-specific miRNAs (9, 10) and function as self-renewal, stemness, and inhibiting differentiation in ESCs (11). Introduction of miR-302-367 cluster into somatic cells can reprogram into pluripotent stem cell (12). But, in gain and loss of function study, miR-302 in hESCs promotes the mesendodermal lineage and miR-427 (ortholog of miR-302) controls mesodermal patterning and organizer induction in xenopus laevis (13).
Hence, we hypothesized that miR-302 and miR-367 have crucial roles in early tissue development. To understand the functions of these miRNAs, we performed to establish a high-resolution profile of their spatial and temporal distribution according to developmental stages of early chick.
Locked Nucleic Acids (LNAs), a novel type of RNA analog, as miRNA detection probes have extremely high specificity and stability with RNA sequences and so can detect small mature miRNAs, specially for whole mount in situ hybridization detection (14, 15).
In this study, we used the early chick embryos, a classic model of vertebrate developmental biology and an alternative to mouse for study (16). We performed a whole mount in situ hybridization (WISH) on miR-302, and miR-367 and analyzed their spatial and temporal expressions during early chick development according to Hamburger and Hamilton (HH) stages (17).
Fertilized chicken eggs were purchased from poultry breeding farms. Fertile chicken eggs were incubated in a humidified incubator at 38°C to develop. Chicken embryos at different developmental stages corresponding to HH stages were isolated (17).
We used the Locked Nucleic Acid (LNA) is a class of bicyclic high-affinity RNA analogs in which the furanose ring of the ribose sugar is chemically locked in an NA-mimicking conformation by the introduction of an O2’,C4’-methylene bridge, leading to high affinity toward complementary RNA molecules. All LNA- modified DNA oligonucleotides probes (miRCURY detection probes, product number; 37104 for miR-302 detcetion, 381725 for miR-367 detcetion) were supplied from Exiqon (Denmark). LNA probes were labeled with digoxigenin-ddUTP using the the 3′ end labeling kit (Roche) according to the manufacturers recommendations.
For in situ hybridization, chick embryos were collected in chilled chick saline (123 mM NaCl in nanopure water). Embryos were fixed in fresh, cold 4% paraformaldehyde in PBS, pH 7.2 overnight at 4°C. Embryos were then rinsed in PBS and stored in methanol at −20°C for no more than 5 days. Embryos were rehydrated in a series from methanol to phosphate-buffered saline (PBS, pH 7.4) containing 0.1% Tween-20 (PBT). Embryos were treated with 10 μg/ml proteinase K for 5 to 10 min at room temperature. Embryos were washed gently and re-fixed in 4% paraformaldehyde/0.1% glutaraldehyde. After further washing in PBT, the embryos were transferred to a standard prehybridization solution (50% formamide, 5×SSC, 2% blocking powder, 0.1% Tween-20, 0.1% CHAPS, 50 μg/ml yeast RNA, 5 mM EDTA, 50 μg/ml heparin in DEPC water) for 2 hr in EP tubes in a shaking hybridization oven at a temperature between 20∼25°C below the reported melting temperature of the LNAs (15). After 1 h, probe was added to the embryos to a final concentration of 1 μg/ml and incubated overnight. Embryos were washed in 2×SSC, 1% CHAPS in the hybridization temperature. Embryos were washed 3×20 min in the high salt wash, then 3×20 min in 0.2×SSC, 0.1% Chaps, and then rinsed twice in TBT (50 mM Tris, pH 7.5, 150 mM NaCl, 10 mM KCl, 1% Tween-20). Embryos were pre-treated in 20% sheep serum in TBT at 4°C for 2∼3 hr. Sheep anti-digoxygenin antibody coupled to alkaline phosphatase (Roche) was added at a concentration of 1:1000 and the embryos incubated overnight. After washing, color development was carried out in alkaline phosphatase buffer (100 mM Trip pH 9.5, 50 mM MgCl2, 100 mM NaCl, 0.1% Tween-20) with NBT (338 μg/ml) and BCIP (175 μg/ml). Reactions were stopped with TBT and embryos were then washed in PBS, dehydrated by a methanol series to remove background and enhance signal, then rehydrated and stored in PBS plus 0.1% sodium azide.
We used TaqMan miRNA assays as previously described (18). Reverse transcription (RT) reactions were run in a GeneAmp PCR 9,700 Thermocycler (Applied Biosystems, CA). Gene expression levels were quantified using the ABI 7300 RT-PCR System (Applied Biosytems). Comparative real-time PCR were performed in triplicate using each specific primer for miR-302 (ID: 000529), miR-367 (ID: 000555), and 18S rRNA (ID: Hs99999901_s1). Reactions were performed at 95°C for 10 minutes, followed by 40 cycles of 95°C for 15 seconds, and 60°C for 1 minute. (USA). The expression of each miRNA relative to 18S rRNA was determined using the 2−ΔΔCt method (19). All primer sets for specific miRNAs and PCR reagents for Taqman miRNA assay were purchased from Applied Biosystems.
Embryos were photographed on a Leica PlanApo stereo-microscope using a digital acquisition system and transmitted, lateral and/or direct illumination. Sections were cut at 14 μm, mounted using Cytoseal XYL (Richard-Allan Scientific), and photographed using DIC optics on a Leica DMRXE microscope. Images were modified using Adobe Photoshop only to correct brightness, contrast, and color balance.
We performed whole mount in situ hybridization (Fig. 1A–F) and then transverse section (Fig. 1A–F black lines) to confirm the expression pattern of miR-302 in HH stages 1–13 embryos. miR-302 was expressed in epiblast (Fig. 1A1, B1), primitive streak (Fig. 1A2, B2, C2, D arrowhead), neural plate (Fig. 1C1, D1, D2), neural fold (Fig. 1C1, D1, D2), prosencephalon (Fig. 1E1), oral cavity (Fig. 1E1), neural tube (Fig. 1E2), and notochord (Fig. 1F1). Our result indicated that miR-302 was more strongly expressed in both neural fold at HH8 (Fig. 1D1) and dorsal parts in neural tubes in HH10 and HH13 (Fig. 1E2, F1 dotted rectangle) but not observed in the hypoblast (Fig. 1A1, B1, C1). The qPCR result showed that the miR-302 expression level was highly expressed at HH5 and then dramatically reduced (Fig. 1G).
miR-367 expression pattern was clarified by whole mount in situ hybridization (Fig. 2A–F) and transverse sections (Fig. 2A–F black lines) was performed in chick embryos from HH stages 1 to 12. The expression pattern of miR-367 was similar to that of miR-302 during the embryonic chick development. miR-367 was expressed in epiblast (Fig. 2A1, B1), primitive streak (Fig. 2A2, B2, C2, D arrowhead), neural plate (Fig. 2C1, D1), neural fold (Fig. 1C1, D1), prosencephalon (Fig. 2E1), oral cavity (Fig. 2E1), neural tube (Fig. 2E2), and notochord (Fig. 2D1, F2). miR-367 was strongly expressed in neural fold at HH8 (Fig. 2D1). At HH10 and HH12, miR-367 expression was reduced in dorsal parts of developing chick embryos (Fig. 2E1, E2, F1, F1 dotted rectangle). Similar to miR-302, miR-367 also was not detected in the hypoblast (Fig. 2A1, B1, C1). Highly expressed miR-367 by qPCR was observed at HH4 and HH5 compared with other stages (Fig. 2G).
Originally, miR-302 cluster expressed only in pluripotent tissue of embryos and undifferentiated pluripotent stem cells in mouse and human (9, 10). Recent studies showed that miR-302 targets epigenetic regulators such as AOF1/2, MECP1-p66, MECP2 and MBD2, cell cycle regulators such as Cyclin D1/D2, CDK2, BMI-1 and PTEN, TGF-β regulators such as Lefty1/2 and TGFBR2, and BMP inhibitors including DAZAP2, SLAIN1 and TOB2 (20).
However, using mouse and human developmental embryos as a model system is technically high demanding and human system has bioethical problems. To utilize the benefit of the chick embryo development system as a developmental model in vitro for miRNA study, we tested the expression pattern of miR-302 and miR-367.
miR-302 and miR-367 were expressed in various ectodermal tissues, primitive streak, and notochord, a mesodermal tissue. Specifically, miR-302 and miR-367 were more strongly expressed in both neural folds at HH8 (Fig. 1D1, Fig. 2D1), in which neural fold and neural crest cells are induced by SLUG gene (21). miR-302 was concentrated on the dorsal part of neural tubes after HH10 (Fig. 1E2, F1), while miR-367 was concentrated on the lateral and ventral part of neural tubes after HH10 (Fig. 2E1, E2, F1, F2).
miR-367 is one of the members of miR-302 cluster and its seed sequences (AUUGCA) are not same as those of miR-302 (AAGUGC). miR-367 with the same seeds with miR-25 gene family may be related with cell proliferation during morphogenesis (http://www.mirbase.org). Spatial expression patterns of miR-302 and miR-367 were a little different in neural tubes in HH10, HH12, and HH13 (Fig. 1 and Fig. 2 dotted rectangle). Recently, Rosa et al. reported that miR-302 is specifically and abundantly expressed in embryonic/epiblast region of the mouse embryo during gastrulation and absent in extraembryonic tissues. They also mentioned that this miRNA enriched in the primitive streak region as well as in neural anterior ectoderm and after this developmental stage, its expression sharply decreased and becomes confined to the cranial neural folds (13).
As a consequence, even though miR-302 and miR-367 have different seed sequences and classified as different family type, they transcribed polycistronically from its miRNA gene. Our data suggest that these temporally and spatially expressed miRNAs can be involvement in early morphogenesis and neural development in chick based on their expression patterns.
ACKNOWLEDGMENTS
This research was supported by the National Research Foundation of Korea (NRF) funded by the Ministry of Science, ICT & Future Planning (2012M3A9B4028738).
References
1. Bartel DP. MicroRNAs: genomics, biogenesis, mechanism, and function. Cell. 2004. 116:281–297.
2. Filipowicz W, Bhattacharyya SN, Sonenberg N. Mechanisms of post-transcriptional regulation by microRNAs: are the answers in sight? Nat Rev Genet. 2008. 9:102–114.


3. He L, Hannon GJ. MicroRNAs: small RNAs with a big role in gene regulation. Nat Rev Genet. 2004. 5:522–531.


4. Lee RC, Feinbaum RL, Ambros V. The C. elegans heterochronic gene lin-4 encodes small RNAs with antisense complementarity to lin-14. Cell. 1993. 75:843–854.


5. Kozomara A, Griffiths-Jones S. miRBase: integrating microRNA annotation and deep-sequencing data. Nucleic Acids Res. 2011. 39(Database issue):D152–D157.


6. Lagos-Quintana M, Rauhut R, Yalcin A, Meyer J, Lendeckel W, Tuschl T. Identification of tissue-specific microRNAs from mouse. Curr Biol. 2002. 12:735–739.


7. Watanabe T, Takeda A, Mise K, Okuno T, Suzuki T, Minami N, Imai H. Stage-specific expression of microRNAs during Xenopus development. FEBS Lett. 2005. 579:318–324.
8. Marson A, Levine SS, Cole MF, Frampton GM, Brambrink T, Johnstone S, Guenther MG, Johnston WK, Wernig M, Newman J, Calabrese JM, Dennis LM, Volkert TL, Gupta S, Love J, Hannett N, Sharp PA, Bartel DP, Jaenisch R, Young RA. Connecting microRNA genes to the core transcriptional regulatory circuitry of embryonic stem cells. Cell. 2008. 134:521–533.


9. Houbaviy HB, Murray MF, Sharp PA. Embryonic stem cell-specific MicroRNAs. Dev Cell. 2003. 5:351–358.


10. Suh MR, Lee Y, Kim JY, Kim SK, Moon SH, Lee JY, Cha KY, Chung HM, Yoon HS, Moon SY, Kim VN, Kim KS. Human embryonic stem cells express a unique set of microRNAs. Dev Biol. 2004. 270:488–498.


11. Kim KS, Kim JS, Lee MR, Jeong HS, Kim J. A study of microRNAs in silico and in vivo: emerging regulators of embryonic stem cells. FEBS J. 2009. 276:2140–2149.


12. Anokye-Danso F, Trivedi CM, Juhr D, Gupta M, Cui Z, Tian Y, Zhang Y, Yang W, Gruber PJ, Epstein JA, Morrisey EE. Highly efficient miRNA-mediated reprogramming of mouse and human somatic cells to pluripotency. Cell Stem Cell. 2011. 8:376–388.


13. Rosa A, Spagnoli FM, Brivanlou AH. The miR-430/427/302 family controls mesendodermal fate specification via species-specific target selection. Dev Cell. 2009. 16:517–527.


14. Wienholds E, Kloosterman WP, Miska E, Alvarez-Saavedra E, Berezikov E, de Bruijn E, Horvitz HR, Kauppinen S, Plasterk RH. MicroRNA expression in zebrafish embryonic development. Science. 2005. 309:310–311.


15. Kloosterman WP, Wienholds E, de Bruijn E, Kauppinen S, Plasterk RH. In situ detection of miRNAs in animal embryos using LNA-modified oligonucleotide probes. Nat Methods. 2006. 3:27–29.


16. Brown WR, Hubbard SJ, Tickle C, Wilson SA. The chicken as a model for large-scale analysis of vertebrate gene function. Nat Rev Genet. 2003. 4:87–98.


17. Hamburger V, Hamilton HL. A series of normal stages in the development of the chick embryo. 1951. Dev Dyn. 1992. 195:231–272.
18. Chen C, Ridzon DA, Broomer AJ, Zhou Z, Lee DH, Nguyen JT, Barbisin M, Xu NL, Mahuvakar VR, Andersen MR, Lao KQ, Livak KJ, Guegler KJ. Real-time quantification of microRNAs by stem-loop RT-PCR. Nucleic Acids Res. 2005. 33:e179.


19. Livak KJ, Schmittgen TD. Analysis of relative gene expression data using real-time quantitative PCR and the 2(−Delta Delta C(T)) Method. Methods. 2001. 25:402–408.


Fig. 1.
Expression patterns and level of miR-302 during early chick development. Embryos expressed miR-302 by HH stages 4–13 (A–F). Black lines indicate level of tranverse sections presented in right or left beside of each embryo. miR-302 was expressed in epiblast (A1, B1), primitive streak (A2, B2, C2, D arrowhead), neural plate (C1, D1, D2), neural fold (C1, D1, D2), prosencephalon (E1), oral cavity (E1), neural tube (E2), and notochord (F1). Expression level of miR-302 was much higher at HH5 compared with other stages Relative expression value of miR-302 was normalized to the level of 18s mRNA(G). Bars represent mean±SEM. Student’s t-test: *p<0.05 and ***p<0.001 vs. HH1. Abbreviations: epi, epiblast; hyp, hyperblast; fg, foregut; nc, notochord; np, neural plate; nf, neural fold; nt, neurlal tube; oc, oral cavity; ps, primitive streak; pros, prosencephalon; sm, somite; HH, Hamburger and Hamilton.
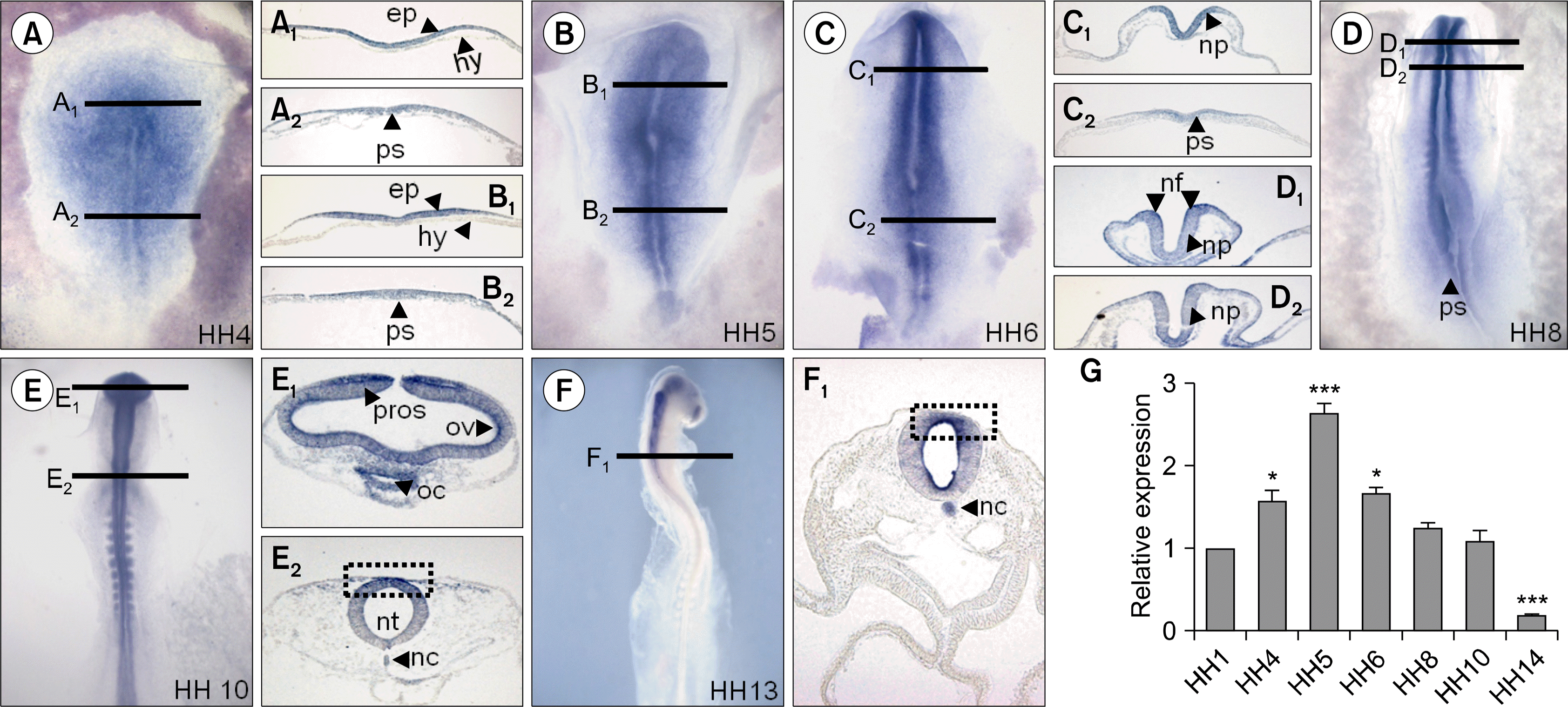
Fig. 2.
Differential expression patterns and level of miR-367 in early chick embryos. miR-367 was expressed in epiblast (A1, B1), primitive streak (A2, B2, C2, D arrowhead), neural plate (C1, D1), neural fold (C1, D1), prosencephalon (E1), Oral cavity (E1), neural tube (E2), notochord (D1, F1). Higher expression of miR-367 was detected at HH4 and HH5 during chick development. Relative expression value of miR-367 was normalized to the level of 18s mRNA(G). Bars represent mean±SEM. Student’s t-test: *p<0.05, **p<0.01, and ***p<0.001 vs. HH1.
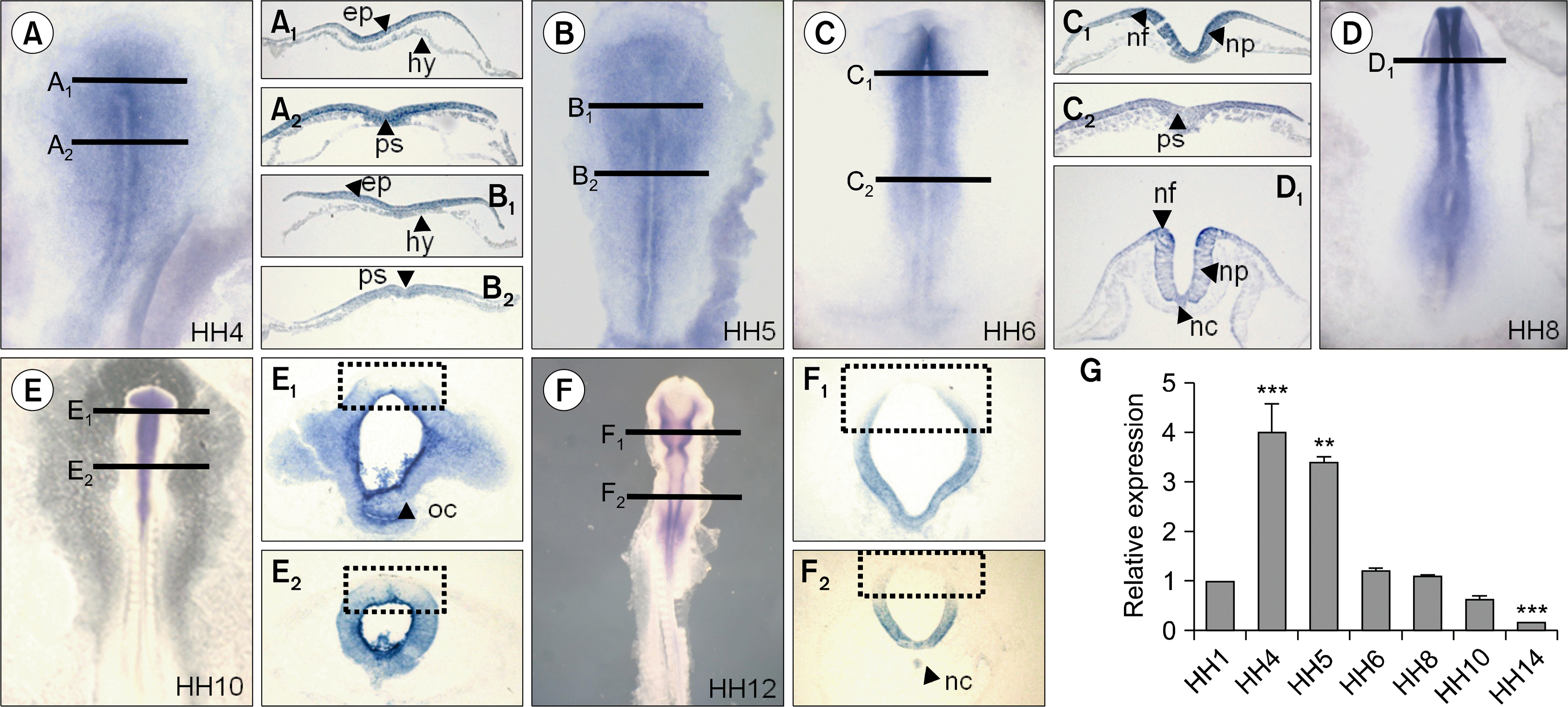