Abstract
Background and Objectives:
Recent findings suggest that therapeutic potential of mesenchymal stem cells (MSCs) could be increased through aggregation into three-dimensional (3D) bodies, and different culture methods have been employed to obtain 3D spheroids of MSCs. In the current study we report accidentally encountered spontaneous formation of adipose-derived stem cell (ASC) bodies in standard ASC culture of a single donor.
Methods and Results:
Human ASCs from passages 1 to 3, cultured in a medium containing 5% autologous serum (AS), spontaneously clustered and formed floating 3D bodies. After a transfer of floating ASC bodies onto new adherent plastic dish, they attached to the surface and gradual migration of spindle-shaped ASCs out of the bodies was detected. A substitution of AS with allogeneic sera did not hinder this ability, but commercial medium containing fetal bovine serum delayed the process. Substantial part of ASCs surrounding transferred ASC bodies showed alkaline phosphatase (AP) activity, while ASC aggregates were AP negative. Similar 3D bodies formed when ASCs were grown on an uncoated glass surface. These ASC aggregates as well as clusters of ASCs, where formation of the 3D bodies is initiated, expressed pluripotency marker NANOG, but the expression of OCT4A was not detected.
Conclusions:
Obtained results suggest that spontaneously formed ASC aggregates may represent a more primitive cell subpopulation within the individual ASC culture. The ability to form 3D aggregates, the expression of NANOG, and the lack of the AP activity may be used to enrich ASC cultures with potentially more primitive cells serving as an excellent basis for therapeutic applications.
Although bone marrow (BM) is considered to be the main source of mesenchymal stem cells (MSCs), adipose tissue can also be used to easily extract MSCs (1). Adipose-derived stem cells (ASCs) share their fibroblast-like morphology, plastic adherence, expression of group of surface markers, and multilineage differentiation potential with BM MSCs (2). But unlike BM MSCs, ASCs are easy to isolate and the amount of obtained stem cells from one millilitre of adipose tissue is 500-fold greater than from BM (3). It has been shown that ASCs possess the ability to differentiate not only into adipocytes, osteocytes and chondrocytes (1), but also into skeletal muscle cells (4), cardiomyocytes (5), neurons (6), endothelial cells (7), hepatocytes (8), and pancreatic cells (9).
In the field of embryonic stem cell (ESC) research it is well-established practice to characterize ESCs by the expression of specific pluripotency markers that signifies their ability to self renew and differentiate into each cell type within the organism, except extraembryonic tissues. OCT4, NANOG and SOX2 are regarded as the central transcriptional regulators maintaining the pluripotency of ESCs (10). All three key transcription factors are tightly interacted in order to regulate a set of target genes as well as an expression level of one another (11). In the last decade numerous studies have detected OCT4 and other pluripotency markers in adult stem cells (12, 13). These findings have led to speculations that somatic stem cells may possess greater similarities to ESCs in terms of regulatory networks and potency than previously thought (14). However, additional scientific research contrasts to confront this hypothesis by evidence that OCT4 is not essential for maintaining potency and self renewal in the adult mammalian stem cells (15). In addition, alternative splice variants of OCT4, resulting in three isoforms termed OCT4A, OCT4B, and OCT4B1 (16), numerous NANOG and OCT4 pseudogenes (17, 18), as well as vaguely designed studies and poorly chosen methods of detection can also result into misleading conclusions (19).
Another direction where relation between somatic stem cells and ESCs is being explored lies in the culturing of these cells. Three-dimensional (3D) cell culture method has been commonly used in ESC cultures to initiate spontaneous in vitro differentiation through embryoid body formation (20). It has also been widely exploited in neural stem cell studies to propagate, characterize and assay these cells by means of neurosphere system (21) and in cancer research to simulate the tumour environment (22). Recently similar techniques have been successfully applied to aggregate adult stem cells in order to increase their differentiation properties and therapeutic potential (23–26). As 3D culture methods facilitate higher cell-cell and cell-extracellular matrix interactions and are believed to hold a greater promise in mimicking in vivo environment than monolayer culture (24, 25), the use of MSCs in cell therapy is advancing at a rapid pace towards extensive clinical applications (27).
In order to obtain 3D adult stem cell aggregates such methods as cell culture onto non-adherent surfaces, hanging drop technique, forced-aggregation, surface treatment, and microfabrication have been used (for review, see 27). In this study we describe spontaneous ASC aggregate formation without the use of any of above-mentioned techniques and analyse their growth properties, alkaline phosphatase (AP) activity and expression of pluripotency markers in comparison with monolayer ASCs. The ASC aggregates reported herein have not been purposely obtained. This manuscript describes the unique observation accidentally encountered in standard ASC culture of a single donor.
The Latvian Central Medical Ethics Committee has approved the current research involving human participants (permit No.12) and human adipose tissue and blood were collected after written consent was obtained from the donor. Human adipose tissue from abdominal cavity, derived during planned operation, and blood were collected from the healthy, 40 years old man with body mass index 26.3. Blood and tissue samples were processed in collaboration with “Cilmes Šūnu Tehnoloģijas” Ltd. within 3 hours after collection.
Collected blood was allowed to clot for 1 hour at room temperature. The serum was collected, centrifuged at 2000 rpm for 30 min, filtered through 0.2 μm mesh, aliquoted, and stored at −20°C.
2 ml of adipose tissue were scissored and treated with 0.3% pronase (EMD Millipore) for 1 h at RT with gentle rotation followed by centrifugation for 7 min at 1000 rpm. The pellet was suspended, filtered through 40 μm mesh and centrifuged again for 5 min. Erythrocytes were lysed for 3 min at +37°C using erythrocyte lysis buffer Hybri-Max (Sigma-Aldrich). Obtained cell pellet was suspended in a fresh cell culture medium (DMEM/F-12 (Life Technologies) containing 10% autologous serum (AS), 2 mM L-glutamine (Life Technologies), 20 ng/ml basic fibroblast growth factor (BD), 100 U/ml:100 μg/ml penicillin - streptomycin (Life Technologies)) and seeded onto a 25 cm2 tissue culture flask (regarded as passage 0 (P0)). Cells were cultured at +37°C, 5% CO2.
Non adherent cells were removed on the next day by extensive washing with phosphate-buffered saline (PBS) (Life Technologies). The remaining cells were cultivated in the medium supplemented with 10% AS for first 10 days and 5% AS afterwards. When the cells reached 70∼80% confluence they were seeded onto a 75 cm2 tissue culture flask (P1). For the next passages the cells were split in three and seeded onto three 75 cm2 tissue culture flasks after reaching 80∼90% confluence.
The cell culture medium was changed every third day. After removal of the old medium, ASCs were briefly washed with prewarmed PBS (no longer than 30 seconds) before applying the fresh medium.
Alkaline phosphatase activity was tested in the monolayer ASC culture and ASC bodies after the transfer to a new adherent plastic culture flask. The cell samples were fixed in 3.7% formaldehyde (Sigma-Aldrich) for 15 min at +4°C, washed with distilled water and air-dried. To prepare a working solution, a Fast Blue BB salt (Lach-Ner) was dissolved in a stock solution (58.3 mM naphthol AS phosphate (Lach-Ner) dissolved in a dimethyl sulfoxide (Sigma-Aldrich) (final concentration 0.583 mM), 90 mM Tris-HCl (Sigma-Aldrich), pH 9.0∼9.2, 5 mM MgCl2 (Merck Millipore)) to a concentration of 1 mg/ml right before use. Addition of levamisole (Serva) to the working solution at a final concentration of 1 mM served as a negative control. After filtration the naphthol AS phosphate/Fast Blue BB working solution was added to the cells and incubated in dark for 25 min at room temperature followed by washing with distilled water.
ASCs from P2 were grown onto 13 mm uncoated glass coverslips. When adherent ASC bodies started to form, the immunocytochemistry was performed as follows: the coverslips were gently washed with prewarmed PBS, fixed in cold methanol (Sigma-Aldrich) for 10 min at −20°C and 10 times dipped in ice cold acetone. After extensive washing with 1x Tris-buffered saline (TBS), cells were blocked in 1% BSA (Carl Roth)/TBS solution for 15 min at room temperature. Primary antibodies (mouse monoclonal anti-NANOG (Sigma-Aldrich) and anti-OCT4A (C-10) (Santa Cruz Biotechnology)) were diluted in 1% BSA/TBS (1:100 and 1:75 respectively) and incubated overnight at 4°C. In the next day cells were washed with 1x TBS and secondary goat anti-mouse Alexa Fluor 488 antibodies (Life Technologies) diluted in 1x TBS were applied in dark for 40 min at room temperature. The samples were mounted in ProLong Gold Antifade reagent with DAPI (Life Technologies) and analysed after 12 hours using laser scanning confocal system TCS SP2 SE (Leica).
Two days after isolation spindle-shaped plastic-adherent cells were observed. The first ten days ASCs were grown in a medium supplemented with 10% AS but afterwards it was reduced to 5%. When cells with MSC phenotype reached approximately 80% confluence they were firstly seeded onto 75 cm2 tissue culture flask followed by reseeding into three 75 cm2 tissue culture flasks for the next passages. At early passages (P1–P3), above the monolayer of ASCs, floating cell bodies were observed in the uppermost layer of the medium after each change of the fresh medium that also included a brief wash with a PBS. Initially they were detected as faint clumps (Fig. 1A) resembling dead cells. But the ASCs in these clusters continued to divide and grow (Fig. 1B) and started to form compact structures (Fig. 1C). These cell bodies began to congregate into clusters (Fig. 1D), eventually forming large aggregates of interconnected ASC bodies (Fig. 1E, F). Since the medium was changed every third day and the ASC bodies were removed together with the old medium, no more than 72 hours were needed for the formation of observed large aggregates. In each tissue culture flask floating ASC aggregates in different sizes could be observed simultaneously. Both single bodies (Fig. 2A) and smaller or larger aggregates of ASC bodies (Fig. 2B, C) could be seen. Their dense structure and size made it possible to easily detect ASC aggregates even with the naked eye.
When individual floating ASC bodies were transferred onto new adherent plastic tissue culture flasks, they adhered to the surface within 24 hours. Soon after new ASCs started to migrate out of the cell body, ultimately covering the available growth surface and reducing the volume and density of initial ASC body (Fig. 3). Since the size and compactness of original ASC bodies differed, it is not possible to precisely define the time needed for the ASC body to completely disappear forming new cell monolayer after transfer to the new tissue culture flask. Smaller ASC bodies can transform after a week, while bigger ASC bodies can still be observed after a month.
In order to test the influence of medium on above-mentioned process, single floating ASC bodies were transferred onto new adherent plastic tissue culture flasks and cultivated in three different media. In one case the commercial medium MesenPRO RS™ (Life Technologies) containing 2% fetal bovine serum (FBS) was used. For the other two experiments the cell growth medium was applied but autologous serum was substituted with 5% serum from different human donors (donors No.2 and No.3). After 48 hours most of the ASC bodies grown in the medium supplemented with human serum were attached to the surface and gradual expansion of spindle-shaped ASCs around the ASC body could be observed (Fig. 4A and D). In the MesenPRO RS™ medium none of the ASC bodies was adhered to the flask (Fig. 4G). Using commercial medium, four days were needed for few ASC bodies to attach to the surface and plastic-adherent cell growth (Fig. 4H), while in the medium containing human serum every ASC body was attached to the flask and dynamic expansion of cells could be seen (Fig. 4B and E). After 10 days ASC bodies in the medium with 5% human serum were surrounded by extensive cell monolayer (Fig. 4C and F), whereas in the MesenPRO RS™ medium intense migration of ASCs out of the bodies was not observed (Fig. 4I) and few ASC bodies were still floating.
Similar ASC bodies could be observed when ASCs were grown on an uncoated glass surface. In this case ASCs initially formed a monolayer and soon after started to cluster (Fig. 5A). More of the cells were huddled together creating an adherent ASC bodies (Fig. 5B). Eventually most of the ASCs were assembled into dense ASC bodies (Fig. 5C) that could remain adhered to the surface or detach and form floating ASC bodies. But contrary to above-mentioned ASC bodies, this type of floating bodies did not form the aggregates.
The monolayer ASCs culture and ASC bodies after the transfer to new adherent plastic culture flasks were tested for an AP activity using naphthol AS phosphate/Fast Blue BB solution. Separate ASCs in monolayer showed the AP activity detected by two types of blue colouring. Most of the cells, positive for AS, displayed uniform staining (Fig. 6A), while in the others granular staining was observed (Fig. 6B). Addition of levamisole to the naphthol AS phosphate/Fast Blue BB solution served as an evidence for the specificity of reaction, since the levamisole inhibits the AP activity (28) resulting in a lack of blue colouring (Fig. 6C).
When ASC bodies after the transfer to a new adherent plastic culture flasks were tested for an AP activity, the ASCs assembled into bodies did not stain positive for AP irrespective of their size, but large fraction of cells migrating out of the ASC bodies exhibited AP activity detected by blue staining (Fig. 7).
To test whether single ASC bodies expressed such pluripotency markers as NANOG and OCT4, immunocytochemistry with monoclonal anti-NANOG and anti-OCT4A antibodies was employed on glass adherent ASC bodies. The obtained results showed the expression of NANOG in ASC bodies (Fig. 8A–C) and prominent NANOG positive nuclear staining was also observed in clustered ASCs (Fig. 8D–F) where the formation of ASC bodies is initiated. The monolayer ASCs neighbouring ASC bodies were NANOG negative. No positive signal was detected in ASC bodies or monolayer cells using anti-OCT4A antibodies against N-terminus of OCT4 of human origin (data not shown).
In the last few years 3D culture method has become progressively acknowledged as a tool to increase therapeutic properties of adult stem cells (24). Various approaches have been used to obtain somatic stem cell aggregates (27), but in the current study we have shown spontaneous formation of ASC aggregates and their growth properties. Our previous work has demonstrated that obtained cell population is ASCs, based on the expression of MSC surface markers and differentiation ability of these cells into adipocytes, osteocytes and chondrocytes at different passages (29, 30). In this study we observed floating ASC bodies at early passages (P1–P3) in the uppermost layer of a medium that spontaneously assembled after each change of the fresh medium involving a short wash with a prewarmed PBS. What was first detected as small clusters of unattached cells, transformed into large aggregates of joint ASC bodies. We have obtained and propagated ASCs from 10 different human donors, but only in one case have observed the spontaneous formation of herein described ASC bodies. It has been previously shown that adult human mesenchymal cell populations contain a specific type of stem cells, called Muse cells, able to form clusters in suspension culture after subjection to different stress conditions (31). Since donor-to-donor variability in adult stem cell cultures covers diverse aspects of their characteristics (32), it is possible that stress tolerance also varies between donors. Presumably a slight wash of ASCs from this particular donor with PBS was sufficient to trigger the expansion of a distinct subpopulation of stem cells from the individual ASC culture.
When ASCs were seeded onto uncoated glass surface, similar 3D ASC bodies were observed. In this case the ASC bodies formed from the clusters of adherent ASCs and only later detached from the surface. Since MSCs are plastic adherent and their growth and morphological characteristics can be influenced when expanded on the untreated glass surface (33), it is feasible to suspect that reduced cell attachment ability caused the clustering of ASCs and their assembly into bodies.
After a transfer onto new plastic tissue culture flasks spontaneously formed floating ASC bodies, irrespective of their size, were capable to adhere to the surface and gradual spread of spindle-shaped ASCs out of the bodies was observed. This ability of 3D spheroids to convert back to functional monolayer culture has been also described for MSC aggregates obtained by other methods (23). Our results showed that even the replacement of autologous serum in the cell growth medium with allogeneic sera did not impede this process, while commercial medium MesenPRO RS™ delayed the attachment of ASC bodies to the surface and reorganization back to 2D culture (based on a visual assessment). It is known that the addition of FBS to MSC culture medium is essential for the attachment and proliferation of the cells (34). Since the MesenPRO RS™ medium contains only 2% FBS and is adapted to the expansion of monolayer MSCs above clonal densities (according to manufacturer), it may not be suitable for the analysis of growth properties of ASC bodies. However, we have previously shown that FBS can be substituted with AS without the loss of characteristics of ASCs (29), and this study has demonstrated that such culture medium supports the formation of 3D bodies and growth of the new ASC monolayer after the body transfer onto new adherent culture flask.
Few ASCs in monolayer and substantial part of cells migrating out of the ASC bodies after their transfer to a new adherent culture flask displayed AP activity. High activity of AP in human adult can be observed in small intestine, kidney, liver, placenta, bone and neutrophils (35), but in embryo all tissues show strong AP activity at early stages of development (36). Four isozymes of AP are discriminated in the humans: tissue-nonspecific (liver/bone/kidney), intestinal, placental and germ cell (37). The tissue-nonspecific AP (TNAP) expression is a known marker for embryonic stem cells and osteoblast differentiation, but it has also been observed in un-differentiated BM MSCs (38). Authors suggest that TNAP positive (TNAP+) BM MSCs possess lower multi-potentiality than TNAP negative (TNAP−) cells and are prone to differentiate into osteoblasts more often than into adipocytes or chondrocytes. TNAP+ BM MSCs also show lower proliferation rates and diminished expression of pluripotency marker genes NANOG and REX-1 when compared to TNAP- BM MSCs. Since ASCs share most of their characteristics with BM MSCs, it could be possible that ASCs in monolayer, staining positive for AP, represented more mature cells, while the major part of the monolayer ASCs were AP negative, hence illustrating their multipotentiality. As ASC bodies after the transfer onto new adherent surface did not show the AP activity, but significant part of the surrounding cells were AP positive, the ASCs forming 3D bodies may indicate more primitive cells than ASCs migrating out of the bodies.
Similar conclusion can be drawn from the observation that glass adherent ASC bodies as well as clustered ASCs beginning the formation of ASC bodies expressed pluripotency marker NANOG. None of the adjacent monolayer ASC was NANOG positive. We also used anti-OCT4A antibodies to detect isoform A of the transcription factor OCT4 that is responsible for the self-renewal and pluripotency of ESCs (39), but no OCT4A positive ASCs were detected in 3D bodies or ASC monolayer. It has been previously shown that this particular antibody results in a very weak cytoplasmic and nuclear staining in ASCs (40). Although we detected the NANOG positive cells in ASC bodies and clusters of ASCs, this in vitro expression alone does not prove the pluripotency of particular ASCs. Firstly, all three transcriptional regulators OCT4, NANOG and SOX2 are required in order to maintain the pluripotency (11) and the expression of one of them is not sufficient. Secondly, further functional assays must be carried out to show the pluripotent nature of a stem cell population. And thirdly, it has been thought that albeit pluripotency markers can be observed at a basal level in adult stem cells, they may not have the same biological functions as in ESCs (15, 19).
In conclusion, our overall observations suggest that particular stress conditions can cause the spontaneous formation of adherent or floating 3D ASC bodies that may represent more primitive cell subpopulation within the individual ASC culture. While the expression of NANOG in ASC bodies may not be enough to draw similarities with ESCs, the ability to form 3D aggregates, the expression of this pluripotency marker, and the lack of the AP activity may be used to enrich the ASC cultures with potentially more primitive cells.
ACKNOWLEDGMENTS
This work was financially supported by the European Regional Development Fund grant 2010/0230/2DP/2.1.1. 1.0/10/APIA/VIAA/075. We are grateful to “Cilmes Šūnu Tehnoloģijas” Ltd. for providing human ASCs and Dr.habil. med. J. Erenpreisa who kindly provided anti-NANOG antibodies, naphthol AS phosphate and Fast Blue BB salt, as well as consulted on immunocytochemistry method. We thank Dr.habil.med R. Bruvere for performing light microscopy and Dr.biol. D.Pjanova for performing confocal microscopy. We would also like to thank Dr.habil.biol. P. Pumpens and I. Timofejeva.
References
1. Zuk PA, Zhu M, Mizuno H, Huang J, Futrell JW, Katz AJ, Benhaim P, Lorenz HP, Hedrick MH. Multilineage cells from human adipose tissue: implications for cell-based therapies. Tissue Eng. 2001. 7:211–228.


2. Kern S, Eichler H, Stoeve J, Klüter H, Bieback K. Comparative analysis of mesenchymal stem cells from bone marrow, umbilical cord blood, or adipose tissue. Stem Cells. 2006. 24:1294–1301.


3. Fraser JK, Wulur I, Alfonso Z, Hedrick MH. Fat tissue: an underappreciated source of stem cells for biotechnology. Trends Biotechnol. 2006. 24:150–154.


4. Zuk PA, Zhu M, Ashjian P, De Ugarte DA, Huang JI, Mizuno H, Alfonso ZC, Fraser JK, Benhaim P, Hedrick MH. Human adipose tissue is a source of multipotent stem cells. Mol Biol Cell. 2002. 13:4279–4295.


5. Planat-Bénard V, Menard C, André M, Puceat M, Perez A, Garcia-Verdugo JM, Pénicaud L, Casteilla L. Spontaneous cardiomyocyte differentiation from adipose tissue stroma cells. Circ Res. 2004. 94:223–229.


6. Jang S, Cho HH, Cho YB, Park JS, Jeong HS. Functional neural differentiation of human adipose tissue-derived stem cells using bFGF and forskolin. BMC Cell Biol. 2010. 11:25.


7. Cao Y, Sun Z, Liao L, Meng Y, Han Q, Zhao RC. Human adipose tissue-derived stem cells differentiate into endothelial cells in vitro and improve postnatal neovascularization in vivo. Biochem Biophys Res Commun. 2005. 332:370–379.


8. Taléns-Visconti R, Bonora A, Jover R, Mirabet V, Carbonell F, Castell JV, Gómez-Lechón MJ. Hepatogenic differentiation of human mesenchymal stem cells from adipose tissue in comparison with bone marrow mesenchymal stem cells. World J Gastroenterol. 2006. 12:5834–5845.


9. Chandra V, Swetha G, Muthyala S, Jaiswal AK, Bellare JR, Nair PD, Bhonde RR. Islet-like cell aggregates generated from human adipose tissue derived stem cells ameliorate experimental diabetes in mice. PLoS One. 2011. 6:e20615.


10. Boyer LA, Lee TI, Cole MF, Johnstone SE, Levine SS, Zucker JP, Guenther MG, Kumar RM, Murray HL, Jenner RG, Gifford DK, Melton DA, Jaenisch R, Young RA. Core transcriptional regulatory circuitry in human embryonic stem cells. Cell. 2005. 122:947–956.


11. Pan G, Thomson JA. Nanog and transcriptional networks in embryonic stem cell pluripotency. Cell Res. 2007. 17:42–49.


12. Tai MH, Chang CC, Kiupel M, Webster JD, Olson LK, Trosko JE. Oct4 expression in adult human stem cells: evidence in support of the stem cell theory of carcinogenesis. Carcinogenesis. 2005. 26:495–502.


13. Izadpanah R, Trygg C, Patel B, Kriedt C, Dufour J, Gimble JM, Bunnell BA. Biologic properties of mesenchymal stem cells derived from bone marrow and adipose tissue. J Cell Biochem. 2006. 99:1285–1297.


14. Greco SJ, Liu K, Rameshwar P. Functional similarities among genes regulated by OCT4 in human mesenchymal and embryonic stem cells. Stem Cells. 2007. 25:3143–3154.


15. Lengner CJ, Camargo FD, Hochedlinger K, Welstead GG, Zaidi S, Gokhale S, Scholer HR, Tomilin A, Jaenisch R. Oct4 expression is not required for mouse somatic stem cell self-renewal. Cell Stem Cell. 2007. 1:403–415.


16. Atlasi Y, Mowla SJ, Ziaee SA, Gokhale PJ, Andrews PW. OCT4 spliced variants are differentially expressed in human pluripotent and nonpluripotent cells. Stem Cells. 2008. 26:3068–3074.


18. Liedtke S, Enczmann J, Waclawczyk S, Wernet P, Kögler G. Oct4 and its pseudogenes confuse stem cell research. Cell Stem Cell. 2007. 1:364–366.


19. Lengner CJ, Welstead GG, Jaenisch R. The pluripotency regulator Oct4: a role in somatic stem cells? Cell Cycle. 2008. 7:725–728.


20. Kurosawa H. Methods for inducing embryoid body formation: in vitro differentiation system of embryonic stem cells. J Biosci Bioeng. 2007. 103:389–398.


21. Jensen JB, Parmar M. Strengths and limitations of the neurosphere culture system. Mol Neurobiol. 2006. 34:153–161.


22. Ivascu A, Kubbies M. Rapid generation of single-tumor spheroids for high-throughput cell function and toxicity analysis. J Biomol Screen. 2006. 11:922–932.


23. Bartosh TJ, Ylöstalo JH, Mohammadipoor A, Bazhanov N, Coble K, Claypool K, Lee RH, Choi H, Prockop DJ. Aggregation of human mesenchymal stromal cells (MSCs) into 3D spheroids enhances their antiinflammatory properties. Proc Natl Acad Sci U S A. 2010. 107:13724–13729.


24. Frith JE, Thomson B, Genever PG. Dynamic three-dimensional culture methods enhance mesenchymal stem cell properties and increase therapeutic potential. Tissue Eng Part C Methods. 2010. 16:735–749.


25. Baraniak PR, McDevitt TC. Scaffold-free culture of mesenchymal stem cell spheroids in suspension preserves multi-lineage potential. Cell Tissue Res. 2012. 347:701–711.


26. Cerwinka WH, Sharp SM, Boyan BD, Zhau HE, Chung LWK, Yates C. Differentiation of human mesenchymal stem cell spheroids under microgravity conditions. Cell Regen. 2012. 1:2.


27. Sart S, Tsai AC, Li Y, Ma T. Three-dimensional aggregates of mesenchymal stem cells: cellular mechanisms, biological properties, and applications. Tissue Eng Part B Rev. 2014. 20:365–380.


28. Van Belle H. Alkaline phosphatase. I. Kinetics and inhibition by levamisole of purified isoenzymes from humans. Clin Chem. 1976. 22:972–976.


29. Bogdanova A, Berzins U, Bruvere R, Eivazova G, Kozlovska T. Adipose-derived stem cells cultured in autologous serum maintain the characteristics of mesenchymal stem cells. Proc Latv Acad Sci Sect B Nat Exact Appl Sci. 2010. 64:106–113.


30. Bogdanova A, Berzins U, Nikulshin S, Skrastina D, Ezerta A, Legzdina D, Kozlovska T. Characterization of human adipose-derived stem cells cultured in autologous serum after subsequent passaging and long term cryopreservation. J Stem Cells. 2014. 9:135–148.
31. Kuroda Y, Kitada M, Wakao S, Nishikawa K, Tanimura Y, Makinoshima H, Goda M, Akashi H, Inutsuka A, Niwa A, Shigemoto T, Nabeshima Y, Nakahata T, Nabeshima Y, Fujiyoshi Y, Dezawa M. Unique multipotent cells in adult human mesenchymal cell populations. Proc Natl Acad Sci U S A. 2010. 107:8639–8643.


32. Siegel G, Kluba T, Hermanutz-Klein U, Bieback K, Northoff H, Schäfer R. Phenotype, donor age and gender affect function of human bone marrow-derived mesenchymal stromal cells. BMC Med. 2013. 11:146.


33. Ho M, Yu D, Davidsion MC, Silva GA. Comparison of standard surface chemistries for culturing mesenchymal stem cells prior to neural differentiation. Biomaterials. 2006. 27:4333–4339.


34. Lennon DP, Haynesworth SE, Bruder SP, Jaiswal N, Caplan AI. Human and animal mesenchymal progenitor cell from bone marrow: identification of serum for optimal selection and proliferation. In Vitro Cell Dev Biol - Animal. 1996. 32:602–611.


35. Butterworth PJ. Alkaline phosphatase. Biochemistry of mammalian alkaline phosphatases. Cell Biochem Funct. 1983. 1:66–70.
36. Berstine EG, Hooper ML, Grandchamp S, Ephrussi B. Alkaline phosphatase activity in mouse teratoma. Proc Natl Acad Sci U S A. 1973. 70:3899–3903.


37. Millán JL. Alkaline Phosphatases : Structure, substrate specificity and functional relatedness to other members of a large superfamily of enzymes. Purinergic Signal. 2006. 2:335–341.
38. Kim YH, Yoon DS, Kim HO, Lee JW. Characterization of different subpopulations from bone marrow-derived mesenchymal stromal cells by alkaline phosphatase expression. Stem Cells Dev. 2012. 21:2958–2968.


Fig. 1.
Formation of floating aggregates of ASC bodies. (A∼F) Different stages of development of cell aggregates at P1. Scale bar 100 μm (50 μm for Fig. 1B).
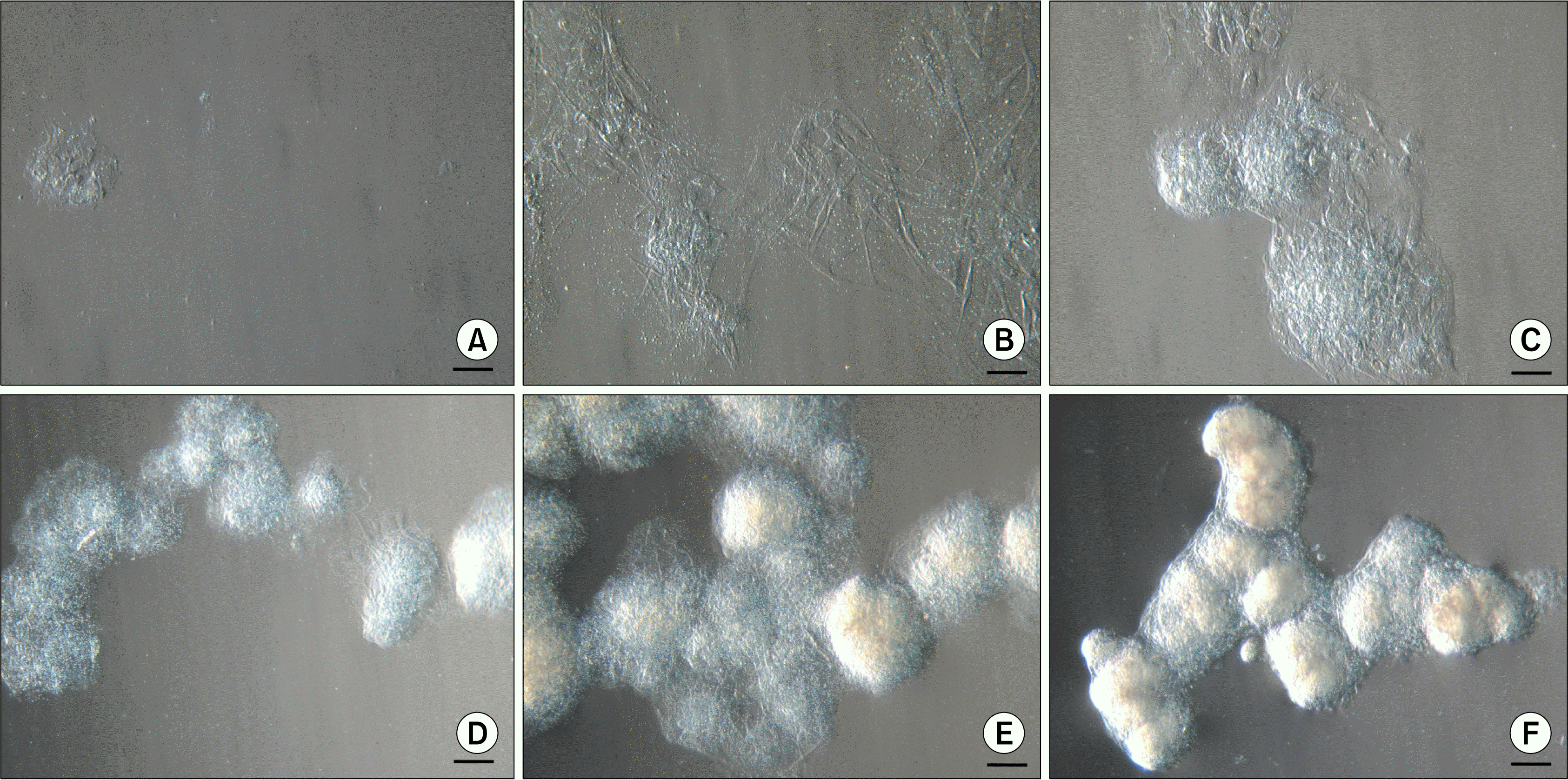
Fig. 2.
Types of floating ASC bodies. (A) Single ASC body. (B) Small aggregate of ASC bodies. (C) Large aggregate of ASC bodies. Scale bar 100 μm (50 μm for Fig. 2A).
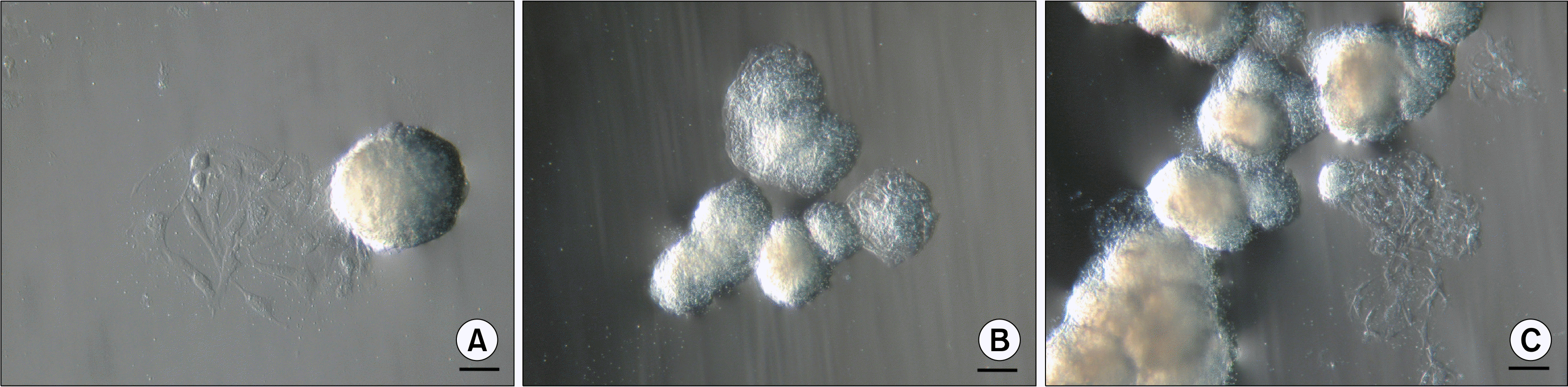
Fig. 3.
Cell growth after the transfer of single ASC bodies onto adherent cell culture plates. (A) ASC body after 3 days. (B) ASC body after 10 days. (C) ASC body after 30 days. Scale bar 100 μm.
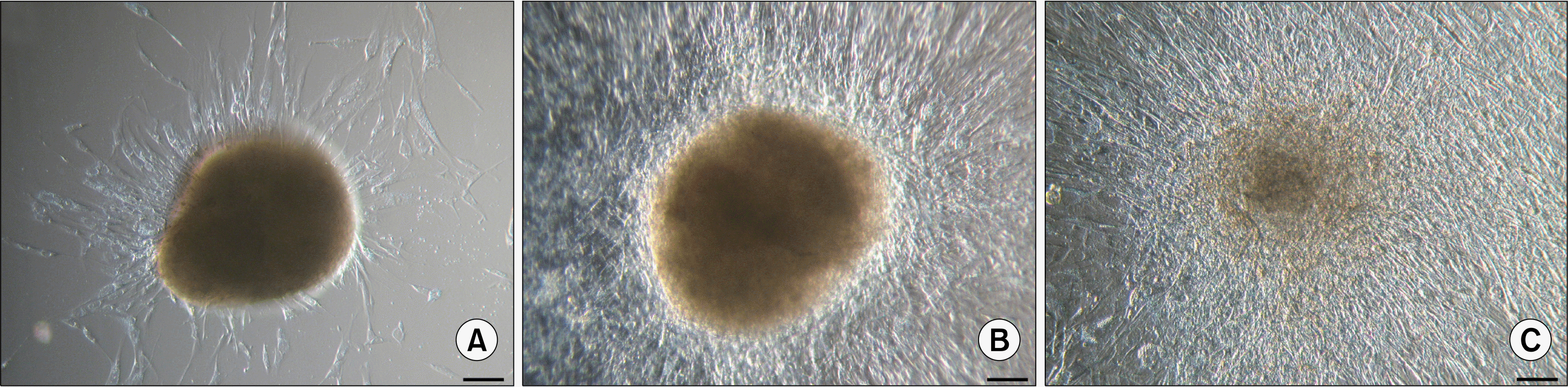
Fig. 4.
Cell growth in the different cell culture media after the transfer of ASC bodies onto adherent cell culture plates. (A∼C) ASC body in the medium supplemented with 5% serum from donor No.2. (D∼F) ASC body in the medium supplemented with 5% serum from donor No.3. (G∼I) ASC body in the commercial medium MesenPRO RS™. Scale bar 100 μm.

Fig. 5.
Formation of adherent ASC bodies onto a glass surface. (A) ASCs in a monolayer start to gather into clusters (day 2). (B) As more ASCs are congregated in the clusters, ASC bodies are formed (day 5). (C) Eventually most of the monolayer ASCs are clustered into dense bodies (day 8). Scale bar 100 μm.
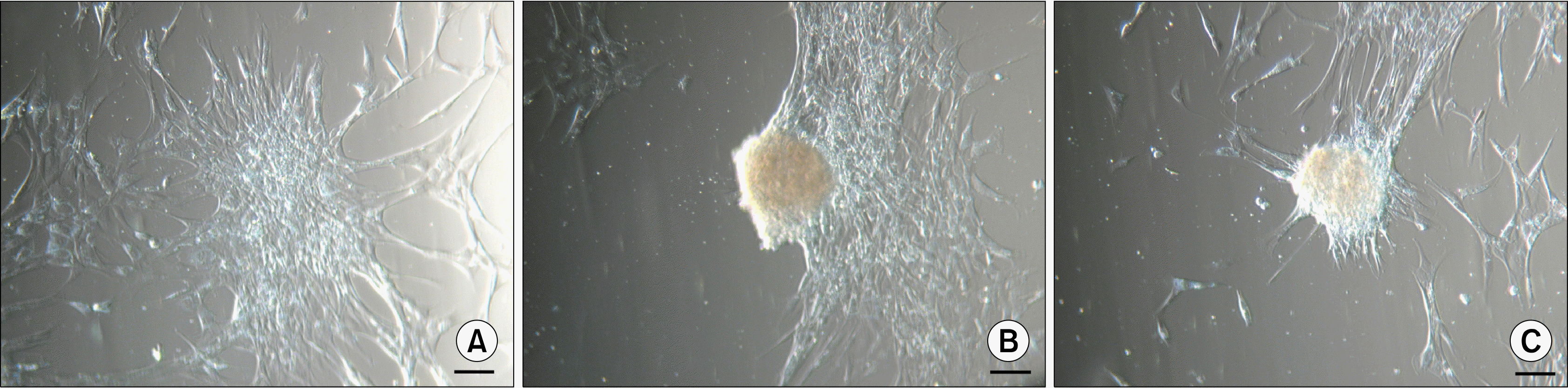
Fig. 6.
Detection of the alkaline phosphatase activity in ASCs at P3 using naphthol AS phosphate/Fast Blue BB solution. (A, B) ASCs positive for alkaline phosphatase activity (blue) demonstrating uniform and granular staining. (C) Inhibition of alkaline phosphatase activity by levamisole. Scale bar 50 μm.
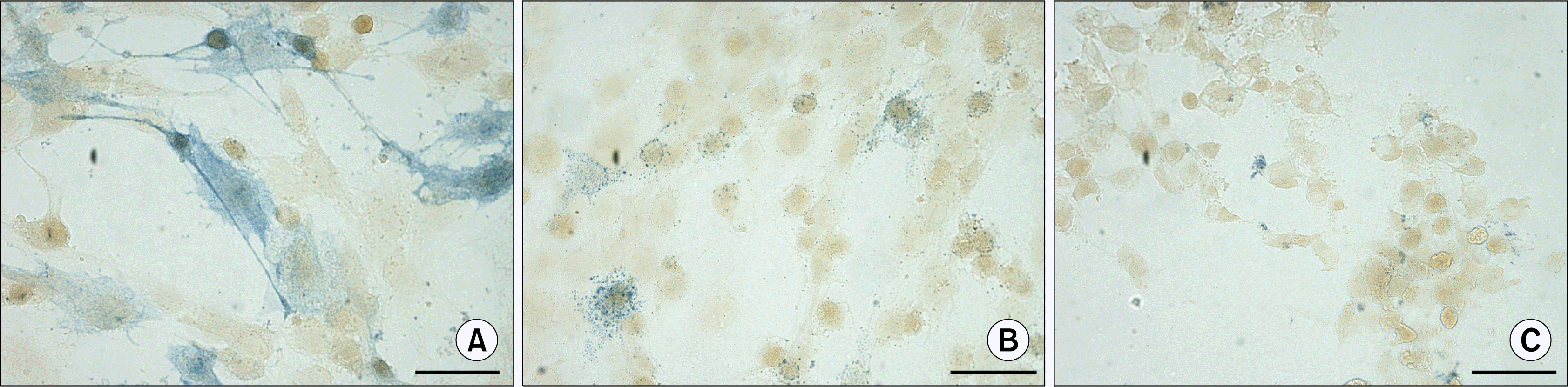
Fig. 7.
Detection of the alkaline phosphatase activity in ASC bodies 9 days after the transfer onto adherent cell culture plates using naphthol AS phosphate/Fast Blue BB solution. (A) Absence of AP activity in large ASC body. (B) Lack of AP activity in small ASC body and evident AP activity (blue) in the cells surrounding the ASC body. (C) AP activity (blue) in the cells migrating out of the ASC body. Scale bar 100 μm (50 μm for Fig. 7C).
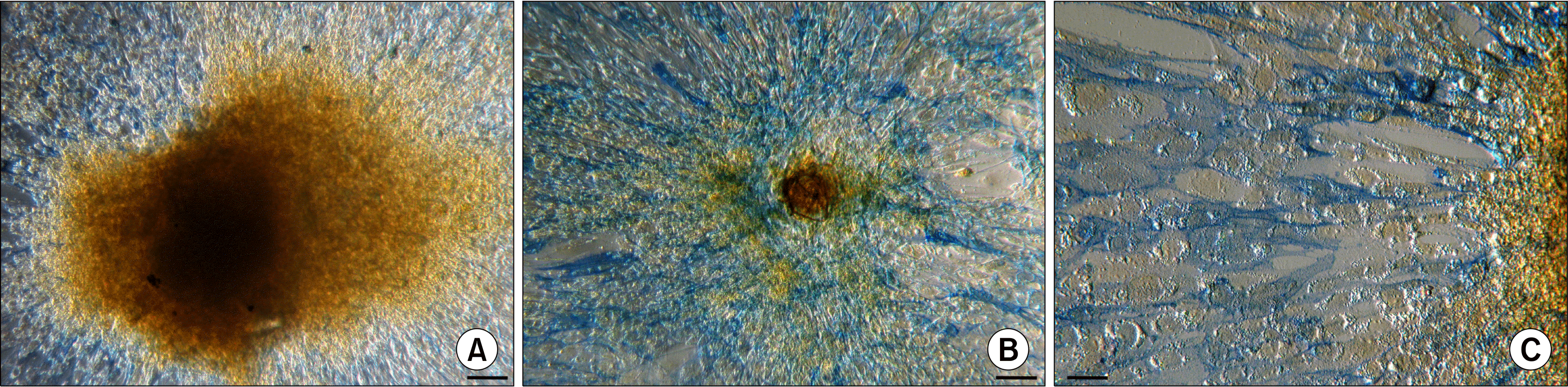