Abstract
Cancer stem cells (CSCs) are a small subset of cancer cells with stem cell-like properties, self-renewal potential, and differentiation capacity into multiple cell types. Critical genetic alterations or aberrantly activated signaling pathways associated with drug resistance and recurrence have been observed in multiple types of CSCs. In this context, CSCs are considered to be responsible for tumor initiation, growth, progression, therapeutic resistance, and metastasis. Therefore, to effectively eradicate CSCs, tremendous efforts have been devoted to identify specific target molecules that play a critical role in regulating their distinct functions and to develop novel therapeutics, such as proteins, monoclonal antibodies, selective small molecule inhibitors, and small antisense RNA (asRNA) drugs. Similar to other CSC types, oral CSCs can be characterized by certain pluripotency-associated markers, and oral CSCs can also survive and form 3D tumor spheres in suspension culture conditions. These oral CSC-targeting therapeutics selectively suppress specific surface markers or key signaling components and subsequently inhibit the stem-like properties of oral CSCs. A large number of new therapeutic candidates have been tested, and some products are currently in the pre-clinical or clinical development phase. In the present study, we review new oral CSC-targeted therapeutic strategies and discuss the various specific CSC surface markers and key signaling components involved in the stem-like properties, growth, drug resistance, and tumorigenicity of oral CSCs.
Head and neck cancer, including oral cancer, is the sixth leading type of cancer worldwide (1), representing approximately 6% of all solid tumors. Approximately 90% of diagnosed cases of head and neck cancers are oral squamous cell carcinoma (SCC), which is the most common malignancy in the oral cavity (2). In recent decades, the five-year survival rate after treatment of oral cancer is still approximately 50% for most countries (3-5), which indicates a poor prognosis for the developing world (6). Several chemotherapeutic agents for the treatment of oral cancer have been tested individually or in combination with other anticancer drugs, such as cetuximab, cisplatin, docetaxel, 5-fluorouracil, methotrexate, and paclitaxel (7-10). However, even in patients that are most sensitive to these chemotherapeutic drugs, they experience tumor recurrence due to the emergence of genetically modified cell populations, which ultimately leads to drug resistance and tumor re-growth (11). Indeed, the recurrence or metastasis rates for oral squamous cell carcinoma vary from 18% to 76% in patients undergoing standard treatment (12). The CSC theory provided a supportive explanation for drug resistance and subsequent tumor recurrence after chemotherapy. CSCs were first identified and characterized in the bone marrow of acute myeloid leukemia and described as key “seeds” for tumor initiation, growth, and therapy resistance (13). Currently, CSCs have also been found in almost all types of solid tumors, including those in the brain (14), colon (15), gastric (16), head and neck (17), lung (18), pancreatic (19), and prostate cancers (20), and are defined as a small subpopulation of tumor cells with stem cell-like characteristics. Unlike bulk tumor cells, they have both tumor-initiating potential and the capacity to reconstitute the bulk tumor with cellular heterogeneity, as observed in healthy tissues (21, 22). Furthermore, oral CSCs are commonly defined by several unique genomic and functional properties: (a) oral CSCs are intrinsically resistant to the majority of conventional chemotherapeutic agents; thus, the remaining CSC subpopu-lation can subsequently regenerate tumors in patients (23-25), (b) oral CSCs can be characterized by certain pluripotency-associated markers (22, 26-28), and (c) CSCs can survive and form 3D tumor spheres in suspension culture conditions (29-31). These functional characteristics of CSCs indicate that the majority of traditional therapeutic strategies, such as surgery with chemotherapy and/or radiation, can only kill the bulk tumor cells but leave rare, oral CSC subpopulations untouched. In this context, new therapeutic strategies that can effectively eradicate oral CSCs would ultimately improve treatment outcomes and enhance the quality of life of patients with oral cancer (32). However, there is not enough information currently available to make a conclusive statement regarding oral CSC-targeted therapeutic strategies. In this study, we provide current information about several genomic and functional properties of oral CSCs and discuss various therapeutic approaches targeting oral CSC-specific signaling pathways and pluripotency-associated markers involved in the maintenance and drug resistance of CSCs.
Given the functional similarities between somatic stem cells and CSCs, researchers have attempted to determine whether CSCs arise from tissue-resident stem/progenitor cells or differentiated cells that acquire stem cell-like characteristics during the malignant process. However, the origin of cancer stem cells remains elusive, because the origin of CSCs varies greatly depending on the malignancy (33, 34). Tissue-resident stem/progenitor cells exhibit unique biological features, such as long-term reconstitution, self-renewal, and transdifferentiation capacity. Functionally, stem cells self-renew asymmetrically but also give rise to daughter cells that are committed to lineage-specific differentiation to reconstitute specific tissues and maintain homeostasis. The tissue-resident stem cells that give rise to cancer is an attractive hypothesis, given that the multistage theory of tumor development requires actively dividing and long-lived stem cells in which consecutive genetic mutations can accumulate (35). Similarly, intermediate progenitor cells, which are more abundant in specific tissues than stem cells and commonly undergo limited cell proliferation, aberrantly acquire enhanced self-renewal capacity, and ultimately become the long-lived target that acquires consecutive genetic mutations (36). Indeed, a number of studies have hypothesized that tissue-resident stem cells or certain cells with stem cell characteristics can directly give rise to CSCs. Some characteristics and functions of leukemic CSCs with hierarchically arranged subpopulations (similar to those observed with normal bone marrow stem cells) support the stem-cell origin hypothesis (37). Interestingly, in some tissues, fully differentiated cells can also revert to the stem cell phenotype through dedifferentiation or reprogramming (38). Therefore, some researchers have hypothesized that cancer cells could be derived from mature and fully differentiated cells that re-acquire stem cell-like properties under certain conditions. In the “differentiated cell origin model”, the requisite of consecutive oncogenic mutations may lead to the dedifferentiation of mature cells into pluripotent stem cell-like cells. The corresponding mechanisms or physiological conditions that determine which cell types would undergo dedifferentiation have not been completely elucidated. Surprisingly, this dedifferentiation process can be accelerated by simple genetic modifications (39, 40). Indeed, Takahashi et al. (40) induced the dedifferentiation of fully differentiated somatic cells into pluripotent stem cells with multidifferentiation potential by introducing only four pluripotency-associated genes (Oct3/4, Sox2, c-Myc, and Klf4). Regardless of their cellular origin, whether tissue-resident stem/progenitor cells or differentiated somatic cells, CSCs are defined as the subpopulation of cells that retain stem cell-like characte-ristics. Similar to normal stem cells, CSCs can divide symmetrically or asymmetrically, giving rise to both other CSCs and more differentiated progenies that ultimately reconstitute the bulk tumor through differentiation into many different cell types.
The majority of cancer cells in heterogeneous bulk tumors have limited proliferative potential, tumor-forming capacity, and resistance to chemotherapy. The diverse phenotypic heterogeneity and plasticity among cancer cells in bulk tumors have been explained by the CSC model of hierarchically organized tumors (42). After their identification from leukemia, CSCs were first isolated and characterized from solid breast cancer tumors. The CD44+/CD24−/low and epithelial cell adhesion molecule-positive phenotypes may be typically considered pluripotency-as-sociated characteristics in breast CSCs (43, 44). As few as 100 cells exhibiting these cellular properties can grow rapidly in vitro and effectively form new tumors in vivo after reconstitution (43). The evidence of oral CSCs has been first suggested by the study, which showed that even just a small fraction of oral squamous cell carcinoma cells with “stem-like” characteristics is able to rapidly reconstitute a new bulk tumor after reconstitution (45). The isolation of oral CSCs from tumor masses has mainly been conducted using the cell surface marker CD44, which is an important cell surface marker for isolating stem-like cells from breast cancer (46, 47). Although CD44 was originally identified as a receptor for hyaluronic acid, it has also been suggested as a putative marker for various types of stem cells (48, 49) and a key regulator for the maintenance of the properties of various CSCs (50, 51). Aldehyde dehydrogenases (ALDH), a family of intracellular enzymes that catalyze cellular detoxification and subsequent drug resistance via oxidation of intracellular aldehydes, has been proposed as a putative biomarker for oral CSCs (52). Interestingly, ALDH-positive subpopulations isolated from head and neck squamous cell carcinoma have typical CSC characteristics and enhanced tumorigenic potential in vivo (53, 54). Overall, acquired resistance to standard chemotherapy or radiation therapy is an important cause of treatment failure. Emerging evidence indicate that CSC subpopulations are more resistant to standard chemotherapeutic drugs when compared to non-CSC subpo-pulations (55). CSC-mediated drug resistance has been previously demonstrated in multiple types of cancer, such as brain (56), breast (57), colorectal (58), leukemias (59), skin (60), and pancreatic (19) cancers. In addition, CSC-mediated radioresistance has also been reported in brain (61) and breast (62) cancers. Oral CSCs are also commonly resistant to various conventional chemotherapeutic agents (23-25) and radiation therapies (27, 63, 64) that target proliferating cells (Fig. 1). Therefore, the remaining resistant oral CSCs can proliferate extensively and reconstitute new tumors in patients and may ultimately lead to the failure of durable clinical responses. Therefore, research efforts are underway to develop novel therapeutic strategies for selectively eradicating resistant oral CSCs without affecting normal cells (65-67).
Therapeutic efficiency could be enhanced by selectively targeting subtle expression differences in surface markers and alterations in various signaling regulators between CSC subpopulations and non-CSC subpopulations. Currently, many researchers have identified several promising therapeutic targets for oral CSCs, including signaling pathways, cell surface markers, pluripotency-associated genes, and transcription factors that can selectively eliminate oral CSCs and subsequently reduce the risk of cancer recurrence (Fig. 2).
A rare subpopulation of CSCs can be identified and isolated from the tumor mass by using single or combinations of multiple surface markers, thus providing a more effective CSC-targeted therapeutic strategy (68). Currently, magnetic cell sorting, flow cytometry, fluorescent antibody staining, and real-time PCR are commonly used to isolate and characterize heterogeneous CSCs within a tumor mass. Although considerable progress has been achieved in identifying CSCs on the basis of their specific surface makers, the development of selective CSC therapies remains a challenge, largely due to the many common properties between CSCs and normal stem cells.
Aldehyde dehydrogenase: Aldehyde dehydrogenases (ALDHs) are a superfamily of intracellular enzymes that play important roles in cellular detoxification and subsequent drug resistance by metabolizing various intracellular aldehyde derivatives. Over two decades ago, ALDHs were first known to confer resistance to chemotherapeutic alkylating agents such as cyclophosphamide in hematopoietic and leukemic stem cells (69). ALDH-positive subpopulations were found in breast (70) and brain (71) cancers. In these tumors, cells with high ALDH activity were characterized as having enhanced self-renewal capacity and subsequent tumorigenic potential in vivo, which are typical characteristics of CSCs. Indeed, many recent studies have revealed that high levels of endogenous ALDH activity may be associated with increased in vitro clonogenic activity, tumorigenic properties, and drug resistance in oral CSCs (27, 53, 72, 73). ALDH1 protein expression levels are positively correlated with the tumor formation ability of neck squamous cell carcinoma and negatively correlated with patients’ responses to ongoing treatments (27). Consistently, as few as 5×102 ALDH1-positive cells of head and neck squamous cell carcinoma were able to reconstitute visible tumors in vivo, and these subpopulations showed increased 3D sphere-forming ability in vitro, higher migratory capacity, and enhanced radiation resistance (27, 53). Interestingly, the ALDHhigh subpopulation significantly overlaps with the CSC surface marker CD44-expressing populations (50.6%∼74.4%). Conversely, when CD44-positive subpopulations were sorted for ALDH activity, only 9.8%∼23.6% of the CD44+ cells overlapped with high ALDH activity (53). This result suggests that ALDH activity can be used as a selective marker for CSCs in head and neck squamous cell carcinoma (53). In addition, ALDH+ stem-like populations were also associated with epithelial-to-mesenchymal transition (EMT), which is a key process in metastasis during malignant progression in head and neck squamous cell carcinoma (27).
CD44: CD44 is a transmembrane receptor for hyaluronic acid, which is highly expressed in many cancers, and regulates cell migration and invasion processes (74). The CD44-hyaluronic acid signaling axis can promote tumor progression and subsequent metastasis by increasing self-renewal capacity, cell survival, and drug resistance (75, 76). CD44 can also bind to several growth factors and some metalloproteinases (MMPs), such as MMP-2, MMP-9, and MMP-14, resulting in an increased ability for metastasis (77, 78), angiogenesis (79), and drug resistance (80). CD44-positive subpopulations with high tumorigenic potential have been identified in multiple types of CSCs, such as in cervical (81), prostate (82), lung (83), breast (43), colon (84), ovarian (85), gastric (86), bladder (87), and pancreatic (88) cancer. In this context, the CD44high subpopulation with elevated tumorigenicity and metastatic ability is an attractive therapeutic strategy for the treatment of multiple cancer types. Emerging evidence has revealed the association of CD44 with tumor aggressiveness and its prognostic impact on patients with oral squamous cell carcinoma (89, 90). Consistently, it has been described that certain CD44 (i.e., v3, v6, v10) variant isoforms seem to act as metastasis genes and are associated with metastasis and cancer progression in oral squamous cell carcinoma (91). CD44-positive cell subpopulations express high levels of Bmi-1, which plays a key role in the self-renewal capacity of various stem cell types and is involved in tumorigenesis (22). Since then, many previous studies have suggested that CD44-positive cells exhibit a significantly higher potential for 3D sphere-forming ability in vitro, higher migratory capacity, and drug resistance in both primary tumor tissues and cell lines of oral squamous cell carcinoma (22, 92-96). In addition, the frequency of CD44-positive cells was significantly associated with poor prognosis and higher rates of recurrence and metastasis after radiation therapy in patients with oral squamous cell carcinoma (92).
CD133: CD133 (also known as AC133 and prominin-1), a 5-pass transmembrane glycoprotein with a molecular mass of 120 kDa, has been identified as a novel marker for hematopoietic stem/precursor cells and endothelial progenitor cells in various organs (97). However, several previous studies have indicated that CD133 can also be used as a marker for the identification of CSCs in many solid tumors, including hepatocellular carcinoma (98), oral squamous carcinoma (99), prostate carcinoma (100), renal cell carcinoma (101), and thyroid carcinoma (102). Consistently, CD133-positive subpopulations from the head and neck squamous cell carcinoma cell line Hep-2 have a markedly increased capacity for tumor formation in vivo when compared with CD133-negative cells (103). Previous studies have revealed a significant correlation between enhanced levels of CD133 and poor prognosis in patients with oral squamous cell carcinoma (104, 105). Moreover, CD133-positive cells showed increased drug resistance, self-renewal ability in vitro, tumorigenesis in vivo, and expression levels of pluripotency-associated genes, such as ALDH, NANOG, OCT4, and SOX2 (28). Elevated expression of CD133 was observed in oral cancer stem-like cells from patients with oral squamous cell carcinoma (104). Its expression is also correlated with increased migration, tumorigenicity, and the expression level of the ABC transporter gene ABCG2 (104). Additionally, oral cancer patients who are NANOG/OCT4/CD133 triple-positive were predicted to have the worst survival prognosis. However, due to differences in research design, sample size, and target population, the functions and mechanisms of CD133 in various aspects of oral CSCs are still not clear, and further investigation is necessary.
CD177: CD117, also known as the c-kit receptor, is a transmembrane protein with tyrosine kinase activity that plays a pivotal role in the maintenance and proliferation of hematopoietic stem cells (106). CD117 depletion in the bone marrow or spleen leads to a significant decrease in the number of cells from the erythrocyte and lymphocyte lineages (107). The CD117 signaling cascade is activated when CD117 binds to its cognate ligand, the stem cell factor (SCF), leading to the activation of the MAPK cascade (cRaf/Mek/Erk), the JAK/STAT pathway, and PI3K signaling events (108). The signaling pathways stimulated by CD117 are involved in various important cellular functions, such as self-renewal, apoptosis/survival, differentiation, and cell migration (109). CD117-positive cells were observed in the bone marrow, lung, spleen, and thymus (110). Previous studies have shown that CD117 is overexpressed in a number of solid cancer types, such as breast cancers (111), gastrointestinal stromal tumors (112), germ cell tumors (113), salivary gland tumors (114), and small cell lung cancer (115). Ongkeko et al. (116) analyzed the expression levels of CD117 in 44 patients with primary oral squamous cell carcinoma. Although its expression did not correlate with disease-free survival, the expression of CD117 was significantly higher in oral squamous cell carcinoma of the pharynx than in laryngeal cancers. Tsai et al. (117) demonstrated that chemoresis-tance to cisplatin was possibly correlated with the upregulation of CD117 and the ABC transporter gene (ABCG2) in oral cancer stem-like cells. However, experimental evidence regarding the reactivity of oral squamous cell carcinoma to CD117 expression is limited and contradictory. While Mărgăritescu et al. (118) and Galbiatti-Dias et al. (119) used CD117 with other stemness-related genes to identify and isolate oral cancer stem-like cells from oral squamous cell carcinomas, Barth et al. (120) analyzed CD117 expression in squamous cell carcinomas of the oral cavity, pharynx, and larynx and found that its expression was restricted only to stromal spindle cells.
The most frequently used strategy for the isolation, characterization, and targeting of CSCs within the tumor mass is based on specific cell surface biomarkers, such as CD133, CD44, and CD24 (34, 68, 121). However, these pluripotency-associated markers are not uniquely specific for CSCs, and they frequently overlap with their tissue-resident stem cells as well as normal somatic cells in many organs (122). Therefore, the use of single cell surface markers is considered under debate for selectively targeting CSCs within the tumor mass, because many studies found no cells with their specific characteristics (123). Alternatively, CSCs have also been identified and eliminated by selectively targeting key CSC-specific signaling pathways involved in their maintenance and various tumorigenic functions. Although the relationship between certain signaling pathways and several CSC functions still needs to be fully characterized, some of them appear useful for developing CSC-specific therapeutic strategies that avoid the deleterious side effects caused by affecting normal somatic cells or tissue-resident stem cells.
Wnt/β-catenin signaling pathway: Wnt proteins are a group of secreted glycoproteins that bind to cell surface receptors, including their cognate Frizzled (Fzd) receptors, and cause an accumulation of β-catenin in the cytoplasm (124). It regulates numerous processes essential for embryogenesis, tissue homeostasis, and cancer development (41, 125). Wnt/β-Catenin-mediated signaling, which is implicated in controlling various aspects of CSC-related tumorigenicity, has also been found to be involved in the maintenance, survival, metastasis, and drug resistance of various CSC types, including acute myeloid leukemia (126), breast (127), colon (128), liver (129), and lung (130) cancer. Iwai et al. (131) suggests that the Wnt/β-catenin signaling pathway plays a critical role in the oncogenesis of oral squamous cell carcinoma. They also demonstrated that the aberrant cytoplasmic accumulation of β-catenin can enhance the invasion and migration of oral squamous cell carcinoma by upregulating MMP-7 expression and inducing epithelial-mesenchymal transition (EMT) (131). Consistently, Yang et al. (132) reported that introducing a β-catenin gene into oral squamous cell carcinoma cells using a retrovirus vector-mediated transfection system resulted in a significantly increased resistance to TNF-α-induced apoptosis in transfected cells. Warrier et al. (133) also clearly demonstrated that Wnt/β-catenin signaling can increase the in vitro sphere-forming capacity and drug resistance of oral CSCs. They also reported that oral CSCs from squamous cell carcinoma are chemosensitized by naturally occurring Wnt inhibitors (sFRP4), via increasing apoptosis and/or reducing pluripotency (133). All-trans-retinoic acid (ATRA) reduced the self-renewal capacity of oral CSCs in vitro and subsequent tumorigenicity in vivo by inhibiting Wnt/β-catenin signaling from squamous cell carcinoma (134).
Notch signaling pathways: Notch signaling is an evolutionarily conserved intercellular signaling pathway that regulates various aspects of development and disease progression (135, 136). Therefore, dysfunction frequently results in a variety of congenital anomalies and diverse pathological disorders (41). The Notch receptors are four conserved single-pass transmembrane proteins (Notch1-4) and contain multiple arranged epidermal growth factor-like repeats (EGFR) that bind to Notch ligands (137). Among their family members, Notch 1 and 2 share the highest degree of similarity and are the most widely expressed in a large number of fetal and adult tissues, while Notch 3 is primarily limited to vascular smooth muscle cells, and Notch 4 is most predominantly expressed in endothelial cells (138). While the pro-oncogenic functions of dysregulated Notch signaling have been relatively well characterized in multiple types of cancers (138-140), its role in many aspects of CSCs is just emerging. Notch1 mutations are found in approximately 10%∼15% of patients with oral squamous cell carcinoma, suggesting its possible roles in the unique biological features of oral CSCs, such as long-term reconstitution, self-renewal, and transdifferentiation capacity (141-143). Shrivastava et al. (144) demonstrated that Notch1 was highly expressed in oral squamous cell carcinoma-derived 3D sphere-forming cells compared to monolayer cells. Consistently, they also observed the activation of Hes1, a well-known target of Notch signaling, in oral squamous cell carcinoma-derived sphere-forming cells as compared to adjacent monolayer cells (144). Moreover, Lee et al. (145) found that prolonged exposure to tumor necrosis factor alpha (TNF-α), a major proinflammatory cytokine, significantly enhanced multiple oral CSC-associated characteristics such as self-renewal capacity, pluripotency-associated genes, drug resistance, and tumorigenic potential in vivo by activating the Notch-Hes1 signaling cascade. Zou et al. (146) also reported that Notch2 expression was markedly increased in ALDH-positive CSC-like subpopulations in tongue squamous cell carcinoma. These results suggest that activation of the Notch signaling pathway can be mechanistically associated with the various characteristics of oral CSCs.
Hh signaling pathways: Hedgehog signaling was initially discovered as a critical segment polarity gene of pattern formation during early embryonic development in Drosophila, and its dysfunction frequently results in critical developmental anomalies and diverse pathological disorders (147, 148). It plays an essential role in regulating diverse cellular functions such as cell growth, survival/apoptosis, cell migration/invasion, and embryonic cell differentiation (149-152). While only one Hh gene has been identified in Drosophila, three different Hh family members have been found in vertebrates: the Desert Hedgehog (DHh), Indian Hedgehog (IHh), and Sonic Hedgehog (SHh) (153-155). Upon binding to its cognate receptor Patched-1 (a 12-pass transmembrane glycopro-tein), Hh initiates signal transduction through the transcription factor Gli, either dependently or independently (156). Many previous studies on several different types of human cancer, such as breast cancer (157), chronic myeloid leukemia (158), colorectal cancer (159), glioblastoma (160), lung cancer (161), multiple myeloma (162), and pancreatic cancer (163), have indicated that the Hh signaling network can possibly increase the self-renewal capacity, drug resistance, and tumorigenic potential of various CSC types. Consistently, Takebe et al. (164) provided a comprehensive survey of the Hh signaling pathway as a major regulator of many fundamental functions of CSCs, such as drug resistance, tumorigenic potential, pluripotency, and self-renewal capacity. Wang et al. (165) demonstrated that Shh is highly expressed in approximately 70% of oral squamous cell carcinoma specimens. In addition, statistical analyses showed that Shh overexpression is highly associated with the enhanced expression of the well-known Hh target gene Gli-1 and Hh receptor Ptch, suggesting that Hedgehog signaling is likely activated in oral squamous cell carcinoma cells (165). Consistently, Ptch expression is significantly associated with recurrence rates in patients with oral squamous cell carcinoma (165). Therefore, targeting dysregulated Hh signaling activity may provide effective diagnostic and therapeutic strategies for the treatment of oral cancers.
Hippo-YAP signaling pathways: The Hippo-YAP signaling cascades have been found as important oncogenic signaling pathways in various types of cancers (166). Its signaling activity is regulated by key downstream transcription co-activator Yes-associated protein 1 (YAP1) with PDZ-binding motif (TAZ) (167). In addition, YAP1 is essential transcription factor for maintaining undifferentiated state of embryonic stem cells by increasing the expression levels of various pluripotency-associated factors (168, 169). Importantly, significantly deregulated Hippo-YAP signaling pathway is widely observed in multiple types of human cancers including oral squamous cell carcinoma (170, 171). Indeed, local amplification of 11q22 locus in the human YAP1 gene is observed in 8.6% of patients with head-and-neck squamous cell carcinoma (172) and it signaling activity is closely related with poor prognosis and malignant phenotypes both in vitro and in vivo (172, 173). Endogenous YAP1 hyperactivation promotes surprisingly rapid and highly reproducible tumorigenesis of head-and-neck squamous cell carcinoma (174). Importantly, Li et al. (175) found that Hippo-TAZ signaling cascades enriched in CSCs subpopulation (CD44+/CD133+) by promoting the expression of pluripotency-associated transcription factor SOX2 in head neck squamous cell carci-noma. Similarly, several lines of evidence suggested that YAP1 could transcriptionally induce the expression of reprogramming factor SOX2 through a physical interaction with pluripotency-associated gene OCT4 to promote self-renewal capacity of CSCs subpopulation in lung cancer model (176).
Since the identification of CSC subpopulations in oral squamous cell carcinoma (22), a number of studies have described the positive correlation between the presence of oral CSCs and poor clinical outcomes (177, 178). Chen et al. (179) showed that the relative expression levels of ALDH1 and CD44 were significantly higher in high-grade oral squamous cell carcinoma. They also found that cucurbitacin I (JSI-124) can effectively induce the apoptosis of CD44+/ALDH1+ oral squamous cell carcinoma subpopulations through STAT3 signaling pathways (179). Likewise, Chen et al. (27) also demonstrated that ALDH1-positive cell subpopulations from oral squamous cell carcinoma have higher tumorigenic potential and are more resistant to chemotherapeutic agents and radiation than adjacent ALDH1-negative cell subpopulations. In this context, chemotherapy and radiation, the most commonly used therapeutic strategies together with surgery, often fail, as they do not effectively eliminate quiescent oral CSCs, which can reconstitute the entire bulk tumor. Therefore, it appears that establishing new adjuvant therapeutic approaches that can effectively eliminate oral CSCs within the tumor mass may provide a more effective treatment strategy to overcome therapeutic resistance and subsequent recurrence. Identifying highly selective oral CSC surface markers, as well as establishing effective therapeutic strategies, still requires intensive investigation (180, 181). Currently, therapeutic attempts to selectively target oral CSCs have not yet been used in clinical applications. Moreover, various pluripotency-associated CSC surface markers are not exclusively unique for oral CSCs, and they overlap with their tissue-resident stem cells as well as normal somatic cells (182). Therefore, a better understanding of CSC characteristics and the development of novel therapeutic approaches that selectively target oral CSCs are urgently needed to increase the clinical outcome in patients with different types of malignan-cies.
After the identification of CSCs from leukemia over 40 years ago, they were first isolated and characterized from solid breast cancer tumors. CSCs not only play an essential role in cancer initiation, maintenance, and tumor progression, but they are also particularly important for mediating resistance to chemotherapeutic drugs and radiation, subsequently leading to the failure of these conventional therapeutic approaches. In this context, identifying and selectively targeting CSCs with specific pluripotency-associated signaling pathways and/or surface markers is a potential therapeutic strategy for inhibiting various types of cancer. However, no single cell surface marker that can specifically target the oral CSC subpopulation is currently available. The identification of specific surface markers or their signaling regulators is the first step in uncovering the characteristics and functions of oral CSCs. Indeed, combinations of a set of putative cell surface markers and/or signaling pathways representing the pluripotency-associated phenotypes will be helpful in achieving a better chance of developing new therapeutic alternatives for patients with oral cancer.
Acknowledgments
This work was supported by the Nattional Research Foundation of Korea (NRF) grant funded by the Korea government (MSIP) (NRF-2020R1I1A2061281).
References
1. Williams HK. 2000; Molecular pathogenesis of oral squamous carcinoma. Mol Pathol. 53:165–172. DOI: 10.1136/mp.53.4.165. PMID: 11040937. PMCID: PMC1186964.


2. Jemal A, Siegel R, Ward E, Hao Y, Xu J, Thun MJ. 2009; Cancer statistics, 2009. CA Cancer J Clin. 59:225–249. DOI: 10.3322/caac.20006. PMID: 19474385.


3. Kantola S, Parikka M, Jokinen K, Hyrynkangs K, Soini Y, Alho OP, Salo T. 2000; Prognostic factors in tongue cancer - relative importance of demographic, clinical and histopathological factors. Br J Cancer. 83:614–619. DOI: 10.1054/bjoc.2000.1323. PMID: 10944601. PMCID: PMC2363505.


4. Sankaranarayanan R, Masuyer E, Swaminathan R, Ferlay J, Whelan S. 1998; Head and neck cancer: a global perspective on epidemiology and prognosis. Anticancer Res. 18:4779–4786. PMID: 9891557.
5. Carvalho AL, Magrin J, Kowalski LP. 2003; Sites of recurrence in oral and oropharyngeal cancers according to the treatment approach. Oral Dis. 9:112–118. DOI: 10.1034/j.1601-0825.2003.01750.x. PMID: 12945592.


6. Jemal A, Bray F, Center MM, Ferlay J, Ward E, Forman D. 2011; Global cancer statistics. CA Cancer J Clin. 61:69–90. DOI: 10.3322/caac.20107. PMID: 21296855.


7. Catimel G, Verweij J, Mattijssen V, Hanauske A, Piccart M, Wanders J, Franklin H, Le Bail N, Clavel M, Kaye SB. 1994; Docetaxel (Taxotere): an active drug for the treatment of patients with advanced squamous cell carcinoma of the head and neck. EORTC Early Clinical Trials Group. Ann Oncol. 5:533–537. DOI: 10.1093/oxfordjournals.annonc.a058908. PMID: 7918125.


8. Clavel M, Vermorken JB, Cognetti F, Cappelaere P, de Mulder PH, Schornagel JH, Tueni EA, Verweij J, Wildiers J, Clerico M, Dalesio O, Kirkpatrick A, Snow GB. 1994; Randomized comparison of cisplatin, methotrexate, bleomycin and vincristine (CABO) versus cisplatin and 5-fluorouracil (CF) versus cisplatin (C) in recurrent or metastatic squamous cell carcinoma of the head and neck. A phase III study of the EORTC Head and Neck Cancer Cooperative Group. Ann Oncol. 5:521–526. DOI: 10.1093/oxfordjournals.annonc.a058906. PMID: 7522527.


9. Haddad R, Sonis S, Posner M, Wirth L, Costello R, Braschayko P, Allen A, Mahadevan A, Flynn J, Burke E, Li Y, Tishler RB. 2009; Randomized phase 2 study of concomitant chemoradiotherapy using weekly carboplatin/paclitaxel with or without daily subcutaneous amifostine in patients with locally advanced head and neck cancer. Cancer. 115:4514–4523. DOI: 10.1002/cncr.24525. PMID: 19634161.


10. Vermorken JB, Mesia R, Rivera F, Remenar E, Kawecki A, Rottey S, Erfan J, Zabolotnyy D, Kienzer HR, Cupissol D, Peyrade F, Benasso M, Vynnychenko I, De Raucourt D, Bokemeyer C, Schueler A, Amellal N, Hitt R. 2008; Platinum-based chemotherapy plus cetuximab in head and neck cancer. N Engl J Med. 359:1116–1127. DOI: 10.1056/NEJMoa0802656. PMID: 18784101.


11. Greenberg JS, El Naggar AK, Mo V, Roberts D, Myers JN. 2003; Disparity in pathologic and clinical lymph node staging in oral tongue carcinoma. Implication for therapeutic decision making. Cancer. 98:508–515. DOI: 10.1002/cncr.11526. PMID: 12879467.


12. Shah JP, Lydiatt W. 1995; Treatment of cancer of the head and neck. CA Cancer J Clin. 45:352–368. DOI: 10.3322/canjclin.45.6.352. PMID: 7583907.


13. Visvader JE, Lindeman GJ. 2012; Cancer stem cells: current status and evolving complexities. Cell Stem Cell. 10:717–728. DOI: 10.1016/j.stem.2012.05.007. PMID: 22704512.


14. Hemmati HD, Nakano I, Lazareff JA, Masterman-Smith M, Geschwind DH, Bronner-Fraser M, Kornblum HI. 2003; Cancerous stem cells can arise from pediatric brain tumors. Proc Natl Acad Sci U S A. 100:15178–15183. DOI: 10.1073/pnas.2036535100. PMID: 14645703. PMCID: PMC299944.


15. O'Brien CA, Pollett A, Gallinger S, Dick JE. 2007; A human colon cancer cell capable of initiating tumour growth in immunodeficient mice. Nature. 445:106–110. DOI: 10.1038/nature05372. PMID: 17122772.
16. Fukuda K, Saikawa Y, Ohashi M, Kumagai K, Kitajima M, Okano H, Matsuzaki Y, Kitagawa Y. 2009; Tumor initiating potential of side population cells in human gastric cancer. Int J Oncol. 34:1201–1207. DOI: 10.3892/ijo_00000248. PMID: 19360333.


17. Abdulmajeed AA, Dalley AJ, Farah CS. 2013; Putative cancer stem cell marker expression in oral epithelial dysplasia and squamous cell carcinoma. J Oral Pathol Med. 42:755–760. DOI: 10.1111/jop.12073. PMID: 23614644.


18. Eramo A, Lotti F, Sette G, Pilozzi E, Biffoni M, Di Virgilio A, Conticello C, Ruco L, Peschle C, De Maria R. 2008; Identification and expansion of the tumorigenic lung cancer stem cell population. Cell Death Differ. 15:504–514. DOI: 10.1038/sj.cdd.4402283. PMID: 18049477.


19. Hermann PC, Huber SL, Herrler T, Aicher A, Ellwart JW, Guba M, Bruns CJ, Heeschen C. 2007; Distinct populations of cancer stem cells determine tumor growth and metastatic activity in human pancreatic cancer. Cell Stem Cell. 1:313–323. DOI: 10.1016/j.stem.2007.06.002. PMID: 18371365.


20. Collins AT, Berry PA, Hyde C, Stower MJ, Maitland NJ. 2005; Prospective identification of tumorigenic prostate cancer stem cells. Cancer Res. 65:10946–10951. DOI: 10.1158/0008-5472.CAN-05-2018. PMID: 16322242.


21. da Silva SD, Hier M, Mlynarek A, Kowalski LP, Alaoui-Jamali MA. 2012; Recurrent oral cancer: current and emerging therapeutic approaches. Front Pharmacol. 3:149. DOI: 10.3389/fphar.2012.00149. PMID: 23060791. PMCID: PMC3459356.


22. Prince ME, Sivanandan R, Kaczorowski A, Wolf GT, Kaplan MJ, Dalerba P, Weissman IL, Clarke MF, Ailles LE. 2007; Identification of a subpopulation of cells with cancer stem cell properties in head and neck squamous cell carcinoma. Proc Natl Acad Sci U S A. 104:973–978. DOI: 10.1073/pnas.0610117104. PMID: 17210912. PMCID: PMC1783424.


23. Sinha N, Mukhopadhyay S, Das DN, Panda PK, Bhutia SK. 2013; Relevance of cancer initiating/stem cells in carcinogenesis and therapy resistance in oral cancer. Oral Oncol. 49:854–862. DOI: 10.1016/j.oraloncology.2013.06.010. PMID: 23876628.


24. Todoroki K, Ogasawara S, Akiba J, Nakayama M, Naito Y, Seki N, Kusukawa J, Yano H. 2016; CD44v3+/CD24-cells possess cancer stem cell-like properties in human oral squamous cell carcinoma. Int J Oncol. 48:99–109. DOI: 10.3892/ijo.2015.3261. PMID: 26647656. PMCID: PMC4734600.


25. Pozzi V, Sartini D, Rocchetti R, Santarelli A, Rubini C, Morganti S, Giuliante R, Calabrese S, Di Ruscio G, Orlando F, Provinciali M, Saccucci F, Lo Muzio L, Emanuelli M. 2015; Identification and characterization of cancer stem cells from head and neck squamous cell carcinoma cell lines. Cell Physiol Biochem. 36:784–798. DOI: 10.1159/000430138. PMID: 26021266.


26. Okamoto A, Chikamatsu K, Sakakura K, Hatsushika K, Takahashi G, Masuyama K. 2009; Expansion and characterization of cancer stem-like cells in squamous cell carcinoma of the head and neck. Oral Oncol. 45:633–639. DOI: 10.1016/j.oraloncology.2008.10.003. PMID: 19027347.


27. Chen YC, Chen YW, Hsu HS, Tseng LM, Huang PI, Lu KH, Chen DT, Tai LK, Yung MC, Chang SC, Ku HH, Chiou SH, Lo WL. 2009; Aldehyde dehydrogenase 1 is a putative marker for cancer stem cells in head and neck squamous cancer. Biochem Biophys Res Commun. 385:307–313. DOI: 10.1016/j.bbrc.2009.05.048. PMID: 19450560.


28. Zhang Q, Shi S, Yen Y, Brown J, Ta JQ, Le AD. 2010; A subpopulation of CD133(+) cancer stem-like cells characterized in human oral squamous cell carcinoma confer resistance to chemotherapy. Cancer Lett. 289:151–160. DOI: 10.1016/j.canlet.2009.08.010. PMID: 19748175.


29. Khammanivong A, Gopalakrishnan R, Dickerson EB. 2014; SMURF1 silencing diminishes a CD44-high cancer stem cell-like population in head and neck squamous cell carcinoma. Mol Cancer. 13:260. DOI: 10.1186/1476-4598-13-260. PMID: 25471937. PMCID: PMC4265428.


30. Shigeishi H, Biddle A, Gammon L, Emich H, Rodini CO, Gemenetzidis E, Fazil B, Sugiyama M, Kamata N, Mackenzie IC. 2013; Maintenance of stem cell self-renewal in head and neck cancers requires actions of GSK3β influenced by CD44 and RHAMM. Stem Cells. 31:2073–2083. DOI: 10.1002/stem.1418. PMID: 23649588.


31. Biddle A, Liang X, Gammon L, Fazil B, Harper LJ, Emich H, Costea DE, Mackenzie IC. 2011; Cancer stem cells in squamous cell carcinoma switch between two distinct phenotypes that are preferentially migratory or prolifera-tive. Cancer Res. 71:5317–5326. DOI: 10.1158/0008-5472.CAN-11-1059. PMID: 21685475.


32. Boman BM, Huang E. 2008; Human colon cancer stem cells: a new paradigm in gastrointestinal oncology. J Clin Oncol. 26:2828–2838. DOI: 10.1200/JCO.2008.17.6941. PMID: 18539961.


33. Croker AK, Allan AL. 2008; Cancer stem cells: implications for the progression and treatment of metastatic disease. J Cell Mol Med. 12:374–390. DOI: 10.1111/j.1582-4934.2007.00211.x. PMID: 18182063. PMCID: PMC3822530.


34. Clarke MF, Dick JE, Dirks PB, Eaves CJ, Jamieson CH, Jones DL, Visvader J, Weissman IL, Wahl GM. 2006; Cancer stem cells--perspectives on current status and future directions: AACR Workshop on cancer stem cells. Cancer Res. 66:9339–9344. DOI: 10.1158/0008-5472.CAN-06-3126. PMID: 16990346.


35. Vogelstein B, Fearon ER, Hamilton SR, Kern SE, Preisinger AC, Leppert M, Nakamura Y, White R, Smits AM, Bos JL. 1988; Genetic alterations during colorectal-tumor development. N Engl J Med. 319:525–532. DOI: 10.1056/NEJM198809013190901. PMID: 2841597.


36. Passegué E, Jamieson CH, Ailles LE, Weissman IL. 2003; Normal and leukemic hematopoiesis: are leukemias a stem cell disorder or a reacquisition of stem cell characteristics? Proc Natl Acad Sci U S A. 100(Suppl 1):11842–11849. DOI: 10.1073/pnas.2034201100. PMID: 14504387. PMCID: PMC304096.


37. Hope KJ, Jin L, Dick JE. 2004; Acute myeloid leukemia originates from a hierarchy of leukemic stem cell classes that differ in self-renewal capacity. Nat Immunol. 5:738–743. DOI: 10.1038/ni1080. PMID: 15170211.


38. Zhou D, Luo Y, Dingli D, Traulsen A. 2019; The invasion of de-differentiating cancer cells into hierarchical tissues. PLoS Comput Biol. 15:e1007167. DOI: 10.1371/journal.pcbi.1007167. PMID: 31260442. PMCID: PMC6625723.


39. Yu J, Vodyanik MA, Smuga-Otto K, Antosiewicz-Bourget J, Frane JL, Tian S, Nie J, Jonsdottir GA, Ruotti V, Stewart R, Slukvin II, Thomson JA. 2007; Induced pluripotent stem cell lines derived from human somatic cells. Science. 318:1917–1920. DOI: 10.1126/science.1151526. PMID: 18029452.


40. Takahashi K, Tanabe K, Ohnuki M, Narita M, Ichisaka T, Tomoda K, Yamanaka S. 2007; Induction of pluripotent stem cells from adult human fibroblasts by defined factors. Cell. 131:861–872. DOI: 10.1016/j.cell.2007.11.019. PMID: 18035408.


41. Abdou AG, Maraee AH, El-Sayed EM, Elnaidany NF. 2011; Immunohistochemical expression of ezrin in cutaneous basal and squamous cell carcinomas. Ann Diagn Pathol. 15:394–401. DOI: 10.1016/j.anndiagpath.2011.05.005. PMID: 21849257.


42. Reya T, Morrison SJ, Clarke MF, Weissman IL. 2001; Stem cells, cancer, and cancer stem cells. Nature. 414:105–111. DOI: 10.1038/35102167. PMID: 11689955.


43. Al-Hajj M, Wicha MS, Benito-Hernandez A, Morrison SJ, Clarke MF. 2003; Prospective identification of tumorigenic breast cancer cells. Proc Natl Acad Sci U S A. 100:3983–3988. DOI: 10.1073/pnas.0530291100. PMID: 12629218. PMCID: PMC153034.


44. Fillmore CM, Kuperwasser C. 2008; Human breast cancer cell lines contain stem-like cells that self-renew, give rise to phenotypically diverse progeny and survive chemotherapy. Breast Cancer Res. 10:R25. DOI: 10.1186/bcr1982. PMID: 18366788. PMCID: PMC2397524.


45. Mackenzie IC. 2004; Growth of malignant oral epithelial stem cells after seeding into organotypical cultures of normal mucosa. J Oral Pathol Med. 33:71–78. DOI: 10.1111/j.1600-0714.2004.00157.x. PMID: 14720192.


46. de Moraes FP, Lourenço SV, Ianez RC, de Sousa EA, Silva MM, Damascena AS, Kowalski LP, Soares FA, Coutinho-Camillo CM. 2017; Expression of stem cell markers in oral cavity and oropharynx squamous cell carcinoma. Oral Surg Oral Med Oral Pathol Oral Radiol. 123:113–122. DOI: 10.1016/j.oooo.2016.09.009. PMID: 27866975.


47. Mohanta S, Siddappa G, Valiyaveedan SG, Dodda Thimmasandra Ramanjanappa R, Das D, Pandian R, Khora SS, Kuriakose MA, Suresh A. 2017; Cancer stem cell markers in patterning differentiation and in prognosis of oral squamous cell carcinoma. Tumour Biol. 39:1010428317703656. DOI: 10.1177/1010428317703656. PMID: 28631562.


48. Thapa R, Wilson GD. 2016; The importance of CD44 as a stem cell biomarker and therapeutic target in cancer. Stem Cells Int. 2016:2087204. DOI: 10.1155/2016/2087204. PMID: 27200096. PMCID: PMC4856920.
49. Morath I, Hartmann TN, Orian-Rousseau V. 2016; CD44: more than a mere stem cell marker. Int J Biochem Cell Biol. 81(Pt A):166–173. DOI: 10.1016/j.biocel.2016.09.009. PMID: 27640754.


50. Wang L, Zuo X, Xie K, Wei D. 2018; The role of CD44 and cancer stem cells. Methods Mol Biol. 1692:31–42. DOI: 10.1007/978-1-4939-7401-6_3. PMID: 28986884.


51. Yan Y, Zuo X, Wei D. 2015; Concise review: emerging role of CD44 in cancer stem cells: a promising biomarker and therapeutic target. Stem Cells Transl Med. 4:1033–1043. DOI: 10.5966/sctm.2015-0048. PMID: 26136504. PMCID: PMC4542874.


52. Tian S, Liu DH, Wang D, Ren F, Xia P. 2018; Aldehyde dehydrogenase 1 (ALDH1) promotes the toxicity of TRAIL in non-small cell lung cancer cells via post-transcriptional regulation of MEK-1 expression. Cell Physiol Biochem. 51:217–227. DOI: 10.1159/000495202. PMID: 30448845.


53. Clay MR, Tabor M, Owen JH, Carey TE, Bradford CR, Wolf GT, Wicha MS, Prince ME. 2010; Single-marker identification of head and neck squamous cell carcinoma cancer stem cells with aldehyde dehydrogenase. Head Neck. 32:1195–1201. DOI: 10.1002/hed.21315. PMID: 20073073. PMCID: PMC2991066.


54. Bertrand G, Maalouf M, Boivin A, Battiston-Montagne P, Beuve M, Levy A, Jalade P, Fournier C, Ardail D, Magné N, Alphonse G, Rodriguez-Lafrasse C. 2014; Targeting head and neck cancer stem cells to overcome resistance to photon and carbon ion radiation. Stem Cell Rev Rep. 10:114–126. DOI: 10.1007/s12015-013-9467-y. PMID: 23955575.


55. Cho K, Wang X, Nie S, Chen ZG, Shin DM. 2008; Therapeutic nanoparticles for drug delivery in cancer. Clin Cancer Res. 14:1310–1316. DOI: 10.1158/1078-0432.CCR-07-1441. PMID: 18316549.


56. Eramo A, Ricci-Vitiani L, Zeuner A, Pallini R, Lotti F, Sette G, Pilozzi E, Larocca LM, Peschle C, De Maria R. 2006; Chemotherapy resistance of glioblastoma stem cells. Cell Death Differ. 13:1238–1241. DOI: 10.1038/sj.cdd.4401872. PMID: 16456578.


57. Li X, Lewis MT, Huang J, Gutierrez C, Osborne CK, Wu MF, Hilsenbeck SG, Pavlick A, Zhang X, Chamness GC, Wong H, Rosen J, Chang JC. 2008; Intrinsic resistance of tumorigenic breast cancer cells to chemotherapy. J Natl Cancer Inst. 100:672–679. DOI: 10.1093/jnci/djn123. PMID: 18445819.


58. Dylla SJ, Beviglia L, Park IK, Chartier C, Raval J, Ngan L, Pickell K, Aguilar J, Lazetic S, Smith-Berdan S, Clarke MF, Hoey T, Lewicki J, Gurney AL. 2008; Colorectal cancer stem cells are enriched in xenogeneic tumors following chemotherapy. PLoS One. 3:e2428. DOI: 10.1371/journal.pone.0002428. PMID: 18560594. PMCID: PMC2413402.


59. Ishikawa F, Yoshida S, Saito Y, Hijikata A, Kitamura H, Tanaka S, Nakamura R, Tanaka T, Tomiyama H, Saito N, Fukata M, Miyamoto T, Lyons B, Ohshima K, Uchida N, Taniguchi S, Ohara O, Akashi K, Harada M, Shultz LD. 2007; Chemotherapy-resistant human AML stem cells home to and engraft within the bone-marrow endosteal region. Nat Biotechnol. 25:1315–1321. DOI: 10.1038/nbt1350. PMID: 17952057.


60. Frank NY, Margaryan A, Huang Y, Schatton T, Waaga-Gasser AM, Gasser M, Sayegh MH, Sadee W, Frank MH. 2005; ABCB5-mediated doxorubicin transport and chemoresistance in human malignant melanoma. Cancer Res. 65:4320–4333. DOI: 10.1158/0008-5472.CAN-04-3327. PMID: 15899824.


61. Bao S, Wu Q, McLendon RE, Hao Y, Shi Q, Hjelmeland AB, Dewhirst MW, Bigner DD, Rich JN. 2006; Glioma stem cells promote radioresistance by preferential activation of the DNA damage response. Nature. 444:756–760. DOI: 10.1038/nature05236. PMID: 17051156.


62. Phillips TM, McBride WH, Pajonk F. 2006; The response of CD24(-/low)/CD44+ breast cancer-initiating cells to radiation. J Natl Cancer Inst. 98:1777–1785. DOI: 10.1093/jnci/djj495. PMID: 17179479.
63. Gemenetzidis E, Gammon L, Biddle A, Emich H, Mackenzie IC. 2015; Invasive oral cancer stem cells display resistance to ionising radiation. Oncotarget. 6:43964–43977. DOI: 10.18632/oncotarget.6268. PMID: 26540568. PMCID: PMC4791279.


64. Chikamatsu K, Ishii H, Takahashi G, Okamoto A, Moriyama M, Sakakura K, Masuyama K. 2012; Resistance to apoptosis-inducing stimuli in CD44+ head and neck squamous cell carcinoma cells. Head Neck. 34:336–343. DOI: 10.1002/hed.21732. PMID: 21472882.


65. LaBarge MA. 2010; The difficulty of targeting cancer stem cell niches. Clin Cancer Res. 16:3121–3129. DOI: 10.1158/1078-0432.CCR-09-2933. PMID: 20530700. PMCID: PMC3182451.


66. Lacerda L, Pusztai L, Woodward WA. 2010; The role of tumor initiating cells in drug resistance of breast cancer: implications for future therapeutic approaches. Drug Resist Updat. 13:99–108. DOI: 10.1016/j.drup.2010.08.001. PMID: 20739212.


67. Wang Z, Li Y, Ahmad A, Azmi AS, Kong D, Banerjee S, Sarkar FH. 2010; Targeting miRNAs involved in cancer stem cell and EMT regulation: an emerging concept in overcoming drug resistance. Drug Resist Updat. 13:109–118. DOI: 10.1016/j.drup.2010.07.001. PMID: 20692200. PMCID: PMC2956795.


68. Dou J, Gu N. 2010; Emerging strategies for the identification and targeting of cancer stem cells. Tumour Biol. 31:243–253. DOI: 10.1007/s13277-010-0023-y. PMID: 20336402.


69. Kastan MB, Schlaffer E, Russo JE, Colvin OM, Civin CI, Hilton J. 1990; Direct demonstration of elevated aldehyde dehydrogenase in human hematopoietic progenitor cells. Blood. 75:1947–1950. DOI: 10.1182/blood.V75.10.1947.1947. PMID: 2337669.


70. Ginestier C, Hur MH, Charafe-Jauffret E, Monville F, Dutcher J, Brown M, Jacquemier J, Viens P, Kleer CG, Liu S, Schott A, Hayes D, Birnbaum D, Wicha MS, Dontu G. 2007; ALDH1 is a marker of normal and malignant human mammary stem cells and a predictor of poor clinical outcome. Cell Stem Cell. 1:555–567. DOI: 10.1016/j.stem.2007.08.014. PMID: 18371393. PMCID: PMC2423808.


71. Rasper M, Schäfer A, Piontek G, Teufel J, Brockhoff G, Ringel F, Heindl S, Zimmer C, Schlegel J. 2010; Aldehyde dehydrogenase 1 positive glioblastoma cells show brain tumor stem cell capacity. Neuro Oncol. 12:1024–1033. DOI: 10.1093/neuonc/noq070. PMID: 20627895. PMCID: PMC3018920.


72. Yu CC, Lo WL, Chen YW, Huang PI, Hsu HS, Tseng LM, Hung SC, Kao SY, Chang CJ, Chiou SH. 2011; Bmi-1 regulates snail expression and promotes metastasis ability in head and neck squamous cancer-derived ALDH1 positive cells. J Oncol. 2011:609259. DOI: 10.1155/2011/609259. PMID: 20936121. PMCID: PMC2948925.


73. Chen YC, Chang CJ, Hsu HS, Chen YW, Tai LK, Tseng LM, Chiou GY, Chang SC, Kao SY, Chiou SH, Lo WL. 2010; Inhibition of tumorigenicity and enhancement of radiochemosensitivity in head and neck squamous cell cancer-derived ALDH1-positive cells by knockdown of Bmi-1. Oral Oncol. 46:158–165. DOI: 10.1016/j.oraloncology.2009.11.007. PMID: 20036608.


74. Chen C, Zhao S, Karnad A, Freeman JW. 2018; The biology and role of CD44 in cancer progression: therapeutic implications. J Hematol Oncol. 11:64. DOI: 10.1186/s13045-018-0605-5. PMID: 29747682. PMCID: PMC5946470.


75. Orian-Rousseau V. 2010; CD44, a therapeutic target for metastasising tumours. Eur J Cancer. 46:1271–1277. DOI: 10.1016/j.ejca.2010.02.024. PMID: 20303742.


76. Chikamatsu K, Takahashi G, Sakakura K, Ferrone S, Masuyama K. 2011; Immunoregulatory properties of CD44+ cancer stem-like cells in squamous cell carcinoma of the head and neck. Head Neck. 33:208–215. DOI: 10.1002/hed.21420. PMID: 20848440. PMCID: PMC3426231.


77. Yu Q, Stamenkovic I. 1999; Localization of matrix metalloproteinase 9 to the cell surface provides a mechanism for CD44-mediated tumor invasion. Genes Dev. 13:35–48. DOI: 10.1101/gad.13.1.35. PMID: 9887098. PMCID: PMC316376.


78. Kim HR, Wheeler MA, Wilson CM, Iida J, Eng D, Simpson MA, McCarthy JB, Bullard KM. 2004; Hyaluronan facilitates invasion of colon carcinoma cells in vitro via interaction with CD44. Cancer Res. 64:4569–4576. DOI: 10.1158/0008-5472.CAN-04-0202. PMID: 15231668.


79. Golshani R, Lopez L, Estrella V, Kramer M, Iida N, Lokeshwar VB. 2008; Hyaluronic acid synthase-1 expression regulates bladder cancer growth, invasion, and angiogenesis through CD44. Cancer Res. 68:483–491. DOI: 10.1158/0008-5472.CAN-07-2140. PMID: 18199543.


80. Yu Q, Toole BP, Stamenkovic I. 1997; Induction of apoptosis of metastatic mammary carcinoma cells in vivo by disruption of tumor cell surface CD44 function. J Exp Med. 186:1985–1996. DOI: 10.1084/jem.186.12.1985. PMID: 9396767. PMCID: PMC2199167.


81. Feng D, Peng C, Li C, Zhou Y, Li M, Ling B, Wei H, Tian Z. 2009; Identification and characterization of cancer stem-like cells from primary carcinoma of the cervix uteri. Oncol Rep. 22:1129–1134. DOI: 10.3892/or_00000545. PMID: 19787230.


82. Dubrovska A, Kim S, Salamone RJ, Walker JR, Maira SM, García-Echeverría C, Schultz PG, Reddy VA. 2009; The role of PTEN/Akt/PI3K signaling in the maintenance and viability of prostate cancer stem-like cell populations. Proc Natl Acad Sci U S A. 106:268–273. DOI: 10.1073/pnas.0810956106. PMID: 19116269. PMCID: PMC2629188.


83. Leung EL, Fiscus RR, Tung JW, Tin VP, Cheng LC, Sihoe AD, Fink LM, Ma Y, Wong MP. 2010; Non-small cell lung cancer cells expressing CD44 are enriched for stem cell-like properties. PLoS One. 5:e14062. DOI: 10.1371/journal.pone.0014062. PMID: 21124918. PMCID: PMC2988826.


84. Haraguchi N, Ohkuma M, Sakashita H, Matsuzaki S, Tanaka F, Mimori K, Kamohara Y, Inoue H, Mori M. 2008; CD133+CD44+ population efficiently enriches colon cancer initiating cells. Ann Surg Oncol. 15:2927–2933. DOI: 10.1245/s10434-008-0074-0. PMID: 18663533.


85. Zhang S, Balch C, Chan MW, Lai HC, Matei D, Schilder JM, Yan PS, Huang TH, Nephew KP. 2008; Identification and characterization of ovarian cancer-initiating cells from primary human tumors. Cancer Res. 68:4311–4320. DOI: 10.1158/0008-5472.CAN-08-0364. PMID: 18519691. PMCID: PMC2553722.


86. Takaishi S, Okumura T, Tu S, Wang SS, Shibata W, Vigneshwaran R, Gordon SA, Shimada Y, Wang TC. 2009; Identification of gastric cancer stem cells using the cell surface marker CD44. Stem Cells. 27:1006–1020. DOI: 10.1002/stem.30. PMID: 19415765. PMCID: PMC2746367.


87. Yang YM, Chang JW. 2008; Bladder cancer initiating cells (BCICs) are among EMA-CD44v6+ subset: novel methods for isolating undetermined cancer stem (initiating) cells. Cancer Invest. 26:725–733. DOI: 10.1080/07357900801941845. PMID: 18608209.


88. Lee CJ, Dosch J, Simeone DM. 2008; Pancreatic cancer stem cells. J Clin Oncol. 26:2806–2812. DOI: 10.1200/JCO.2008.16.6702. PMID: 18539958.


89. Boxberg M, Götz C, Haidari S, Dorfner C, Jesinghaus M, Drecoll E, Boskov M, Wolff KD, Weichert W, Haller B, Kolk A. 2018; Immunohistochemical expression of CD44 in oral squamous cell carcinoma in relation to histomorphological parameters and clinicopathological factors. Histopathology. 73:559–572. DOI: 10.1111/his.13496. PMID: 29468726.


90. Ortiz RC, Lopes NM, Amôr NG, Ponce JB, Schmerling CK, Lara VS, Moyses RA, Rodini CO. 2018; CD44 and ALDH1 immunoexpression as prognostic indicators of invasion and metastasis in oral squamous cell carcinoma. J Oral Pathol Med. 47:740–747. DOI: 10.1111/jop.12734. PMID: 29791975.


91. Wang SJ, Wong G, de Heer AM, Xia W, Bourguignon LY. 2009; CD44 variant isoforms in head and neck squamous cell carcinoma progression. Laryngoscope. 119:1518–1530. DOI: 10.1002/lary.20506. PMID: 19507218. PMCID: PMC2718060.


92. Joshua B, Kaplan MJ, Doweck I, Pai R, Weissman IL, Prince ME, Ailles LE. 2012; Frequency of cells expressing CD44, a head and neck cancer stem cell marker: correlation with tumor aggressiveness. Head Neck. 34:42–49. DOI: 10.1002/hed.21699. PMID: 21322081.


93. Oliveira LR, Oliveira-Costa JP, Araujo IM, Soave DF, Zanetti JS, Soares FA, Zucoloto S, Ribeiro-Silva A. 2011; Cancer stem cell immunophenotypes in oral squamous cell carcinoma. J Oral Pathol Med. 40:135–142. DOI: 10.1111/j.1600-0714.2010.00967.x. PMID: 21073537.


94. Su J, Xu XH, Huang Q, Lu MQ, Li DJ, Xue F, Yi F, Ren JH, Wu YP. 2011; Identification of cancer stem-like CD44+ cells in human nasopharyngeal carcinoma cell line. Arch Med Res. 42:15–21. DOI: 10.1016/j.arcmed.2011.01.007. PMID: 21376257.


95. Gammon L, Biddle A, Fazil B, Harper L, Mackenzie IC. 2011; Stem cell characteristics of cell sub-populations in cell lines derived from head and neck cancers of Fanconi anemia patients. J Oral Pathol Med. 40:143–152. DOI: 10.1111/j.1600-0714.2010.00972.x. PMID: 21138479.


96. Harper LJ, Piper K, Common J, Fortune F, Mackenzie IC. 2007; Stem cell patterns in cell lines derived from head and neck squamous cell carcinoma. J Oral Pathol Med. 36:594–603. DOI: 10.1111/j.1600-0714.2007.00617.x. PMID: 17944752.


97. Corbeil D, Marzesco AM, Wilsch-Bräuninger M, Huttner WB. 2010; The intriguing links between prominin-1 (CD133), cholesterol-based membrane microdomains, remodeling of apical plasma membrane protrusions, extracellular membrane particles, and (neuro)epithelial cell differentiation. FEBS Lett. 584:1659–1664. DOI: 10.1016/j.febslet.2010.01.050. PMID: 20122930.


98. Suetsugu A, Nagaki M, Aoki H, Motohashi T, Kunisada T, Moriwaki H. 2006; Characterization of CD133+ hepatocellular carcinoma cells as cancer stem/progenitor cells. Biochem Biophys Res Commun. 351:820–824. DOI: 10.1016/j.bbrc.2006.10.128. PMID: 17097610.


99. Yu CC, Hu FW, Yu CH, Chou MY. 2016; Targeting CD133 in the enhancement of chemosensitivity in oral squamous cell carcinoma-derived side population cancer stem cells. Head Neck. 38 Suppl 1:E231–E238. DOI: 10.1002/hed.23975. PMID: 25545959.


100. Vander Griend DJ, Karthaus WL, Dalrymple S, Meeker A, DeMarzo AM, Isaacs JT. 2008; The role of CD133 in normal human prostate stem cells and malignant cancer-initiating cells. Cancer Res. 68:9703–9711. DOI: 10.1158/0008-5472.CAN-08-3084. PMID: 19047148. PMCID: PMC3072758.


101. Kim K, Ihm H, Ro JY, Cho YM. 2011; High-level expression of stem cell marker CD133 in clear cell renal cell carcinoma with favorable prognosis. Oncol Lett. 2:1095–1100. DOI: 10.1158/1538-7445.AM2011-499. PMID: 22848273. PMCID: PMC3406563.


102. Bi Y, Meng Y, Wu H, Cui Q, Luo Y, Xue X. 2016; Expression of the potential cancer stem cell markers CD133 and CD44 in medullary thyroid carcinoma: a ten-year follow-up and prognostic analysis. J Surg Oncol. 113:144–151. DOI: 10.1002/jso.24124. PMID: 26799258.


103. Zhou L, Wei X, Cheng L, Tian J, Jiang JJ. 2007; CD133, one of the markers of cancer stem cells in Hep-2 cell line. Laryngoscope. 117:455–460. DOI: 10.1097/01.mlg.0000251586.15299.35. PMID: 17334305.


104. Chiou SH, Yu CC, Huang CY, Lin SC, Liu CJ, Tsai TH, Chou SH, Chien CS, Ku HH, Lo JF. 2008; Positive correlations of Oct-4 and Nanog in oral cancer stem-like cells and high-grade oral squamous cell carcinoma. Clin Cancer Res. 14:4085–4095. DOI: 10.1158/1078-0432.CCR-07-4404. PMID: 18593985.


105. Oliveira LR, Castilho-Fernandes A, Oliveira-Costa JP, Soares FA, Zucoloto S, Ribeiro-Silva A. 2014; CD44+/CD133+ immunophenotype and matrix metalloproteinase-9: influence on prognosis in early-stage oral squamous cell carcinoma. Head Neck. 36:1718–1726. DOI: 10.1002/hed.23527. PMID: 24178866.


106. An N, Cen B, Cai H, Song JH, Kraft A, Kang Y. 2016; Pim1 kinase regulates c-Kit gene translation. Exp Hematol Oncol. 5:31. DOI: 10.1186/s40164-016-0060-3. PMID: 28042518. PMCID: PMC5200960.


107. Kimura Y, Ding B, Imai N, Nolan DJ, Butler JM, Rafii S. 2011; c-Kit-mediated functional positioning of stem cells to their niches is essential for maintenance and regeneration of adult hematopoiesis. PLoS One. 6:e26918. DOI: 10.1371/journal.pone.0026918. PMID: 22046410. PMCID: PMC3202594.


108. Zhan Q, Wang C, Ngai S. 2013; Ovarian cancer stem cells: a new target for cancer therapy. Biomed Res Int. 2013:916819. DOI: 10.1155/2013/916819. PMID: 23509802. PMCID: PMC3581273.


109. Miettinen M, Lasota J. 2005; KIT (CD117): a review on expression in normal and neoplastic tissues, and mutations and their clinicopathologic correlation. Appl Immunohis-tochem Mol Morphol. 13:205–220. DOI: 10.1097/01.pai.0000173054.83414.22. PMID: 16082245.


110. Bolante-Cervantes R, Li S, Sahota A, Tischfield JA, Zwerdling T, Stambrook PJ. 1999; Pattern of localization of primitive hematopoietic cells in vivo using a novel mouse model. Exp Hematol. 27:1346–1352. DOI: 10.1016/S0301-472X(99)00064-8. PMID: 10428512.


111. Hines SJ, Organ C, Kornstein MJ, Krystal GW. 1995; Coex-pression of the c-kit and stem cell factor genes in breast carcinomas. Cell Growth Differ. 6:769–779. PMID: 7545433.
112. Hirota S, Isozaki K, Moriyama Y, Hashimoto K, Nishida T, Ishiguro S, Kawano K, Hanada M, Kurata A, Takeda M, Muhammad Tunio G, Matsuzawa Y, Kanakura Y, Shinomura Y, Kitamura Y. 1998; Gain-of-function mutations of c-kit in human gastrointestinal stromal tumors. Science. 279:577–580. DOI: 10.1126/science.279.5350.577. PMID: 9438854.


113. Strohmeyer T, Peter S, Hartmann M, Munemitsu S, Ackermann R, Ullrich A, Slamon DJ. 1991; Expression of the hst-1 and c-kit protooncogenes in human testicular germ cell tumors. Cancer Res. 51:1811–1816. PMID: 1706218.
114. Jeng YM, Lin CY, Hsu HC. 2000; Expression of the c-kit protein is associated with certain subtypes of salivary gland carcinoma. Cancer Lett. 154:107–111. DOI: 10.1016/S0304-3835(00)00387-6. PMID: 10799746.


115. Sekido Y, Obata Y, Ueda R, Hida T, Suyama M, Shimokata K, Ariyoshi Y, Takahashi T. 1991; Preferential expression of c-kit protooncogene transcripts in small cell lung cancer. Cancer Res. 51:2416–2419. PMID: 1707753.
116. Ongkeko WM, Altuna X, Weisman RA, Wang-Rodriguez J. 2005; Expression of protein tyrosine kinases in head and neck squamous cell carcinomas. Am J Clin Pathol. 124:71–76. DOI: 10.1309/BTLN5WTMJ3PCNRRC. PMID: 15923166.


117. Tsai LL, Yu CC, Lo JF, Sung WW, Lee H, Chen SL, Chou MY. 2012; Enhanced cisplatin resistance in oral-cancer stem-like cells is correlated with upregulation of excision-repair cross-complementation group 1. J Dent Sci. 7:111–117. DOI: 10.1016/j.jds.2012.03.006.


118. Mărgăritescu C, Pirici D, Simionescu C, Stepan A. 2011; The utility of CD44, CD117 and CD133 in identification of cancer stem cells (CSC) in oral squamous cell carcinomas (OSCC). Rom J Morphol Embryol. 52(3 Suppl):985–993. PMID: 22119814.
119. Silva Galbiatti-Dias AL, Fernandes GMM, Castanhole-Nunes MMU, Hidalgo LF, Nascimento Filho CHV, Kawasaki-Oyama RS, Ferreira LAM, Biselli-Chicote PM, Pavarino ÉC, Goloni-Bertollo EM. 2018; Relationship between CD44high/CD133high/CD117high cancer stem cells phenotype and Cetuximab and Paclitaxel treatment response in head and neck cancer cell lines. Am J Cancer Res. 8:1633–1641. PMID: 30210931. PMCID: PMC6129494.
120. Barth PJ, Schenck zu Schweinsberg T, Ramaswamy A, Moll R. 2004; CD34+ fibrocytes, alpha-smooth muscle antigen-positive myofibroblasts, and CD117 expression in the stroma of invasive squamous cell carcinomas of the oral cavity, pharynx, and larynx. Virchows Arch. 444:231–234. DOI: 10.1007/s00428-003-0965-1. PMID: 14758552.


121. Hill RP, Marie-Egyptienne DT, Hedley DW. 2009; Cancer stem cells, hypoxia and metastasis. Semin Radiat Oncol. 19:106–111. DOI: 10.1016/j.semradonc.2008.12.002. PMID: 19249648.


122. Kim WT, Ryu CJ. 2017; Cancer stem cell surface markers on normal stem cells. BMB Rep. 50:285–298. DOI: 10.5483/BMBRep.2017.50.6.039. PMID: 28270302. PMCID: PMC5498139.


123. Gupta PB, Chaffer CL, Weinberg RA. 2009; Cancer stem cells: mirage or reality? Nat Med. 15:1010–1012. DOI: 10.1038/nm0909-1010. PMID: 19734877.


124. Alok A, Lei Z, Jagannathan NS, Kaur S, Harmston N, Rozen SG, Tucker-Kellogg L, Virshup DM. 2017; Wnt proteins synergize to activate β-catenin signaling. J Cell Sci. 130:1532–1544. DOI: 10.1242/dev.154112. PMID: 28289266.


125. Routledge D, Scholpp S. 2019; Mechanisms of intercellular Wnt transport. Development. 146:dev176073. DOI: 10.1242/dev.176073. PMID: 31092504.


126. Luis TC, Ichii M, Brugman MH, Kincade P, Staal FJ. 2012; Wnt signaling strength regulates normal hematopoiesis and its deregulation is involved in leukemia development. Leukemia. 26:414–421. DOI: 10.1038/leu.2011.387. PMID: 22173215. PMCID: PMC3378318.


127. Jang GB, Hong IS, Kim RJ, Lee SY, Park SJ, Lee ES, Park JH, Yun CH, Chung JU, Lee KJ, Lee HY, Nam JS. 2015; Wnt/β-catenin small-molecule inhibitor CWP232228 preferentially inhibits the growth of breast cancer stem-like cells. Cancer Res. 75:1691–1702. DOI: 10.1158/0008-5472.CAN-14-2041. PMID: 25660951.


128. Jang GB, Kim JY, Cho SD, Park KS, Jung JY, Lee HY, Hong IS, Nam JS. 2015; Blockade of Wnt/β-catenin signaling suppresses breast cancer metastasis by inhibiting CSC-like phenotype. Sci Rep. 5:12465. DOI: 10.1038/srep12465. PMID: 26202299. PMCID: PMC5378883.


129. Gedaly R, Galuppo R, Daily MF, Shah M, Maynard E, Chen C, Zhang X, Esser KA, Cohen DA, Evers BM, Jiang J, Spear BT. 2014; Targeting the Wnt/β-catenin signaling pathway in liver cancer stem cells and hepatocellular carcinoma cell lines with FH535. PLoS One. 9:e99272. DOI: 10.1371/journal.pone.0099272. PMID: 24940873. PMCID: PMC4062395.


130. Teng Y, Wang X, Wang Y, Ma D. 2010; Wnt/beta-catenin signaling regulates cancer stem cells in lung cancer A549 cells. Biochem Biophys Res Commun. 392:373–379. DOI: 10.1016/j.bbrc.2010.01.028. PMID: 20074550.


131. Iwai S, Yonekawa A, Harada C, Hamada M, Katagiri W, Nakazawa M, Yura Y. 2010; Involvement of the Wnt-β-catenin pathway in invasion and migration of oral squamous carcinoma cells. Int J Oncol. 37:1095–1103. DOI: 10.3892/ijo_00000761. PMID: 20878057.


132. Yang F, Zeng Q, Yu G, Li S, Wang CY. 2006; Wnt/beta-catenin signaling inhibits death receptor-mediated apoptosis and promotes invasive growth of HNSCC. Cell Signal. 18:679–687. DOI: 10.1016/j.cellsig.2005.06.015. PMID: 16084063.


133. Warrier S, Bhuvanalakshmi G, Arfuso F, Rajan G, Millward M, Dharmarajan A. 2014; Cancer stem-like cells from head and neck cancers are chemosensitized by the Wnt antagonist, sFRP4, by inducing apoptosis, decreasing stemness, drug resistance and epithelial to mesenchymal transition. Cancer Gene Ther. 21:381–388. DOI: 10.1038/cgt.2014.42. PMID: 25104726.


134. Lim YC, Kang HJ, Kim YS, Choi EC. 2012; All-trans-retinoic acid inhibits growth of head and neck cancer stem cells by suppression of Wnt/β-catenin pathway. Eur J Cancer. 48:3310–3318. DOI: 10.1016/j.ejca.2012.04.013. PMID: 22640830.


135. Yin L, Velazquez OC, Liu ZJ. 2010; Notch signaling: emerging molecular targets for cancer therapy. Biochem Pharmacol. 80:690–701. DOI: 10.1016/j.bcp.2010.03.026. PMID: 20361945.


136. Penton AL, Leonard LD, Spinner NB. 2012; Notch signaling in human development and disease. Semin Cell Dev Biol. 23:450–457. DOI: 10.1016/j.semcdb.2012.01.010. PMID: 22306179. PMCID: PMC3638987.


137. Kovall RA, Gebelein B, Sprinzak D, Kopan R. 2017; The canonical Notch signaling pathway: structural and biochemical insights into shape, sugar, and force. Dev Cell. 41:228–241. DOI: 10.1016/j.devcel.2017.04.001. PMID: 28486129. PMCID: PMC5492985.


138. Aster JC, Pear WS, Blacklow SC. 2017; The varied roles of Notch in cancer. Annu Rev Pathol. 12:245–275. DOI: 10.1146/annurev-pathol-052016-100127. PMID: 27959635. PMCID: PMC5933931.


139. Allenspach EJ, Maillard I, Aster JC, Pear WS. 2002; Notch signaling in cancer. Cancer Biol Ther. 1:466–476. DOI: 10.4161/cbt.1.5.159. PMID: 12496471.


140. Colombo M, Mirandola L, Chiriva-Internati M, Basile A, Locati M, Lesma E, Chiaramonte R, Platonova N. 2018; Cancer cells exploit Notch signaling to redefine a supportive cytokine milieu. Front Immunol. 9:1823. DOI: 10.3389/fimmu.2018.01823. PMID: 30154786. PMCID: PMC6102368.


141. Agrawal N, Frederick MJ, Pickering CR, Bettegowda C, Chang K, Li RJ, Fakhry C, Xie TX, Zhang J, Wang J, Zhang N, El-Naggar AK, Jasser SA, Weinstein JN, Treviño L, Drummond JA, Muzny DM, Wu Y, Wood LD, Hruban RH, Westra WH, Koch WM, Califano JA, Gibbs RA, Sidransky D, Vogelstein B, Velculescu VE, Papadopoulos N, Wheeler DA, Kinzler KW, Myers JN. 2011; Exome sequencing of head and neck squamous cell carcinoma reveals inactivating mutations in NOTCH1. Science. 333:1154–1157. DOI: 10.1126/science.1206923. PMID: 21798897. PMCID: PMC3162986.


142. Stransky N, Egloff AM, Tward AD, Kostic AD, Cibulskis K, Sivachenko A, Kryukov GV, Lawrence MS, Sougnez C, McKenna A, Shefler E, Ramos AH, Stojanov P, Carter SL, Voet D, Cortés ML, Auclair D, Berger MF, Saksena G, Guiducci C, Onofrio RC, Parkin M, Romkes M, Weissfeld JL, Seethala RR, Wang L, Rangel-Escareño C, Fernandez-Lopez JC, Hidalgo-Miranda A, Melendez-Zajgla J, Winckler W, Ardlie K, Gabriel SB, Meyerson M, Lander ES, Getz G, Golub TR, Garraway LA, Grandis JR. 2011; The mutational landscape of head and neck squamous cell carcinoma. Science. 333:1157–1160. DOI: 10.1126/science.1208130. PMID: 21798893. PMCID: PMC3415217.


143. Sun W, Gaykalova DA, Ochs MF, Mambo E, Arnaoutakis D, Liu Y, Loyo M, Agrawal N, Howard J, Li R, Ahn S, Fertig E, Sidransky D, Houghton J, Buddavarapu K, Sanford T, Choudhary A, Darden W, Adai A, Latham G, Bishop J, Sharma R, Westra WH, Hennessey P, Chung CH, Califano JA. 2014; Activation of the NOTCH pathway in head and neck cancer. Cancer Res. 74:1091–1104. DOI: 10.1158/0008-5472.CAN-13-1259. PMID: 24351288. PMCID: PMC3944644.


144. Shrivastava S, Steele R, Sowadski M, Crawford SE, Varvares M, Ray RB. 2015; Identification of molecular signature of head and neck cancer stem-like cells. Sci Rep. 5:7819. DOI: 10.1038/srep07819. PMID: 25588898. PMCID: PMC4295111.


145. Lee SH, Hong HS, Liu ZX, Kim RH, Kang MK, Park NH, Shin KH. 2012; TNFα enhances cancer stem cell-like phenotype via Notch-Hes1 activation in oral squamous cell carcinoma cells. Biochem Biophys Res Commun. 424:58–64. DOI: 10.1016/j.bbrc.2012.06.065. PMID: 22728043. PMCID: PMC3488595.


146. Zou B, Sun S, Qi X, Ji P. 2012; Aldehyde dehydrogenase activity is a cancer stem cell marker of tongue squamous cell carcinoma. Mol Med Rep. 5:1116–1120. DOI: 10.3892/mmr.2012.781. PMID: 22307065. PMCID: PMC3493091.


147. Sasai N, Toriyama M, Kondo T. 2019; Hedgehog signal and genetic disorders. Front Genet. 10:1103. DOI: 10.3389/fgene.2019.01103. PMID: 31781166. PMCID: PMC6856222.


148. Abramyan J. 2019; Hedgehog signaling and embryonic craniofacial disorders. J Dev Biol. 7:9. DOI: 10.3390/jdb7020009. PMID: 31022843. PMCID: PMC6631594.


149. Nüsslein-Volhard C, Wieschaus E. 1980; Mutations affecting segment number and polarity in Drosophila. Nature. 287:795–801. DOI: 10.1038/287795a0. PMID: 6776413.


150. Lee JJ, von Kessler DP, Parks S, Beachy PA. 1992; Secretion and localized transcription suggest a role in positional signaling for products of the segmentation gene hedgehog. Cell. 71:33–50. DOI: 10.1016/0092-8674(92)90264-D. PMID: 1394430.


151. Mohler J, Vani K. 1992; Molecular organization and embryonic expression of the hedgehog gene involved in cell-cell communication in segmental patterning of Drosophila. Development. 115:957–971. DOI: 10.1242/dev.115.4.957. PMID: 1280560.


152. Tabata T, Eaton S, Kornberg TB. 1992; The Drosophila hedgehog gene is expressed specifically in posterior compartment cells and is a target of engrailed regulation. Genes Dev. 6:2635–2645. DOI: 10.1101/gad.6.12b.2635. PMID: 1340474.


153. Echelard Y, Epstein DJ, St-Jacques B, Shen L, Mohler J, McMahon JA, McMahon AP. 1993; Sonic hedgehog, a member of a family of putative signaling molecules, is implicated in the regulation of CNS polarity. Cell. 75:1417–1430. DOI: 10.1016/0092-8674(93)90627-3. PMID: 7916661.


154. Krauss S, Concordet JP, Ingham PW. 1993; A functionally conserved homolog of the Drosophila segment polarity gene hh is expressed in tissues with polarizing activity in zebrafish embryos. Cell. 75:1431–1444. DOI: 10.1016/0092-8674(93)90628-4. PMID: 8269519.


155. Marigo V, Tabin CJ. 1996; Regulation of patched by sonic hedgehog in the developing neural tube. Proc Natl Acad Sci U S A. 93:9346–9351. DOI: 10.1073/pnas.93.18.9346. PMID: 8790332. PMCID: PMC38430.


156. Robbins DJ, Fei DL, Riobo NA. 2012; The Hedgehog signal transduction network. Sci Signal. 5:re6. DOI: 10.1126/scisignal.2002906. PMID: 23074268. PMCID: PMC3705708.


157. Kasper M, Jaks V, Fiaschi M, Toftgård R. 2009; Hedgehog signalling in breast cancer. Carcinogenesis. 30:903–911. DOI: 10.1093/carcin/bgp048. PMID: 19237605. PMCID: PMC2691147.


158. Jagani Z, Dorsch M, Warmuth M. 2010; Hedgehog pathway activation in chronic myeloid leukemia. Cell Cycle. 9:3449–3456. DOI: 10.4161/cc.9.17.12945. PMID: 20928937.


159. Wu C, Zhu X, Liu W, Ruan T, Tao K. 2017; Hedgehog signaling pathway in colorectal cancer: function, mechanism, and therapy. Onco Targets Ther. 10:3249–3259. DOI: 10.2147/OTT.S139639. PMID: 28721076. PMCID: PMC5501640.


160. Takezaki T, Hide T, Takanaga H, Nakamura H, Kuratsu J, Kondo T. 2011; Essential role of the Hedgehog signaling pathway in human glioma-initiating cells. Cancer Sci. 102:1306–1312. DOI: 10.1111/j.1349-7006.2011.01943.x. PMID: 21453386.


161. Abe Y, Tanaka N. 2016; The hedgehog signaling networks in lung cancer: the mechanisms and roles in tumor progression and implications for cancer therapy. Biomed Res Int. 2016:7969286. DOI: 10.1155/2016/7969286. PMID: 28105432. PMCID: PMC5220431.


162. Peacock CD, Wang Q, Gesell GS, Corcoran-Schwartz IM, Jones E, Kim J, Devereux WL, Rhodes JT, Huff CA, Beachy PA, Watkins DN, Matsui W. 2007; Hedgehog signaling maintains a tumor stem cell compartment in multiple myeloma. Proc Natl Acad Sci U S A. 104:4048–4053. DOI: 10.1073/pnas.0611682104. PMID: 17360475. PMCID: PMC1805487.


163. Kelleher FC. 2011; Hedgehog signaling and therapeutics in pancreatic cancer. Carcinogenesis. 32:445–451. DOI: 10.1093/carcin/bgq280. PMID: 21186299.


164. Takebe N, Harris PJ, Warren RQ, Ivy SP. 2011; Targeting cancer stem cells by inhibiting Wnt, Notch, and Hedgehog pathways. Nat Rev Clin Oncol. 8:97–106. DOI: 10.1038/nrclinonc.2010.196. PMID: 21151206.


165. Wang YF, Chang CJ, Lin CP, Chang SY, Chu PY, Tai SK, Li WY, Chao KS, Chen YJ. 2012; Expression of hedgehog signaling molecules as a prognostic indicator of oral squamous cell carcinoma. Head Neck. 34:1556–1561. DOI: 10.1002/hed.21958. PMID: 22287313.


166. Han Y. 2019; Analysis of the role of the Hippo pathway in cancer. J Transl Med. 17:116. DOI: 10.1186/s12967-019-1869-4. PMID: 30961610. PMCID: PMC6454697.


167. Zhao C, Zeng C, Ye S, Dai X, He Q, Yang B, Zhu H. 2020; Yes-associated protein (YAP) and transcriptional coacti-vator with a PDZ-binding motif (TAZ): a nexus between hypoxia and cancer. Acta Pharm Sin B. 10:947–960. DOI: 10.1016/j.apsb.2019.12.010. PMID: 32642404. PMCID: PMC7332664.


168. Lian I, Kim J, Okazawa H, Zhao J, Zhao B, Yu J, Chinnaiyan A, Israel MA, Goldstein LS, Abujarour R, Ding S, Guan KL. 2010; The role of YAP transcription coactivator in regulating stem cell self-renewal and differentiation. Genes Dev. 24:1106–1118. DOI: 10.1101/gad.1903310. PMID: 20516196. PMCID: PMC2878649.


169. Tamm C, Böwer N, Annerén C. 2011; Regulation of mouse embryonic stem cell self-renewal by a Yes-YAP-TEAD2 signaling pathway downstream of LIF. J Cell Sci. 124(Pt 7):1136–1144. DOI: 10.1242/jcs.075796. PMID: 21385842.


170. Segrelles C, Paramio JM, Lorz C. 2018; The transcriptional co-activator YAP: a new player in head and neck cancer. Oral Oncol. 86:25–32. DOI: 10.1016/j.oraloncology.2018.08.020. PMID: 30409308.


171. Santos-de-Frutos K, Segrelles C, Lorz C. 2019; Hippo pathway and YAP signaling alterations in squamous cancer of the head and neck. J Clin Med. 8:2131. DOI: 10.3390/jcm8122131. PMID: 31817001. PMCID: PMC6947155.


172. Eun YG, Lee D, Lee YC, Sohn BH, Kim EH, Yim SY, Kwon KH, Lee JS. 2017; Clinical significance of YAP1 activation in head and neck squamous cell carcinoma. Oncotarget. 8:111130–111143. DOI: 10.18632/oncotarget.22666. PMID: 29340043. PMCID: PMC5762311.


173. Hiemer SE, Zhang L, Kartha VK, Packer TS, Almershed M, Noonan V, Kukuruzinska M, Bais MV, Monti S, Varelas X. 2015; A YAP/TAZ-regulated molecular signature is associated with oral squamous cell carcinoma. Mol Cancer Res. 13:957–968. DOI: 10.1158/1541-7786.MCR-14-0580. PMID: 25794680. PMCID: PMC4470857.


174. Omori H, Nishio M, Masuda M, Miyachi Y, Ueda F, Nakano T, Sato K, Mimori K, Taguchi K, Hikasa H, Nishina H, Tashiro H, Kiyono T, Mak TW, Nakao K, Nakagawa T, Maehama T, Suzuki A. 2020; YAP1 is a potent driver of the onset and progression of oral squamous cell carcinoma. Sci Adv. 6:eaay3324. DOI: 10.1126/sciadv.aay3324. PMID: 32206709. PMCID: PMC7080500.


175. Li J, Li Z, Wu Y, Wang Y, Wang D, Zhang W, Yuan H, Ye J, Song X, Yang J, Jiang H, Cheng J. 2019; The Hippo effector TAZ promotes cancer stemness by transcriptional activation of SOX2 in head neck squamous cell carcinoma. Cell Death Dis. 10:603. DOI: 10.1038/s41419-019-1838-0. PMID: 31399556. PMCID: PMC6689034.


176. Bora-Singhal N, Nguyen J, Schaal C, Perumal D, Singh S, Coppola D, Chellappan S. 2015; YAP1 regulates OCT4 activity and SOX2 expression to facilitate self-renewal and vascular mimicry of stem-like cells. Stem Cells. 33:1705–1718. DOI: 10.1002/stem.1993. PMID: 25754111. PMCID: PMC4441573.


177. Sayed SI, Dwivedi RC, Katna R, Garg A, Pathak KA, Nutting CM, Rhys-Evans P, Harrington KJ, Kazi R. 2011; Implications of understanding cancer stem cell (CSC) biology in head and neck squamous cell cancer. Oral Oncol. 47:237–243. DOI: 10.1016/j.oraloncology.2011.02.009. PMID: 21382740.


178. Forastiere A, Koch W, Trotti A, Sidransky D. 2001; Head and neck cancer. N Engl J Med. 345:1890–1900. DOI: 10.1056/NEJMra001375. PMID: 11756581.


179. Chen YW, Chen KH, Huang PI, Chen YC, Chiou GY, Lo WL, Tseng LM, Hsu HS, Chang KW, Chiou SH. 2010; Cucurbitacin I suppressed stem-like property and enhanced radiation-induced apoptosis in head and neck squamous carcinoma--derived CD44(+)ALDH1(+) cells. Mol Cancer Ther. 9:2879–2892. DOI: 10.1158/1535-7163.MCT-10-0504. PMID: 21062915.


180. Shakib K, Schrattenholz A, Soskic V. 2011; Stem cells in head and neck squamous cell carcinoma. Br J Oral Maxillofac Surg. 49:503–506. DOI: 10.1016/j.bjoms.2010.07.016. PMID: 20832149.


181. Klonisch T, Wiechec E, Hombach-Klonisch S, Ande SR, Wesselborg S, Schulze-Osthoff K, Los M. 2008; Cancer stem cell markers in common cancers - therapeutic implications. Trends Mol Med. 14:450–460. DOI: 10.1016/j.molmed.2008.08.003. PMID: 18775674.


182. Yang YM, Chang JW. 2008; Current status and issues in cancer stem cell study. Cancer Invest. 26:741–755. DOI: 10.1080/07357900801901856. PMID: 18608212.


Fig. 1
Schematic diagram summarizing the therapeutic strategies targeting oral CSCs. The diverse phenotypic heterogeneity and plasticity among cancer cells in bulk tumors have been explained by the CSC model of hierarchically organized tumors. Unlike bulk tumor cells, CSCs are intrinsically resistant to the majority of conventional chemotherapeutic agents; thus, the remaining CSC subpopulation can subsequently reconstitute tumors in patients with cellular heterogeneity.
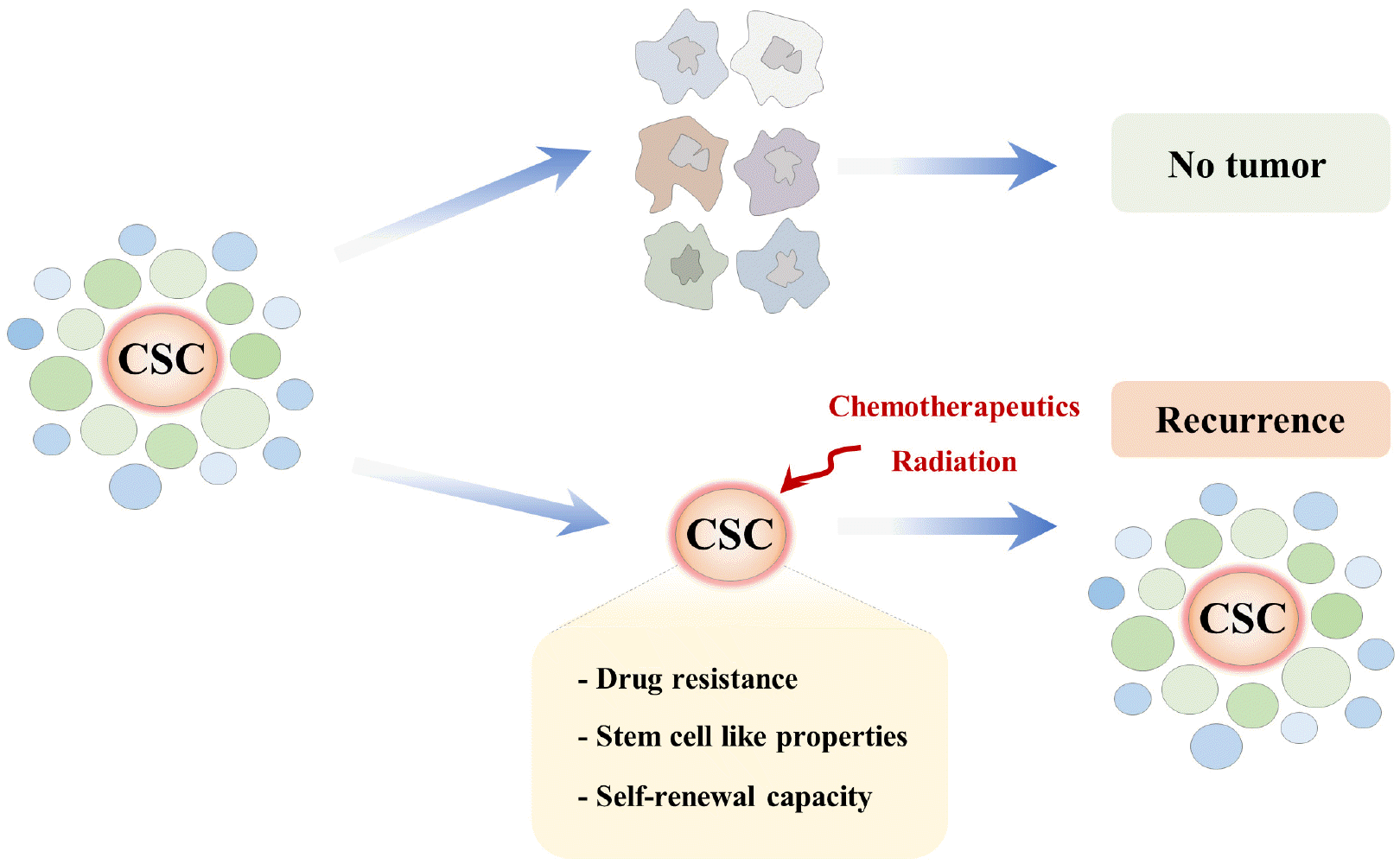
Fig. 2
Schematic diagram summarizing the therapeutic strategies targeting oral CSC-specific surface markers or signaling pathways. Therapeutic efficiency could be enhanced by selectively targeting subtle expression differences in surface markers and alterations in various signaling regulators between CSC subpopulations and non-CSC subpopulations. For this reason, CSC markers can be used to identify CSC-enriched subpopulations and various therapeutic approaches effectively inhibit oral CSCs by targeting pluripotency-associated genes (ALDHs, CD44, CD133, and CD177) and CSC-specific signaling pathways (Wnt/β-catenin, Notch, and hedgehog signaling pathways).
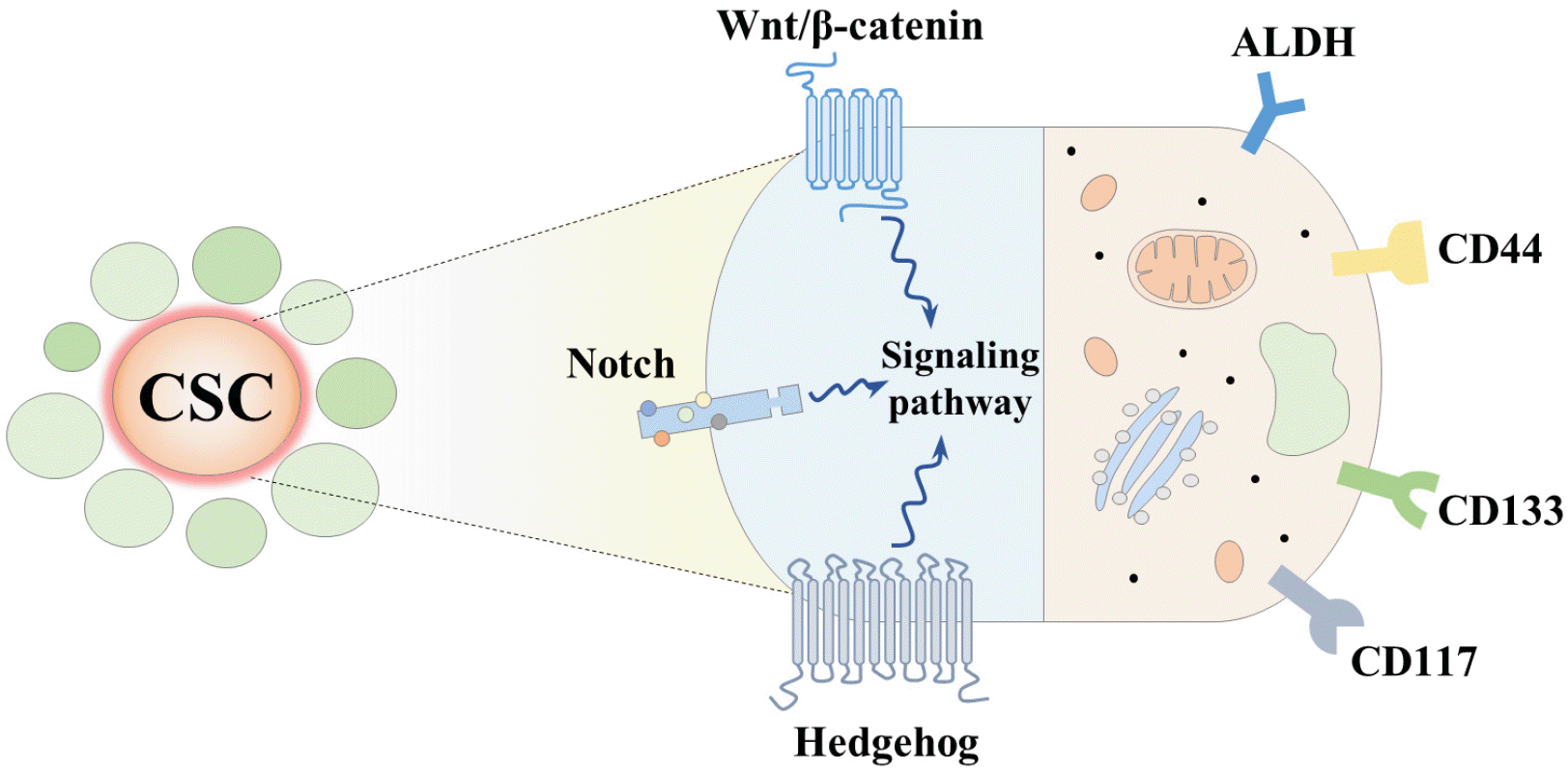