Abstract
Background and Objectives:
Stem cell technology offers a new hope for many chronic disorders patients. The types of stem cells are different with many differences existing between each type. Mesenchymal stem cells (MSCs) represent one type of adult stem cells that can be easily isolated, then re-transplanted to the patients. This offers potential for their future application in treating many disorders without fear of rejection possibility. MSCs can be isolated from different sources e.g. bone marrow (BMSCs) and adipose tissue (ADSCs). In the present study we compared BMSCs and ADSCs isolated from Sprague-Dawley rats.
Methods and Results:
For this comparison, immunophenotyping, the analysis of growth rates, proliferation by colony forming unit-fibroblast assay, population doubling time, and trilineage differentiation assays were performed for both BMSCs and ADSCs. The findings revealed that despite no difference in immunphenotypic character between BMSC and ADSC, a better proliferative capacity was observed for ADSCs which would advocate their better use in regenerative applications. On the other hand, BMSCs showed more potential for osteogenic and chondrogenic differentiation.
Conclusions:
Our study showed that, despite many similarities between both types of cells, there are differences existing which can offer assistance on choosing type of cell to be used in specific diseases. Although ADSCs seem more promising for regenerative application generally, BMSCs may represent a better choice for treating bone disorders.
There is no universally acceptable definition of the term “Stem cell”. Many efforts have been made for defining certain characters which can differentiate stem cells from other cells encountered either in vivo or in cell cultures (1). One of the definitions accepted by many authors is that stem cells are unspecialized cells in the human body that are capable of becoming specialized cells, each with new specialized cell functions (2). The unique capabilities of stem cells for pluripotency and long-term self-renewal make them ideal source for the regeneration of injured tissue. Despite the numerous obstacles, the potential for cell-based therapy in the treatment of degenerative diseases of all organ systems, provides an exciting arena for continued research (3, 4). The multiple applications of stem cells; not only for regeneration but also in predictive toxicology, drug discovery and drug delivery studies denote the importance of the field of stem cell technology (5, 6).
Mesenchymal stem cells (MSCs) are adult stem cells that possess self-renewal and multi-lineage differentiation potentials, and considered an attractive source of stem cells for tissue engineering (7–10). Previous pre-clinical studies have shown that MSCs from different sources, including human bone marrow (BMSCs), cord blood, embryo and fetal membranes can be applied in tissue repair, such as promoting recovery from acute kidney injury (AKI) induced by various causes (11, 12). Nonetheless, do the different sources of mesenchymal stem cells offer different characters of these cells? Although previous researches tried to answer this question, inconsistent findings were reported (13–17). In the current study we will compare in vitro characteristics of bone marrow mesenchymal stem cells (BMSCs) and adipose tissue mesenchymal stem cells (ADSCs) obtained from Sprague-Dawley rats.
Isolation of MSCs from bone marrow (BM) was performed as previously described (18) with some modifications. Eight-week-old male Sprague-Dawley rats were sacrificed by cervical dislocation and their femurs and tibiae were carefully cleaned from skin by pulling toward the foot, which is cut at the ankle bone. The muscle and connective tissue were removed from both the tibia and the femur by scraping the diaphysis of the bone clean then pulling the tissue toward the ends of the bone. The bones were put in ethyl alcohol 10% for sterilization and leave it some seconds. The ends of the tibia and femur were cut by sharp Scissors. A 27-gauge needle were Inserted and flushed with Dulbecco’s Modified Eagle’s Medium DMEM and collect in a 15-ml tube. The cell suspension was filtered through a 70-μm filter mesh. BM cells were cultured in DMEM+10% FBS+1% antibiotic-antimycotic solution (Thermo scientific, USA) in 25 cm2 tissue culture flask and incubated at 37°C with 5% CO2.
Isolation of MSCs from adipose tissue was performed as previously described (19) with some modifications. The inguinal fat pad was collected under sterile conditions from eight-week-old male Sprague-Dawley rats and washed with phosphate-buffered saline (PBS) containing 1% antibiotic-antimycotic solution (Thermo scientific, USA). The washing step was repeated until all blood vessels and connective tissues appeared to have been liberated (usually 3 washes).
Adipose tissue sample (3ml for each 1 gm of tissue) was minced into small pieces and digested in 0.1% Collagenase Type 1 (GIBCO, cat. no. 17100) at 37°C with shaking for 2 hr. After digestion, an equal volume of DMEM containing 10% fetal bovine serum (Thermo scientific, USA) was added. For further disintegration of tissue aggregates, the sample was pipetted up and down several times. The cell suspension was filtered through 100 μm filter (BD Falcon, USA) for the removal of the solid aggregates. The sample was subsequently centrifuged at 2000 rpm for 5 min at room temperature and vigorously mixed to complete the separation of the stromal cells from the adipocytes. The centrifugation step was repeated, and the supernatant removed without disturbing the cells. The pellet was re-suspended in 1 ml of lysis buffer (Promega, Germany) to lyse Red Blood Cells, incubated for 10 min, washed with 10 ml of PBS+1% antibiotic-antimycotic mixture and centrifuged at 2000 rpm for 5 min. Supernatant was removed and the cell pellet was re-suspended in complete medium (DMEM with 20% fetal bovine serum (FBS) and 1% antibiotic antimycotic solution) in 25 cm2 culture flask and maintained in an incubator supplied with humidified atmosphere of 5% CO2 at 37°C.
After 1 day, non-adherent cells were removed by two to three washes with PBS and adherent cells further cultured in complete medium. The medium was changed every 3 days until the monolayer of adherent cells reached 70∼80% confluence. Cell passaging was performed using trypsin-EDTA solution (0.25%, Sigma Aldrich, USA). The number of recovered cells evaluated with the use of hemocytometer and cellular viability was quantified by the Trypan Blue exclusion test. Approximatly 250–300×103 cells were used to inoculate 75 cm2 culture flask and cultures were incubated at 37°C and 5% CO2. Cell cultivation was performed up to the 3rd passage.
Cells were characterized using cell surface markers by fluorescence-activated cell sorting (FACS) analysis. The cells were stained with different fluorescently labeled monoclonal antibodies (mAb) (eBioscience, company). In brief, 5×105 cells (in 100 μl PBS/0.5% BSA/2 mmol/EDTA) were mixed with 10 μl of the fluorescently labeled mAb (anti-rat CD45-FITC mAb, CD29-PE mAb and CD 90-PCY5) and incubated in the dark at 2∼8°C for 30 min. Washing with PBS containing 2% BSA was done twice and the pellet was re-suspended in PBS and analyzed immediately on EPICS-XL flow cytometer (Coulter, Miami, Fl, USA).
For colony forming unit-fibroblast (CFU-F) assays, about 100 passage-3 cells were plated in 100-mm tissue culture dish (Falcon) in complete culture medium. Cells were maintained in complete medium for 10∼14 d at 37°C in 5% humidified CO2, wish half-weekly media changes. On day 14, cells were washed with PBS and fixed in 95% ethanol for 5 minutes, subsequently, the cells were incubated for 30 minutes at room temperature in 0.5% crystal violet solution (Sigma Aldrich, USA) in 95% ethanol. Then the plates were washed twice with distilled H2O, dried and the resulting CFU-F colonies were counted.
PDT is the time by which cell population doubles in number. Population doubling time calculated according to the equation PDT=culture time (CT)/PDN. Population doubling number (PDN) was calculated according to the formulae PDN=log N/N0×3.31 where N is the cell number at the end of the cultivation period, N0 the cell number at culture initiation. Cells were plated 104 cells/cm2 in 25 cm2 culture flasks until reaching confluence. Susequently cells were trypsinized and counted; this information is used to calculate PDT.
To plot the growth curve, passage-3 cells were plated in 12-well plate at the seeding density of 5×104 cells/well and allowed to become confluent. Some wells were trypsinized daily and counted. The growth curve was then plotted using the cell counting data.
Passage 3 MSCs were harvested by trypsin digestion as described above; the cells were counted and seeded at a density of 5×104 per well in a 6-well plate. When∼80% confluent, osteogenesis differentiation media (DMEM supplemented with 10% FBS, 0.1 μM dexamethasone, 50 μM Ascorbic acid, 10 mM β-glycerol phosphate (Sigma-Aldrich, USA) was added to 4 wells whereas complete culture Media was added to other 2 wells as negative controls. The medium was changed twice per week for 2∼3 weeks. The differentiation potential for osteogenesis was assessed by 40 mM Alizarin Red (pH 4.1) staining after fixation in 10% Neutral buffered formalin.
Passage 3 MSCs were counted and seeded at a density of (10×104 per well) in a 6-well plate. When ∼100% confluent, adipogenesis differentiation media DMEM supplemented with 10% FBS, 1 μM dexamethasone, 500 μM isobutylmethylxanthine (IBMX) 5 μg/ml insulin, 200 μM Indomethacin (Sigma-Aldrich, USA) was added to 4 well-sand complete culture Media was added to other 2 well as negative controls. The medium was changed twice per week for 2 weeks. The differentiation potential for adipogenesis and formation of intracellular lipid droplets were assessed by Oil-red-O staining after fixation in 10% Neutral buffered formalin.
Both osteogenic and adipogenic differentiation was analysed digitally: 20 different digital images corresponding to 4 different preparations of differentiated cells and undifferentiated controls were analyzed using ImageJ 1.42 software (U.S. National Institutes of Health http://rsb.Info.nih.gov/ij/) on an appropriate threshold.
Passage 3 MSCs were counted and seeded (at a density of 0.25×106 per Eppendorf tube) in chondrogenic differentiation medium, which consists of high glucose DMEM supplemented with 10 ng/ml TGF-β3, 100n M dexamethasone, 200 μM ascorbate 2 phosphate, 40 μg/ml proline, 1 mM pyruvate, 1 mg/ml BSA and 50 mg/ml ITS +3. The medium was replaced every 2∼3 days for 21 days. Production of sulfated glycosaminoglycans was measured in an Alcian blue binding assay (Immunodiagnostic Systems, Boldon, UK) following digestion in 100 μl of pa-pain solution. Absorbance was read at 630 nm, as previously described (20).
The cultures were observed by using an inverted light microscope. Attachment of spindle-shaped cells to tissue culture plastic flask was observed after 1 day of culture of both BMSCs and ADSCs. Primary cultures reached 70∼80% confluence in approximately 7∼9 days for BMSCs and 5∼6 days for ADSCs. During the passaging, the cell growth tended to accelerate and morphology of cells changed gradually. Cells become more flat-shape with increasing in passage number. After three passages (Fig. 1) both cultures were represented a homogenously fibroblastic cell monolayer. ADSCs showed ability to form multilayer after confluence (Fig. 1E).
Cultures of third passage BMSCs and ADSCs were analyzed for expression of MSC specific cell-surface markers (21). BMSCs and ADSCs were negative for the hematopoietic lineage marker CD45 with average percentages of 1.75±0.23 and 1.59±0.18, respectively. BMSCs and ADSCs were positive for CD29 with average percentages of 98.2±0.41 and 99.7±0.25, respectively. BMSCs and ADSCs were positive for CD90 with average percentages of 93.5±0.5 and 91±0.3, respectively (Table 1).
CFU-F assay is a suitable tool for evaluating the proliferation and colonogenic capacity of the cells (3th passage) expanded in culture. The colony number of 100 BMSC and ADSCs per 100-mm tissue culture dish was 3±1.8 and 37±1.4 respectively (p<0.05). The difference was statistically significant (Fig. 2A).
The PDT value for ADSCs appeared to be significantly lower than that for the BMSCs indicating the more rapid rate of proliferation of ADSCs. Based on the results, ADSCs tended to double their population in the average of 39.88±4.4 hours while those from BMSCs exhibited a doubling time of 49.9±4.2 hours (p<0.05). (Fig. 2B).
According to the plotted curve, both BMSCs and ADSCs had no lag phase and started proliferating immediately after being plated. ADSCs had a better proliferation ability than BMSCs. ADSCs unlike BMSCs showed low contact inhibition (Fig. 3C).
BMSCs and ADSCs differentiated into osteoblastic line-age (Fig. 3A and B). Image J analysis of differentiated vs. undifferentiated cells revealed 4.2±0.8 fold increase for and BMSCs 3.4±0.5 fold increase for ADSCs suggesting BMSCs appeared to have a better ability for osteogenic differentiation.
BMSCs and ADSCs differentiated into adipocyte line-age (Fig. 3C and D). Image J analysis of differentiated vs. undifferentiated cells revealed 1.2±0.5 fold increase for BMSCs 2.6±0.7 fold increase for ADSCs. Suggesting ADSCs appeared to have a better ability for adipogenic differentiation.
Both BMSCs and ADSCs differentiated into chondrocytes but BMSCs had more ability to produce sulfated GAG than ADSCs. Concentration of sulfated GAG in BMSC-derived pellets was is 15.95 μg/ml (versus negative control of 3.29 μg/ml). Concentration of sulfated GAG in ADSC-derived pellets was 11.66 μg/ml (versus negative control of 0.99 μg/ml) (Fig. 3E).
This study provides some insights on the differences existing between BMSCs and ADSCs regarding their basic biological properties in vitro. Using such data, investigators will be able to select the right cells for their experimental, preclinical and clinical research.
In the present study, we compared BMSCs with ADSCs from the same species, age and sex (eight-week-old male Sprague-Dawley rat). Young male rats were chosen because experimentally, mesenchymal stem cells were found in larger number in young more than adult and in males more than females (22, 23).
Stromal vascular fraction (SVF) was cultured, like bone marrow cells, in DMEM with FBS in plastic flasks. Media of both bone marrow cells and SVF of adipose tissue were changed after 24 hours to get rid of non adherent cells and leave adherent cells which are mostly MSCs. The morphological changes of cells have been noted every day, cells gave spindle shape after about one day and we noticed that cells become more flat-shaped with increasing in passage number. These findings are consistent with the results of Nadri and Soleimani, but their study was on C57BL/6 mouse bone marrow (18). No significant morphologic differences were observed between BMSCs and ADSCs, but BMSCs took longer time than ADSCs to reach 70∼80% confluence and ADSCs had ability to form multilayer after reaching confluence; this was likely due to low contact inhibition consistent with results of Zhu et al. (24).
Immunophenotyping characterization was performed by flow cytometry. Flow cytometry measurements showed no significant differences between BMSCs and ADSCs, that were both positive for CD29, CD90 and negative for CD45, and these results are consistent with previously published studies (22, 25). Colony forming unit-fibroblast (CFU-F) assays were performed to measure the ability of cells for proliferation and clonogenic capacity. Both cell types had an ability to proliferate and form colonies of fibroblasts but ADSCs had better such abilities compared to BMSCs; these results are consistent with findings of Peng et al. (26).
Population doubling time (PDT) were performed for measuring the time that cells took for doubling their number and BMSCs took longer time for doubling than ADSCs. Growth curve analysis was performed to measure proliferation of cells and phases of their growth throughout 8 days. Both BMSCs and ADSCs had no lag phase and started proliferating immediately after being plated but ADSCs had more proliferation ability than BMSCs. ADSCs unlike BMSCs showed low contact inhibition that appeared in the plateau phase. Differentiation capability of BMSCs and ADSCs was tested by placing each cell type cultured in differentiation-induction media compared to control media. Both BMSCs and ADSCs had capability to differentiation to osteoblasts, adipocytes and chondrocytes, however BMSCs appeared to possess better osteogenic and chondrogenic properties compared to ADSCs. On the contrary, in case of adipocyte differentiation, ADSCs appeared to have a better differentiation capability assessed by the amount of fat vacuoles formed in adipocyte differentiated cells. The amount of fat vacuoles in ADSCs adipocyte differentiated cells was higher than that in BMSCs adipocyte differentiated cells and these result are consistent with work of Yoshimura et al. (27) and in contrast to results of Rebelatto et al. (28).
Our results show that both types of cells - BMSCs and ADSCs - have ability for proliferation and the clonogenic capacity. Moreover, we showed that ADSCs are expanding more rapidly than BMSCs. We predict from this in vitro study that ADSCs will be more easily grown than BMSCs in pre-clinical and clinical application. In addition, ADSCs are harvested from patient by a simple and minimally invasive method. This in contrast to Im et al. who casted doubts on the value of adipose tissue as a source of MSCs due to their lower ability for osteogenic differentiation compared with BMSCs (29). On the other hand, our results confirm the findings of Im et al. suggesting that BMSCs may be more efficient than ADSCs in treating bone disease (29).
The present study compared ADSCs with BMSCs as two different sources for MSCs. Our study showed that, despite many similarities between both types of cells, there are differences existing which can offer assistance on choosing type of cell to be used in specific diseases. Although ADSCs seem more promising for regenerative application generally, BMSCs may represent a better choice for treating bone disorders.
ACKNOWLEDGMENTS
The authors thank Dr.Yasser El Sherbiny for his efforts in the establishment of this collaboration. We are grateful to Dr.Thomas Baboolal and Dr. Richard Cuthbert for their help in stem cells characterization.
This work was supported by Medical Experimental Research Center (MERC), Faculty of Medicine, Mansoura University and Leeds Institute of Rheumatic and Musculoskeletal Medicine, University of Leeds, Leeds, UK.
References
1. Bongso A, Fong C. Human embryonic stem cells: their nature, properties, and uses. Baharvand H, editor. Trends in stem cell biology and technology. New York, NY: Humana Press;2009. 1–18.


2. D’Inverno M, Theise N, Prophet J. Mathematical modeling of stem cells: A complexity primer for the stem-cell biologist. Potten CS, Clarke RB, Wilson J, Renehan AG, editors. Tissue stem cells. New York: Taylor & Francis Group;2006. 1–5.
3. Nagy RD, Tsai BM, Wang M, Markel TA, Brown JW, Meldrum DR. Stem cell transplantation as a therapeutic approach to organ failure. J Surg Res. 2005. 129:152–160.
4. Satija NK, Singh VK, Verma YK, Gupta P, Sharma S, Afrin F, Sharma M, Sharma P, Tripathi RP, Gurudutta GU. Mesenchymal stem cell-based therapy: a new paradigm in regenerative medicine. J Cell Mol Med. 2009. 13:4385–4402.


5. Yang KL, Shyr MH. Are Stem Cells the Magical Medical Therapy of the Future? Tzu Chi Med J. 2009. 21:12–17.


6. Liu W, Deng Y, Liu Y, Gong W, Deng W. Stem cell models for drug discovery and toxicology studies. J Biochem Mol Toxicol. 2013. 27:17–27.


7. Jiang Y, Jahagirdar BN, Reinhardt RL, Schwartz RE, Keene CD, Ortiz-Gonzalez XR, Reyes M, Lenvik T, Lund T, Blackstad M, Du J, Aldrich S, Lisberg A, Low WC, Largaespada DA, Verfaillie CM. Pluripotency of mesenchymal stem cells derived from adult marrow. Nature. 2002. 418:41–49.


8. Caplan AI. Adult mesenchymal stem cells for tissue engineering versus regenerative medicine. J Cell Physiol. 2007. 213:341–347.


9. Tae SK, Lee SH, Park JS, Im GI. Mesenchymal stem cells for tissue engineering and regenerative medicine. Biomed Mater. 2006. 1:63–71.


10. Strioga M, Viswanathan S, Darinskas A, Slaby O, Michalek J. Same or not the same? Comparison of adipose tissue-derived versus bone marrow-derived mesenchymal stem and stromal cells. Stem Cells Dev. 2012. 21:2724–2752.


11. Bruno S, Bussolati B. Therapeutic effects of mesenchymal stem cells on renal ischemia-reperfusion injury: a matter of genetic transfer? Stem Cell Res Ther. 2013. 4:55.


12. Mobasheri A, Csaki C, Clutterbuck AL, Rahmanzadeh M, Shakibaei M. Mesenchymal stem cells in connective tissue engineering and regenerative medicine: applications in cartilage repair and osteoarthritis therapy. Histol Histopathol. 2009. 24:347–366.
13. Reich CM, Raabe O, Wenisch S, Bridger PS, Kramer M, Arnhold S. Isolation, culture and chondrogenic differentiation of canine adipose tissue- and bone marrow-derived mesenchymal stem cells--a comparative study. Vet Res Commun. 2012. 36:139–148.


14. Kisiel AH, McDuffee LA, Masaoud E, Bailey TR, Esparza Gonzalez BP, Nino-Fong R. Isolation, characterization, and in vitro proliferation of canine mesenchymal stem cells derived from bone marrow, adipose tissue, muscle, and periosteum. Am J Vet Res. 2012. 73:1305–1317.


15. Nakanishi C, Nagaya N, Ohnishi S, Yamahara K, Takabatake S, Konno T, Hayashi K, Kawashiri MA, Tsubokawa T, Yamagishi M. Gene and protein expression analysis of mesenchymal stem cells derived from rat adipose tissue and bone marrow. Circ J. 2011. 75:2260–2268.


16. Zhang W, Zhang X, Wang S, Xu L, Zhang M, Wang G, Jin Y, Zhang X, Jiang X. Comparison of the use of adipose tissue-derived and bone marrow-derived stem cells for rapid bone regeneration. J Dent Res. 2013. 92:1136–1141.


17. Melief SM, Zwaginga JJ, Fibbe WE, Roelofs H. Adipose tissue-derived multipotent stromal cells have a higher immunomodulatory capacity than their bone marrow-derived counterparts. Stem Cells Transl Med. 2013. 2:455–463.


18. Nadri S, Soleimani M. Isolation murine mesenchymal stem cells by positive selection. In Vitro Cell Dev Biol Anim. 2007. 43:276–282.


19. Bunnell BA, Flaat M, Gagliardi C, Patel B, Ripoll C. Adipose-derived stem cells: isolation, expansion and differentiation. Methods. 2008. 45:115–120.


20. Jones EA, English A, Henshaw K, Kinsey SE, Markham AF, Emery P, McGonagle D. Enumeration and phenotypic characterization of synovial fluid multipotential mesenchymal progenitor cells in inflammatory and degenerative arthritis. Arthritis Rheum. 2004. 50:817–827.


21. Boxall SA, Jones E. Markers for characterization of bone marrow multipotential stromal cells. Stem Cells International. 2012. 2012:975871.


22. Asumda FZ, Chase PB. Age-related changes in rat bone-marrow mesenchymal stem cell plasticity. BMC Cell Biol. 2011. 12:44.


23. Katsara O, Mahaira LG, Iliopoulou EG, Moustaki A, Antsaklis A, Loutradis D, Stefanidis K, Baxevanis CN, Papamichail M, Perez SA. Effects of donor age, gender, and in vitro cellular aging on the phenotypic, functional, and molecular characteristics of mouse bone marrow-derived mesenchymal stem cells. Stem Cells Dev. 2011. 20:1549–1561.


24. Zhu Y, Liu T, Song K, Fan X, Ma X, Cui Z. Adipose-derived stem cell: a better stem cell than BMSC. Cell Biochem Funct. 2008. 26:664–675.


25. Sun CK, Yen CH, Lin YC, Tsai TH, Chang LT, Kao YH, Chua S, Fu M, Ko SF, Leu S, Yip HK. Autologous transplantation of adipose-derived mesenchymal stem cells markedly reduced acute ischemia-reperfusion lung injury in a rodent model. J Transl Med. 2011. 9:118.


26. Peng L, Jia Z, Yin X, Zhang X, Liu Y, Chen P, Ma K, Zhou C. Comparative analysis of mesenchymal stem cells from bone marrow, cartilage, and adipose tissue. Stem Cells Dev. 2008. 17:761–773.


27. Yoshimura H, Muneta T, Nimura A, Yokoyama A, Koga H, Sekiya I. Comparison of rat mesenchymal stem cells derived from bone marrow, synovium, periosteum, adipose tissue, and muscle. Cell Tissue Res. 2007. 327:449–462.


28. Rebelatto CK, Aguiar AM, Moretão MP, Senegaglia AC, Hansen P, Barchiki F, Oliveira J, Martins J, Kuligovski C, Mansur F, Christofis A, Amaral VF, Brofman PS, Goldenberg S, Nakao LS, Correa A. Dissimilar differentiation of mesenchymal stem cells from bone marrow, umbilical cord blood, and adipose tissue. Exp Biol Med (Maywood). 2008. 233:901–913.


Fig. 1.
Image of BMSCs in passage 3 with 80% confluence (A) 10X (B) 20X. Image of ADSCs in passage 3 with 80% confluence (C) 10X. (D) 20X. (E) ADSCs show ability to form multilayer after confluent 20X.
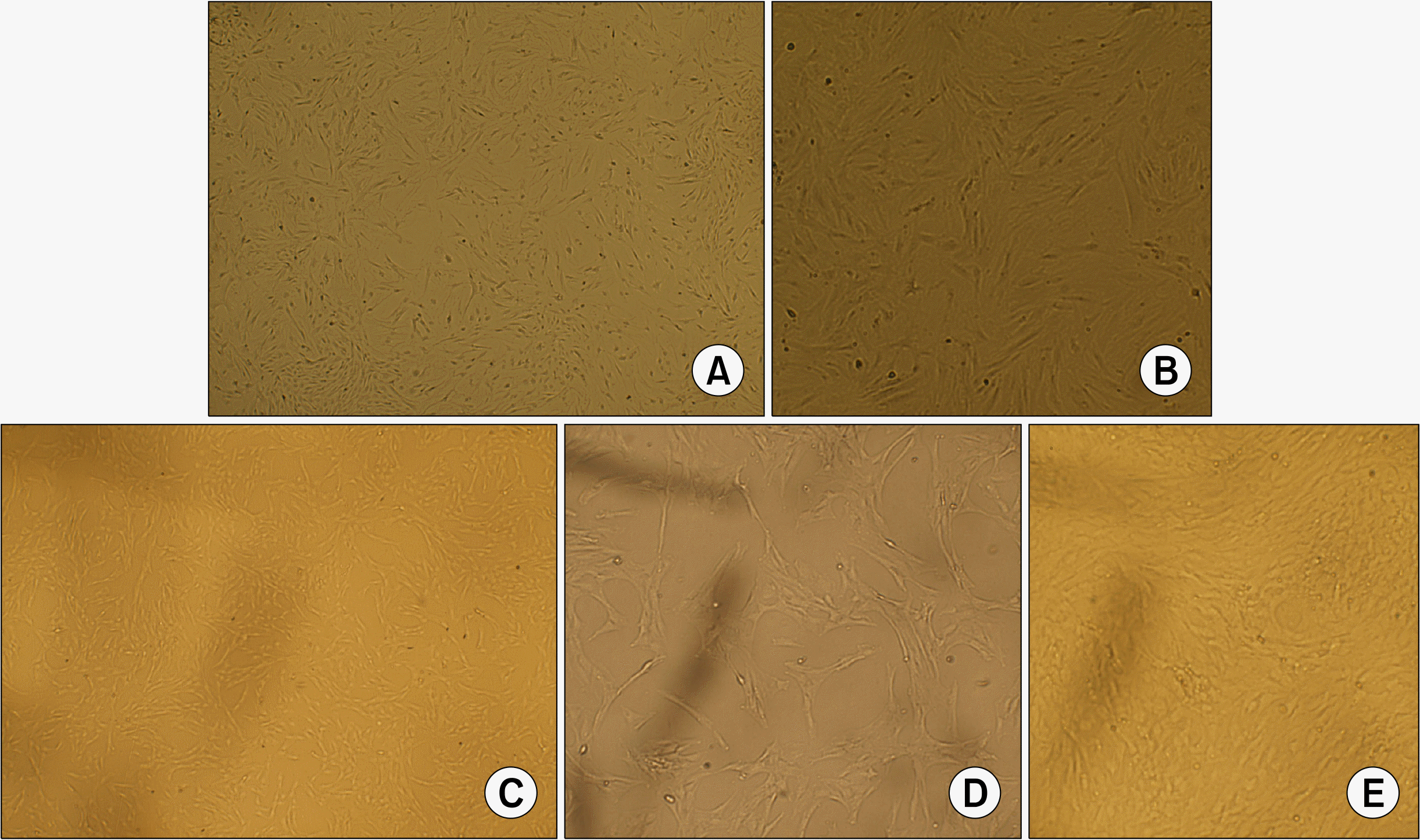
Fig. 2.
(A) Number of CFU.F for BMSCs & ADSCs when plated per 100-mm tissue culture dish. (B) PDT per hours for BMSCs & ADSCs. (C) Growth curve plotted for BMSCs & ADSCs.
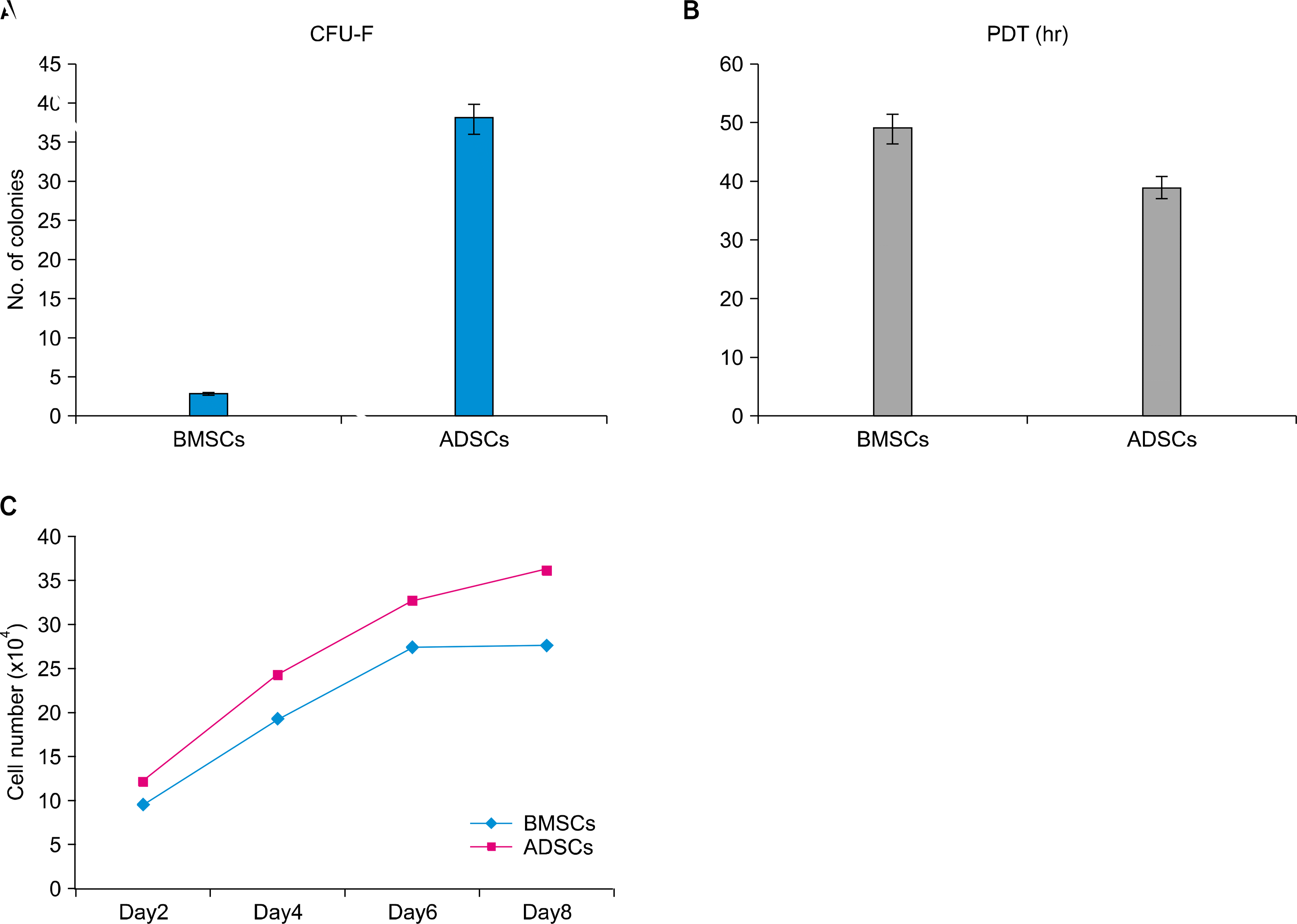
Fig. 3.
(A, B) Osteogenic Differentiation of (A) BMSCs and (B) ADSCs. Both stained with alizarin red. (C, D) Adipogenic Differentiation of (C) BMSCs and (D) ADSCs stained with oil red. (E) Chondrogenic Differentiation, this chart showing level of sulfated GAG for both BMSCs and ADSCs.

Table 1.
Immunophenotypic characterization of BMSCs and ADSCs