Abstract
Cancer is increasingly apparent as a systems-level, network happening. The central tendency of malignant alteration can be described as a two-phase procedure, where an initial increase of network plasticity is followed by reducing plasticity at late stages of tumor improvement. Cancer stem cells (CSCs) are cancer cells that take characteristics associated with normal stem cells. Cancer therapy has been based on the concept that most of the cancer cells have a similar ability to separate metastasise and kill the host. In this review, we addressed the use of nanotechnology in the treatment of cancer stem cells.
Progress in preclinical and clinical cancer research has brought new diagnostic and therapeutic options for cancer patients that would provide significant improvements in the treatment and prevention of cancer (1). The most life-threatening aspect of the cancer is metastasis that spreads cancer cells from their original tissues to other ones (2). Cancerous tissues include collections of heterogeneous cell populations that are different in their apparent state of differentiation (3). These heterogeneous populations contain particular types of “tumor-initiating” cells arisen from mutations of normal stem cells (4). Stem cells are undifferentiated cells which were found in multi-cellular organisms. They have three main properties including differentiation, self-renewal, and homeostatic control (3). Accordingly, tumor-initiating cells were called “cancer stem cells” that have the capacity for self-renewal, being able to differentiate into any cell, and the proliferative ability to expand malignant cells (4). Although the exact cause of cancer is unknown, the understanding of the balance between self-renewal and differentiation is an essential way to prevent cancer formation and the therapeutic utilisation of stem cells in human diseases. Current cancer therapies including radiotherapy, surgery, and chemotherapy intend to destroy or kill every cell of the body (5). Cancer stem cells (CSCs) do not destroy easily with conventional methods of cancer treatment such as chemotherapy and radiation. Although, anticancer drugs can lessen tumor size, but do not kill cancer stem cells. Though CSCs might only create up a small part of a tumor, their resistance to drugs lets them persist. Therefore, the identification of tumor-initiating cancer stem cells (CSCs) is of significant interest in cancer research and suggests varied approaches (6). In the last few decades, nanotechnology and nanomaterials have found essential roles in cancer diagnosis and treatment for better early detections and also, more efficient drug delivery to the tumor cells. This review aims to highlight the opportunities and challenges for integrating the nanoscience with cancer biology and thereby, researches regarding the advanced nano-based detecting systems for cancer stem cells.
Cancer is defined as a biological condition with an uncontrolled division of the natural body cells. Until now, the complete treatment of cancer as a disease is difficult and costly. One reason for this difficulty relates to the presence of specific receptors on the surface of the cancer cells that pump the drugs out of the cells. Therefore, it is difficult to find a drug to eliminate these cells. However, all cells involved in tumor development are not equally dangerous. The leading cause of cancer is a group of dividing cells with high power and high resistance to the drugs that they called CSCs. CSCs are the cells in tumors or blood that are known particularly with the ability to turn into all cell types that are found in the host body. CSCs were identified and characterized in the 1990s, when a stem cell biologist, John Dick, said that his team had isolated scarce cells in the blood of people with leukemia that seemed to have a crucial role in cancer (7). Most researchers believe that CSC, in addition to leukemia, exists in solid tumors such as prostate, breast, and pancreas cancers. They can renew and keep themselves in the tumor masses (8–10). CSCs differentiate into several types of cells through the renewable process that can be the reason for the tumor formation. Such tumor cells as a distinct population remain and cause the disease to recur, metastasis and tumor development.
Some of the characteristics of the CSCs are similar to normal stem cells. These cells have extraordinary capability of self-renewal, differentiation and proliferative potential. The CSCs have been identified in tumor tissues and cell cultures by detecting the expressions of specific protein markers, such as CD133, CD44 and CD24 (11–13). Table 1 shows the markers of cancer stem cells in a variety of solid tumors (6, 10, 12, 14–31). The CSCs have the potential to be adapted to different environments. Therefore, the high flexibility of the CSCs can be used as a high potential for changing the plasticity and rigidity of their network (32). Environmental changes would often be strong enough for increasing the tumorigenicity of cancer stem cells (33). Generally, studies and trials have shown that the CSC self-renewal potential is determined by the high proliferative potential with a loss of regular differentiation program and high efficiency in response to environmental changes. The CSCs have two main phenotypes include the proliferative and quiescent state that characterized by symmetric and asymmetric cell divisions. The cancer stem cells with proliferative phenotypes have symmetric cell division and are less aggressive and could be classified as a plasticity network (34).
On the other hand, the CSCs with the quiescent phenotype have asymmetric cell division with dynamic properties and are classified as a rigid network (35). The CSCs with quiescent phenotype may be the main factor in the metastasis that would appear years after surgical treatment of a primary tumor. Protection of the CSCs against therapeutic agents and their resistance to the treatment process can be obtained by, quiescence, expression of ABC (ATP-binding cassette) drug pumps, increased expression of anti-apoptotic proteins and resistance to DNA damaging agents (Fig. 1).
It can be concluded that the tumor cells without the CSC marker would be killed by chemotherapy, resulting in tumor regression, but the cells with the CSC markers are resistant to the treatment and their number would be risen in a tumor (36–38). Surface markers of the CSC, in many types of cancers, are shown in Table 1.
The percentage of CSCs are different in many types of cancers (39, 40) and there are relations between the number of CSCs and patient prognosis (41, 42). Studies have demonstrated that cancer therapy could target the tumor cell mass and create a partial regression of a tumor, but the CSCs could make and develop new tumor clones, therefore, identification and directed targeting against this kind of stem cells have been reported to be more effective (Fig. 2) (40, 43). Cancer therapy, based on this hypothesis, was reported to decrease mechanisms of resistance to conventional chemotherapeutic drugs and radiation therapy. The new design of cancer therapeutic drugs could eliminate the CSCs by interfering with the specific pathways (44–48).
Scientists and researchers have suggested that comprehension of disorders in signal pathways or too much activation is one of the most vital importance for anticancer targeting of the CSCs. Wnt, Notch and Hedgehog signaling pathways have critical roles in the reappearance and maintenance of cancer stem cells (49). Wnt marks a group of signaling proteins that bind to surface receptor molecules on the target cells. Activation of Wnt target genes happens by β-Catenin. Also activated Wnt/β-Catenin signaling has been reported to be a key feature of epithelial cancers, epithelial-mesenchymal transition (EMT), and maintenance of the CSCs of melanoma, breast, colon, liver and lung cancers (50).
Notch signaling pathway has a critical role in the cell-fate decision, tissue patterning, morphogenesis (51), and maintenance of the glioblastoma and breast cancer stem cells (52). A reported study about Notch signaling pathway suggested that Notch inhibition in glioblastoma could decrease cancer stem cells via an endothelial cell intermediate (53).
Studies have suggested that the Hedgehog (Hh) signaling can regulate many cancer stem cells including glioblastoma, pancreatic adenocarcinoma, breast cancer, multiple myeloma, and chronic myeloid leukemia (CML) (20). Hh signaling has been found to play multiple roles in development, homeostasis and disease by activating the 7-pass transmembrane protein Smoothened (Smo), to send an intracellular signal. Due to that, the most advanced types of Hh antagonists are Smo-targeted (54, 55). Targeting the signal pathways including the Wnt, Notch, and Hh may improve current strategies for cancer therapy. Although, anti-cancer treatment can reduce tumor size, survived CSCs would trigger tumor formation again. This type of relapse causes metastasis and more resistant to treatment. Therefore, most currently available cancer treatments such as hormone therapy, radiotherapy and chemotherapy, would not be useful in the elimination of cancer stem cells (5). Specific targeting of tumor stem cells has been suggested to be an efficient alternative for cancer treatment (10, 11).
Today, cancer mortality is one of the most common causes of death. The application of nanotechnology in medicine includes six areas including 1) detection of molecular changes in diseases; 2) diagnosis and imaging; 3) drug delivery; 4) combined therapeutic and diagnostic applications; 5) report of therapeutic agent efficacy; and 6) nanotechnology applications in scientific discovery and basic research (56). A variety of nanostructures from organic and inorganic materials have been recently used for cancer therapy and diagnosis in passive or active tumor targeting. A novel formulation of nanovesicles, liposomes, polymeric micelles, dendrimers, and polymeric nanoparticles can enter into the solid tumor site through the porous structure of a tumor vascular system, and then, selectively deliver the therapeutic agents (57, 58). Nanomaterials with proper targeting ligands could bind to antigens or receptors on the target cells. However, this powerful technology leads to a decreased toxicity in non-targeted cells, and therefore, the distribution of nanomaterials will be increased in the target cells (59). Some of the nanomaterials shown in Table 2 (60–73) such as semiconducting nanoparticles or quantum dots (QDs), colloidal nanoparticles, dendrimers, magnetic nanoparticles (MNPs), carbon-based nanomaterials (CBNs), and liposomal nanoparticles systems have been constructed for utilization in therapeutic applications. Quantum dots (QDs) are semiconducting nanoparticles with the diameter ranging from 2~10 nm, narrow emission and broad absorption profile, high photostability, long fluorescence lifespan, ability to be conjugated with proteins (74). Also, QDs have the potential of applications in molecular and cellular labelling, cell tracking, drug delivery and also, cancer detection and therapy (75, 76). Dendrimers are highly branched synthetic polymers (about 1.5~13.5 nm) (77, 78) that have attracted considerable attention because of their hyper-branched structure, monodispersity, functional surface groups, and symmetrical conformation (79).
Furthermore, they have various types of core molecules, such as ethylenediamine, cysteamine, diaminobutane, carbohydrate, calixarene, etc. and functional surface groups like amino, carboxyl, hydroxyl that give the opportunity to modify their surfaces with biomolecules (80). Polyamidoamines (PAMAMs) are the most widely studied dendrimer because of the polycationic properties that can have interaction with charged molecules (81). Gold nanoparticles (GNPs), with diameter size of 0.8 to 200 nm have been used for imaging, targeting, drug delivery, and therapy applications. Biological molecules can be readily attached to the surfaces of the GNPs. Binding of molecules including biotin, peptides or oligonucleotides enhances cell penetration efficiency of the nanoparticles. Surface plasmon resonance of GNPs is used to many applications such as hyperthermia (82). Superparamagnetic NPs (SPIONs) are small synthetic particles of iron oxides with a core size of 0~10 nm. They show new magnetization behaviour totally like standard paramagnetic materials, but with, high field irreversibility, high saturation field, additional anisotropy contributions or shifted loops after field cooling (83, 84). Also, after eliminating the magnetic field, they do not show magnetic interactions (85). The SPIONs well-dispersed in a liquid and especially in water have drastically increased their biological and medical applications in recent years. Applications of SPIONs are diagnosis, therapy, and drug delivery system; Moreover, recent systems are used on stem cell differentiating/tracking (85, 86). Carbon-based nanomaterials (CBNs) are one of the most widely used tools in the fields of biomedicine, biotechnology, environment, and electronics (87, 88). CBNs with excellent physical, mechanical, electronic, and biological properties are engineered by using graphite, in different types of graphene, fullerene, and single- or multi-walled CNTs (89). Besides, modification of CBNs with biomaterials have been utilized in imaging, delivery systems, targeted therapy, tissue scaffold reinforcements, and bio-detection systems (90). Liposomes, as the most clinically established nano-scaled platforms, are merely formed from phospholipids and cholesterol by a self-assembling method in aqueous media in a variable size distribution from 20~1000 nm (91). The most application of liposomes is in drug delivery systems in which encapsulated drugs could be protected and directly delivered to the cells within lipid bilayers (92). Fig. 3 demonstrates the various nanomaterial that targeted cancer stem cells.
The CSCs are believed to drive tumor growth and be highly resistant to conventional therapies (e.g. chemotherapy and radiotherapy) and lead to disease relapse and the formation of metastases. Therefore, there is considerable interest in the use of nano-sized materials for CSC-directed anti-cancer therapies. The surface of nanoparticles has been designed to accurately and effectively target the CSCs (93). Identification of the CSC is possible by some markers, e.g. ALDH, CD44, CD90, and CD133, and specific signaling pathways, i.e. Notch, Hedgehog and transforming growth factor-β (TGF-β) (94, 95) to improve the treatment strategies and therapeutic outcomes (96).
Aldehyde Dehydrogenases (ALDH) is a functional marker of the CSCs and also, the scavenger of reactive oxygen species (ROS) (97–99). Studies have been demonstrated that ALDH activity of converting retinol to retinoic acid increases in many cancers (100, 101), such as cervical cancer (102), non-small cell lung cancer (103), melanoma (104), and also is considered as a specific marker of BCSCs in breast cancer (105). Disulfiram (DS), is a specific inhibitor of the ALDH that have cytotoxic effects on a wide range of cancer cell lines including colon, breast and brain ones (106–112). However, its clinical application is limited due to very short half-life in the bloodstream. A study revealed that encapsulation of the by poly (lactic-co-glycolic) acid (PLGA) nanoparticles (DS-PLGA) causes longer circulating time in the bloodstream, and also, DS-PLGA/Cu combination manifests very likely synergistic cytotoxicity effect with 5-FU and sorafenib. Finally, the results suggest that nano-based controlled delivery system would make DS fast translation into liver cancer cells (97).
CD44 factor is one of the most popular and familiar surface biomarkers associated with cancer stem cells (113). Clinical studies have demonstrated the CD44 as one of the most critical proteins in tumor genesis, growth, metastasis, and chemo-resistant processes in a verity of tumors in colon, breast, head and neck, and pancreatic cancers cancers (26, 114, 115). Antibody-based cancer treatments, over-expressing CD44, represents the dominant anti-CSC approach specifically in BCSCs (116). In a study, active targeting and drug delivery to the breast and colon cancer cell lines was evaluated by biodegradable poly[(D, L-lactide co-glycolide)-co-PEG] (PDLGA-co-PEG) polymeric micelles loaded with paclitaxel (PTX) and functionalized with anti-CD44 antibodies. Evidence has shown that active-targeted PLGA-co-PEG-PTX micelles increase the intracellular concentration of PTX, and therefore, the effectiveness of drug delivery to the CSC (117). Arabi et al. (118) have demonstrated the selective drug delivery potential of liposomal doxorubicin functionalized with anti-CD44 and increased circulation time, biodistribution and therapeutic efficacies of CD44-Doxil compared to the non-targeted liposomal formulation and free drug. Magnetic NPs (MNPs) with anti-CD44 antibody have also shown the potential selective treatment of CD44 positive cancers such as pancreatic and breast cancers cell lines (119).
As mentioned before, the CSCs can be identified by detection of the CD133 expression as a stem cell marker and also, is explicitly regarded as a marker in osteosarcoma. The potential of CD133 targeting for drug delivery to the CSCs in osteosarcoma has been examined previously. It is illustrated in a study that Poly (lactic-co-glycolic acid) nanoparticles conjugated with CD133 aptamers would deliver salinomycin specifically and efficiently to the CD133+ CSCs in osteosarcoma and kill them (120). In another new form of drug nanocarrier, mesoporous silica nanoparticles (MSNs) have been used to deliver both antitumor drugs and siRNA into CD133+ cancer cells. This formulation significantly improved the efficacy of therapeutic drugs in laryngeal cancer cells (121). Curcumin and some other natural chemopreventive agents have recently been shown to inhibit the CSCs, but with low bioavailability.
Furthermore, various nanotechnology-based formulations of curcumin have been recently evaluated as well. Preparation of the nanoscale drug delivery systems has been considered as an innovative approach to overcome the bioavailability and stability issues. For instance, it has been shown that a polymeric nanoparticle (made by mixing N-isopropylacrylamide, vinylpyrrolidone, and acrylic acid in a molar ratio of 60:20:20) encapsulating curcumin, named “nanocurcumin”, can inhibit brain tumor cell growth and deplete CD133+stem-like cells (122, 123).
Another major pathway involved in the CSC regulation isNotch signaling pathway that controls cell-fate determination during development and maintains adult tissue homeostasis (124). This pathway could lead to the development of many cancers including melanoma, medulloblastoma, rhabdomyosarcoma, basal cell carcinoma, breast, lung, liver, pancreas, and prostate cancers (125). Fan et al. (126) examined the effects of Notch pathway inhibition on the growth and xenograft formation of medulloblastoma. They found that Notch blockade results in the growth of tumor cells and tumor-forming capacity, due to the depletion of stem-like cells. Clinical application of Notch inhibitors, γ-secretase inhibitors (GSIs), is restricted by severe side effects. Imagable mesoporous silica nanoparticles (MSNPs) have been developed as vehicles for targeted delivery of GSIs to block Notch signaling. GSI-loaded on biocompatible and biodegradable MSNPs enhanced therapeutic efficacy in tumor reduction and regulation of Notch driven stem cell fates (127). Specific functionalization of nanoparticles could be used to enhance targetability to specific cell populations. Mamaeva et al. (128) have demonstrated that breast CSCs display enhanced glucose uptake compared to normal breast cancer cells. This specific feature of the breast CSCs has been utilized to design functionalized mesoporous silica nanoparticles (MSNs). The MSNs were functionalized with glucose analogues to deliver Notch signaling inhibitors to the CSCs efficiently. The results have demonstrated a reduction in the CSC pool and suppression of tumor growth in vitro and in vivo.
CD90 is glycosylphosphatidylinositol (GPI) anchored glycoprotein that is expressed in leukocytes, bone marrow-derived mesenchymal stem cells, hepatic stem/progenitor cells (HSPCs) and also, is identified in murine breast CSCs. Studies have shown that CD90+ cells have tumorigenicity and metastatic potential (129). 90% of blood samples from liver cancer patients contained CD45−/CD90+ markers (25). Bakalova et al. (130) have utilized CD90 as a valid therapeutic target They have shown that photosensitizer trifluoperazine loaded in anti-CD90 antibody-mediated water-soluble CdSe core nanocrystals could be delivered directly to the CD90+ leukemia CSCs where it could cause leukemia CSCs sensitivity to UV irradiation and apoptotic cell death.
It is known that the Hh pathway helps in controlling cell growth and morphogenesis. The components of the Hh signal transduction pathway can control embryonic development and also, is expressed in postnatal and adult tissues. These components have assigned roles in the maintenance of stem cells and tissue repair (131). At least three Hh gene homologs have been identified in human: Sonic Hh (SHh), Desert Hh (Dhh), and Indian Hh (Ihh), among which SHh is the most widely used one (132). Hh ligand can bind to the 12-transmembrane receptor Patched 1, which relieves seven-pass transmembrane receptor named Smoothened (Smo) from repression and allows downstream activation of the pathway through the translocation of GLI, where it acts as a transcription factor to the nucleus with the mediation of SUFU and KIF17. High expression and aberrant activation of Hh ligands present in the majority of human cancers including brain tumors, melanomas, leukemia’s, gastrointestinal, malignancies of the breast, ovary, prostate and, pancreas cancers (133). Therefore, targeting the Hh signaling pathway may provide a practical therapeutic approach in the treatment of various cancers. GLI inhibition through PLGA-PEG nanoparticles (NanoHHI) has illustrated tumor growth inhibition and antimetastatic effects in hepatocellular carcinoma (HCC) models (134, 135). In another study, the effects of anthothecol-encapsulated PLGA-nanoparticles (Antho-NPs) have been examined on the behaviour of pancreatic cancer stem cells (CSCs). They have discovered that Antho-NPs inhibited the CSC proliferation and induced apoptosis by disrupting GLI-DNA binding and also, it acts as GLIinhibitor. Using PLGA nanoparticles offers many benefits of appropriate stability in physiological environments, entering to a cancerous sphere without any auxiliary reagents, loss of toxicity towards human pancreatic normal cells and PLGA-NPs could cross the blood-tumor barrier (136).
TGF-β signaling is an important prognostic marker in various types of cancers including breast (137), colon (138), liver (139), lung (140) and ovary tumors (141). Also, its potential role in the CSCs has been recently reported in breast (142), colon (143), liver (144), and lung (145), cancers. Thus, selective targeting of TGF-β signaling would significantly improve therapeutic efficiency in tumor models (146). For breast cancer treatment, polyethyleneimine/polyethylene glycol-conjugated MSNPs were developed to load LY364947 as a TGF-β inhibitor (146). These polymeric nanoparticles and inhibition of TNF-β signaling pathway have been used in the delivery of siRNA to the breast CSCs. Nanoparticles could be accumulated in tumors that in turn, increases siRNA concentrations in tumor tissues and notably, lower the proportions of the CSCs (147). Gold NPs (AuNPs) with much application in therapeutic and diagnostic agents could selectively deactivate the TGF-β signaling pathway. Tsai et al. have found that AuNPs could attenuate the immunosuppressive function of TGF-β1β signaling pathway and also, increase the number and frequency of tumor-infiltrating T lymphocytes (148).
The cancer treatment needs the improvement of approaches which can efficiently eliminate cancer and amend the application of this novel drug-delivery modality (nanomedicines) (149, 150). Recently, researchers have been interested in the nanotechnology-based drug because these drugs are most useful for developing anti-cancer therapies and CSCs targeting. There is some clinically approved nanomedicine include Pegylated liposome (Doxil), Albumin-bound paclitaxel particles (Abraxane), Iron oxide nanoparticles (NanoTherm), PEG-1 Asparaginase (Oncaspar), Methoxy-PEG-poly (d,l-lactide)-paclitaxel micelle (Gene-xol-PM) and SMANCS (Zinostatin) (151). Considering these issues that mentioned the nanotechnology-based anticancer drug helps treat and prevent various types of cancers because these drugs have excellent diffusion capacity, selective killing of tumor cells or CSCs. Also, CSC-targeting with nanomaterials is in the early stages because these studies have done in vitro and in vitro phase only. So a vast amount of detail in vitro, in vivo and other relevant information, is essential that these nanomedicine products have a clinical practice. The other important issues are the safety, effective dosages and side effects of nanomaterials used in drug delivery systems which should be given special attention (152).
Finally, the most important thing is that the treatment of cancer using nanotechnology is helpful approaches for cancer patients because most of them suffer from chemotherapy and radiotherapy. Moreover, CSCs targeting with nanomaterial may present a novel method for reducing the costs on the public health care system.
The basic cause of cancer is a group of dividing cells with high power and high resistance to the drugs that they called Cancer Stem Cells (CSCs). CSCs were identified an characterized in the 1990s, in the blood of people with leukemia that seemed to have a vital role in cancer. CSCs are markedly resistant to conventional cancer treatments, such as chemotherapy and radiation. Therefore, understanding tumor biology is critical for the development of successful therapies. Identifying and selectively targeting markers and signaling pathways of CSCs are new therapeutic strategies for cancer treatment. The current success in the fight against CSCs has been reported in studies that using nanotechnology-based therapeutics with using different surface markers and biochemical assays for identification. Based on nanomedicine studies mentioned in our review, it is shown that nanomedicine in the treatment of CSCs can provide additional benefits for cancer patients with fewer drugs side effects such as, increasing their cellular uptake, prolonging systemic circulation, improving biodistribution profiles, and resolving problems of poor stability and solubility. This review has shown bright prospects of cancer treatment by nanomaterials, and specifically nanoparticles with increased therapeutic potency in drug delivery, CSC specificity and fewer side effects.
Acknowledgments
The authors thank the Department of Medical Nanotechnology, School of Advanced Technologies in Medicine, Tehran University of Medical Sciences for all the support provided.
References
1. Siegel R, Naishadham D, Jemal A. Cancer statistics, 2013. CA Cancer J Clin. 2013; 63:11–30. DOI: 10.3322/caac.21166. PMID: 23335087.


2. Sahai E. Mechanisms of cancer cell invasion. Curr Opin Genet Dev. 2005; 15:87–96. DOI: 10.1016/j.gde.2004.12.002. PMID: 15661538.


3. Dalerba P, Cho RW, Clarke MF. Cancer stem cells: models and concepts. Annu Rev Med. 2007; 58:267–284. DOI: 10.1146/annurev.med.58.062105.204854. PMID: 17002552.


4. Jordan CT, Guzman ML, Noble M. Cancer stem cells. N Engl J Med. 2006; 355:1253–1261. DOI: 10.1056/NEJMra061808. PMID: 16990388.


5. World Health Organization. WHO handbook for reporting results of cancer treatment. Geneva: WHO;1979.
6. Li C, Heidt DG, Dalerba P, Burant CF, Zhang L, Adsay V, Wicha M, Clarke MF, Simeone DM. Identification of pancreatic cancer stem cells. Cancer Res. 2007; 67:1030–1037. DOI: 10.1158/0008-5472.CAN-06-2030. PMID: 17283135.


7. Bonnet D, Dick JE. Human acute myeloid leukemia is organized as a hierarchy that originates from a primitive hematopoietic cell. Nat Med. 1997; 3:730–737. DOI: 10.1038/nm0797-730. PMID: 9212098.


8. Ciurea ME, Georgescu AM, Purcaru SO, Artene SA, Emami GH, Boldeanu MV, Tache DE, Dricu A. Cancer stem cells: biological functions and therapeutically targeting. Int J Mol Sci. 2014; 15:8169–8185. DOI: 10.3390/ijms15058169. PMID: 24821540. PMCID: PMC4057726.
9. Castelo-Branco P, Zhang C, Lipman T, Fujitani M, Hansford L, Clarke I, Harley CB, Tressler R, Malkin D, Walker E, Kaplan DR, Dirks P, Tabori U. Neural tumor-initiating cells have distinct telomere maintenance and can be safely targeted for telomerase inhibition. Clin Cancer Res. 2011; 17:111–121. DOI: 10.1158/1078-0432.CCR-10-2075. PMID: 21208905.


10. Li C, Hynes MJ, Jing J. Pancreatic cancer stem cells: new direction for pancreatic cancer treatment. Trends Bio/Pharm Ind. 2010; 6:34–40.
11. Miki J, Furusato B, Li H, Gu Y, Takahashi H, Egawa S, Sesterhenn IA, McLeod DG, Srivastava S, Rhim JS. Identification of putative stem cell markers, CD133 and CXCR4, in hTERT-immortalized primary nonmalignant and malignant tumor-derived human prostate epithelial cell lines and in prostate cancer specimens. Cancer Res. 2007; 67:3153–3161. DOI: 10.1158/0008-5472.CAN-06-4429. PMID: 17409422.


12. Ricardo S, Vieira AF, Gerhard R, Leitão D, Pinto R, Cameselle-Teijeiro JF, Milanezi F, Schmitt F, Paredes J. Breast cancer stem cell markers CD44, CD24 and ALDH1: expression distribution within intrinsic molecular subtype. J Clin Pathol. 2011; 64:937–946. DOI: 10.1136/jcp.2011.090456. PMID: 21680574.


13. Takaishi S, Okumura T, Tu S, Wang SS, Shibata W, Vigneshwaran R, Gordon SA, Shimada Y, Wang TC. Identification of gastric cancer stem cells using the cell surface marker CD44. Stem Cells. 2009; 27:1006–1020. DOI: 10.1002/stem.30. PMID: 19415765. PMCID: PMC2746367.


14. Burnett J. The natural product sulforaphane inhibits breast cancer stem cell targets in triple negative and trastuzumab-resistant breast cancers [PhD dissertation]. Michigan: University of Michigan;2015.
15. Wang JC, Dick JE. Cancer stem cells: lessons from leukemia. Trends Cell Biol. 2005; 15:494–501. DOI: 10.1016/j.tcb.2005.07.004. PMID: 16084092.


16. Hosen N, Park CY, Tatsumi N, Oji Y, Sugiyama H, Gramatzki M, Krensky AM, Weissman IL. CD96 is a leukemic stem cell-specific marker in human acute myeloid leukemia. Proc Natl Acad Sci U S A. 2007; 104:11008–11013. DOI: 10.1073/pnas.0704271104. PMID: 17576927. PMCID: PMC1904175.


17. Croker AK, Goodale D, Chu J, Postenka C, Hedley BD, Hess DA, Allan AL. High aldehyde dehydrogenase and expression of cancer stem cell markers selects for breast cancer cells with enhanced malignant and metastatic ability. J Cell Mol Med. 2009; 13:2236–2252. DOI: 10.1111/j.1582-4934.2008.00455.x. PMID: 18681906. PMCID: PMC6512388.


18. Beier D, Hau P, Proescholdt M, Lohmeier A, Wischhusen J, Oefner PJ, Aigner L, Brawanski A, Bogdahn U, Beier CP. CD133(+) and CD133(−) glioblastoma-derived cancer stem cells show differential growth characteristics and molecular profiles. Cancer Res. 2007; 67:4010–4015. DOI: 10.1158/0008-5472.CAN-06-4180. PMID: 17483311.


19. Singh SK, Clarke ID, Terasaki M, Bonn VE, Hawkins C, Squire J, Dirks PB. Identification of a cancer stem cell in human brain tumors. Cancer Res. 2003; 63:5821–5828. PMID: 14522905.
20. Peacock CD, Wang Q, Gesell GS, Corcoran-Schwartz IM, Jones E, Kim J, Devereux WL, Rhodes JT, Huff CA, Beachy PA, Watkins DN, Matsui W. Hedgehog signaling maintains a tumor stem cell compartment in multiple myeloma. Proc Natl Acad Sci U S A. 2007; 104:4048–4053. DOI: 10.1073/pnas.0611682104. PMID: 17360475. PMCID: PMC1805487.


21. Dammacco F, Leone P, Silvestris F, Racanelli V, Vacca A. Cancer stem cells in multiple myeloma and the development of novel therapeutic strategies. Dammacco F, editor. Oncogenomics. London: Elsevier;2019. p. 121–137. DOI: 10.1016/B978-0-12-811785-9.00009-0.


22. Hermann PC, Huber SL, Herrler T, Aicher A, Ellwart JW, Guba M, Bruns CJ, Heeschen C. Distinct populations of cancer stem cells determine tumor growth and metastatic activity in human pancreatic cancer. Cell Stem Cell. 2007; 1:313–323. DOI: 10.1016/j.stem.2007.06.002. PMID: 18371365.


23. Vermeulen L, De Sousa E, Melo F, van der Heijden M, Cameron K, de Jong JH, Borovski T, Tuynman JB, Todaro M, Merz C, Rodermond H, Sprick MR, Kemper K, Richel DJ, Stassi G, Medema JP. Wnt activity defines colon cancer stem cells and is regulated by the microenvironment. Nat Cell Biol. 2010; 12:468–476. DOI: 10.1038/ncb2048. PMID: 20418870.


24. Ma S, Chan KW, Hu L, Lee TK, Wo JY, Ng IO, Zheng BJ, Guan XY. Identification and characterization of tumorigenic liver cancer stem/progenitor cells. Gastroenterology. 2007; 132:2542–2556. DOI: 10.1053/j.gastro.2007.04.025. PMID: 17570225.


25. Yang ZF, Ho DW, Ng MN, Lau CK, Yu WC, Ngai P, Chu PW, Lam CT, Poon RT, Fan ST. Significance of CD90+ cancer stem cells in human liver cancer. Cancer Cell. 2008; 13:153–166. DOI: 10.1016/j.ccr.2008.01.013. PMID: 18242515.


26. Collins AT, Berry PA, Hyde C, Stower MJ, Maitland NJ. Prospective identification of tumorigenic prostate cancer stem cells. Cancer Res. 2005; 65:10946–10951. DOI: 10.1158/0008-5472.CAN-05-2018. PMID: 16322242.


27. Liu C, Kelnar K, Liu B, Chen X, Calhoun-Davis T, Li H, Patrawala L, Yan H, Jeter C, Honorio S, Wiggins JF, Bader AG, Fagin R, Brown D, Tang DG. The microRNA miR-34a inhibits prostate cancer stem cells and metastasis by directly repressing CD44. Nat Med. 2011; 17:211–215. DOI: 10.1038/nm.2284. PMID: 21240262. PMCID: PMC3076220.


28. Eramo A, Lotti F, Sette G, Pilozzi E, Biffoni M, Di Virgilio A, Conticello C, Ruco L, Peschle C, De Maria R. Identification and expansion of the tumorigenic lung cancer stem cell population. Cell Death Differ. 2008; 15:504–514. DOI: 10.1038/sj.cdd.4402283. PMID: 18049477.


29. Gao MQ, Choi YP, Kang S, Youn JH, Cho NH. CD24+ cells from hierarchically organized ovarian cancer are enriched in cancer stem cells. Oncogene. 2010; 29:2672–2680. DOI: 10.1038/onc.2010.35. PMID: 20190812.


30. Silva IA, Bai S, McLean K, Yang K, Griffith K, Thomas D, Ginestier C, Johnston C, Kueck A, Reynolds RK, Wicha MS, Buckanovich RJ. Aldehyde dehydrogenase in combination with CD133 defines angiogenic ovarian cancer stem cells that portend poor patient survival. Cancer Res. 2011; 71:3991–4001. DOI: 10.1158/0008-5472.CAN-10-3175. PMID: 21498635. PMCID: PMC3107359.


31. Zhang S, Balch C, Chan MW, Lai HC, Matei D, Schilder JM, Yan PS, Huang TH, Nephew KP. Identification and characterization of ovarian cancer-initiating cells from primary human tumors. Cancer Res. 2008; 68:4311–4320. DOI: 10.1158/0008-5472.CAN-08-0364. PMID: 18519691. PMCID: PMC2553722.


32. Csermely P, Hódsági J, Korcsmáros T, Módos D, Perez-Lopez ÁR, Szalay K, Veres DV, Lenti K, Wu LY, Zhang XS. Cancer stem cells display extremely large evolvability: alternating plastic and rigid networks as a potential mechanism: network models, novel therapeutic target strategies, and the contributions of hypoxia, inflammation and cellular senescence. Semin Cancer Biol. 2015; 30:42–51. DOI: 10.1016/j.semcancer.2013.12.004. PMID: 24412105.


33. Liu L, Rando TA. Manifestations and mechanisms of stem cell aging. J Cell Biol. 2011; 193:257–266. DOI: 10.1083/jcb.201010131. PMID: 21502357. PMCID: PMC3080271.


34. Hoek KS, Goding CR. Cancer stem cells versus phenotype-switching in melanoma. Pigment Cell Melanoma Res. 2010; 23:746–759. DOI: 10.1111/j.1755-148X.2010.00757.x. PMID: 20726948.


35. Visvader JE, Lindeman GJ. Cancer stem cells in solid tumours: accumulating evidence and unresolved questions. Nat Rev Cancer. 2008; 8:755–768. DOI: 10.1038/nrc2499. PMID: 18784658.


36. Singh A, Settleman J. EMT, cancer stem cells and drug resistance: an emerging axis of evil in the war on cancer. Oncogene. 2010; 29:4741–4751. DOI: 10.1038/onc.2010.215. PMID: 20531305. PMCID: PMC3176718.


37. Fletcher JI, Haber M, Henderson MJ, Norris MD. ABC transporters in cancer: more than just drug efflux pumps. Nat Rev Cancer. 2010; 10:147–156. DOI: 10.1038/nrc2789. PMID: 20075923.


38. Gottesman MM. Mechanisms of cancer drug resistance. Annu Rev Med. 2002; 53:615–627. DOI: 10.1146/annurev.med.53.082901.103929. PMID: 11818492.


39. Quintana E, Shackleton M, Sabel MS, Fullen DR, Johnson TM, Morrison SJ. Efficient tumour formation by single human melanoma cells. Nature. 2008; 456:593–598. DOI: 10.1038/nature07567. PMID: 19052619. PMCID: PMC2597380.


40. Cho RW, Clarke MF. Recent advances in cancer stem cells. Curr Opin Genet Dev. 2008; 18:48–53. DOI: 10.1016/j.gde.2008.01.017. PMID: 18356041.


41. Bloushtain-Qimron N, Yao J, Snyder EL, Shipitsin M, Campbell LL, Mani SA, Hu M, Chen H, Ustyansky V, Antosiewicz JE, Argani P, Halushka MK, Thomson JA, Pharoah P, Porgador A, Sukumar S, Parsons R, Richardson AL, Stampfer MR, Gelman RS, Nikolskaya T, Nikolsky Y, Polyak K. Cell type-specific DNA methylation patterns in the human breast. Proc Natl Acad Sci U S A. 2008; 105:14076–14081. DOI: 10.1073/pnas.0805206105. PMID: 18780791. PMCID: PMC2532972.


42. Shipitsin M, Campbell LL, Argani P, Weremowicz S, Bloushtain-Qimron N, Yao J, Nikolskaya T, Serebryiskaya T, Beroukhim R, Hu M, Halushka MK, Sukumar S, Parker LM, Anderson KS, Harris LN, Garber JE, Richardson AL, Schnitt SJ, Nikolsky Y, Gelman RS, Polyak K. Molecular definition of breast tumor heterogeneity. Cancer Cell. 2007; 11:259–273. DOI: 10.1016/j.ccr.2007.01.013. PMID: 17349583.


43. Dick JE. Looking ahead in cancer stem cell research. Nat Biotechnol. 2009; 27:44–46. DOI: 10.1038/nbt0109-44. PMID: 19131997.


44. Moitra K, Lou H, Dean M. Multidrug efflux pumps and cancer stem cells: insights into multidrug resistance and therapeutic development. Clin Pharmacol Ther. 2011; 89:491–502. DOI: 10.1038/clpt.2011.14. PMID: 21368752.


45. Vermeulen L, de Sousa e Melo F, Richel DJ, Medema JP. The developing cancer stem-cell model: clinical challenges and opportunities. Lancet Oncol. 2012; 13:e83–e89. DOI: 10.1016/S1470-2045(11)70257-1. PMID: 22300863.


46. Zhou BB, Zhang H, Damelin M, Geles KG, Grindley JC, Dirks PB. Tumour-initiating cells: challenges and opportunities for anticancer drug discovery. Nat Rev Drug Discov. 2009; 8:806–823. DOI: 10.1038/nrd2137. PMID: 19794444.


47. Naujokat C, Steinhart R. Salinomycin as a drug for targeting human cancer stem cells. J Biomed Biotechnol. 2012; 2012:950658. DOI: 10.1155/2012/950658. PMID: 23251084. PMCID: PMC3516046.


48. Domingo-Domenech J, Vidal SJ, Rodriguez-Bravo V, Castillo-Martin M, Quinn SA, Rodriguez-Barrueco R, Bonal DM, Charytonowicz E, Gladoun N, de la Iglesia-Vicente J, Petrylak DP, Benson MC, Silva JM, Cordon-Cardo C. Suppression of acquired docetaxel resistance in prostate cancer through depletion of notch- and hedgehog-dependent tumor-initiating cells. Cancer Cell. 2012; 22:373–388. DOI: 10.1016/j.ccr.2012.07.016. PMID: 22975379. PMCID: PMC5989708.


49. Hu Y, Fu L. Targeting cancer stem cells: a new therapy to cure cancer patients. Am J Cancer Res. 2012; 2:340–356. PMID: 22679565. PMCID: PMC3365812.
50. Zhao C, Blum J, Chen A, Kwon HY, Jung SH, Cook JM, Lagoo A, Reya T. Loss of beta-catenin impairs the renewal of normal and CML stem cells in vivo. Cancer Cell. 2007; 12:528–541. DOI: 10.1016/j.ccr.2007.11.003. PMID: 18068630. PMCID: PMC2262869.


51. Yin L, Velazquez OC, Liu ZJ. Notch signaling: emerging molecular targets for cancer therapy. Biochem Pharmacol. 2010; 80:690–701. DOI: 10.1016/j.bcp.2010.03.026. PMID: 20361945.


52. Guo S, Liu M, Gonzalez-Perez RR. Role of Notch and its oncogenic signaling crosstalk in breast cancer. Biochim Biophys Acta. 2011; 1815:197–213. PMID: 21193018. PMCID: PMC3060666.


53. Hovinga KE, Shimizu F, Wang R, Panagiotakos G, Van Der Heijden M, Moayedpardazi H, Correia AS, Soulet D, Major T, Menon J, Tabar V. Inhibition of notch signaling in glioblastoma targets cancer stem cells via an endothelial cell intermediate. Stem Cells. 2010; 28:1019–1029. DOI: 10.1002/stem.429. PMID: 20506127. PMCID: PMC5532884.


54. Peukert S, Miller-Moslin K. Small-molecule inhibitors of the hedgehog signaling pathway as cancer therapeutics. ChemMedChem. 2010; 5:500–512. DOI: 10.1002/cmdc.201000011. PMID: 20229564.


55. Chen JK, Taipale J, Cooper MK, Beachy PA. Inhibition of Hedgehog signaling by direct binding of cyclopamine to Smoothened. Genes Dev. 2002; 16:2743–2748. DOI: 10.1101/gad.1025302. PMID: 12414725. PMCID: PMC187469.


56. Alexis F, Rhee JW, Richie JP, Radovic-Moreno AF, Langer R, Farokhzad OC. New frontiers in nanotechnology for cancer treatment. Urol Oncol. 2008; 26:74–85. DOI: 10.1016/j.urolonc.2007.03.017. PMID: 18190835.


57. Guler B, Demir B, Guler E, Gulec K, Yesiltepe O, Demirkol DO, Timur S. Targeting and imaging of cancer cells using nanomaterials. Grumezescu AM, editor. Nanobiomaterials in Medical Imaging. Oxford: Elsevier;2016. p. 209–251. DOI: 10.1016/B978-0-323-41736-5.00007-8.


58. Bae KH, Chung HJ, Park TG. Nanomaterials for cancer therapy and imaging. Mol Cells. 2011; 31:295–302. DOI: 10.1007/s10059-011-0051-5. PMID: 21360197. PMCID: PMC3933969.


59. Liu Q, Jin C, Wang Y, Fang X, Zhang X, Chen Z, Tan W. Aptamer-conjugated nanomaterials for specific cancer cell recognition and targeted cancer therapy. NPG Asia Mater. 2014; 6:pii: e95. DOI: 10.1038/am.2014.12. PMID: 29619132. PMCID: PMC5880215.


60. Tan WB, Jiang S, Zhang Y. Quantum-dot based nanoparticles for targeted silencing of HER2/neu gene via RNA interference. Biomaterials. 2007; 28:1565–1571. DOI: 10.1016/j.biomaterials.2006.11.018. PMID: 17161865.


61. Savla R, Taratula O, Garbuzenko O, Minko T. Tumor targeted quantum dot-mucin 1 aptamer-doxorubicin conjugate for imaging and treatment of cancer. J Control Release. 2011; 153:16–22. DOI: 10.1016/j.jconrel.2011.02.015. PMID: 21342659.


62. Ruan J, Song H, Qian Q, Li C, Wang K, Bao C, Cui D. HER2 monoclonal antibody conjugated RNase-A-associated CdTe quantum dots for targeted imaging and therapy of gastric cancer. Biomaterials. 2012; 33:7093–7102. DOI: 10.1016/j.biomaterials.2012.06.053. PMID: 22796163.


63. Li Y, He H, Jia X, Lu WL, Lou J, Wei Y. A dual-targeting nanocarrier based on poly(amidoamine) dendrimers conjugated with transferrin and tamoxifen for treating brain gliomas. Biomaterials. 2012; 33:3899–3908. DOI: 10.1016/j.biomaterials.2012.02.004. PMID: 22364698.


64. Yang W, Cheng Y, Xu T, Wang X, Wen LP. Targeting cancer cells with biotin-dendrimer conjugates. Eur J Med Chem. 2009; 44:862–868. DOI: 10.1016/j.ejmech.2008.04.021. PMID: 18550227.


65. Montet X, Funovics M, Montet-Abou K, Weissleder R, Josephson L. Multivalent effects of RGD peptides obtained by nanoparticle display. J Med Chem. 2006; 49:6087–6093. DOI: 10.1021/jm060515m. PMID: 17004722.


66. Toma A, Otsuji E, Kuriu Y, Okamoto K, Ichikawa D, Hagiwara A, Ito H, Nishimura T, Yamagishi H. Monoclonal antibody A7-superparamagnetic iron oxide as contrast agent of MR imaging of rectal carcinoma. Br J Cancer. 2005; 93:131–136. DOI: 10.1038/sj.bjc.6602668. PMID: 15970924. PMCID: PMC2361484.


67. Chanda N, Kattumuri V, Shukla R, Zambre A, Katti K, Upendran A, Kulkarni RR, Kan P, Fent GM, Casteel SW, Smith CJ, Boote E, Robertson JD, Cutler C, Lever JR, Katti KV, Kannan R. Bombesin functionalized gold nanoparticles show in vitro and in vivo cancer receptor specificity. Proc Natl Acad Sci U S A. 2010; 107:8760–8765. DOI: 10.1073/pnas.1002143107. PMID: 20410458. PMCID: PMC2889350.


68. Patra CR, Bhattacharya R, Mukherjee P. Fabrication and functional characterization of goldnanoconjugates for potential application in ovarian cancer. J Mater Chem. 2010; 20:547–554. DOI: 10.1039/B913224D. PMID: 20436942. PMCID: PMC2860188.


69. Li JL, Wang L, Liu XY, Zhang ZP, Guo HC, Liu WM, Tang SH. In vitro cancer cell imaging and therapy using transferrin-conjugated gold nanoparticles. Cancer Lett. 2009; 274:319–326. DOI: 10.1016/j.canlet.2008.09.024. PMID: 18977071.


70. Li R, Wu R, Zhao L, Hu Z, Guo S, Pan X, Zou H. Folate and iron difunctionalized multiwall carbon nanotubes as dual-targeted drug nanocarrier to cancer cells. Carbon. 2011; 49:1797–1805. DOI: 10.1016/j.carbon.2011.01.003.


71. Yao HJ, Zhang YG, Sun L, Liu Y. The effect of hyaluronic acid functionalized carbon nanotubes loaded with salinomycin on gastric cancer stem cells. Biomaterials. 2014; 35:9208–9223. DOI: 10.1016/j.biomaterials.2014.07.033. PMID: 25115788.


72. Sun X, Liu Z, Welsher K, Robinson JT, Goodwin A, Zaric S, Dai H. Nano-graphene oxide for cellular imaging and drug delivery. Nano Res. 2008; 1:203–212. DOI: 10.1007/s12274-008-8021-8. PMID: 20216934. PMCID: PMC2834318.


73. Laginha KM, Moase EH, Yu N, Huang A, Allen TM. Bioavailability and therapeutic efficacy of HER2 scFv-targeted liposomal doxorubicin in a murine model of HER2-overexpressing breast cancer. J Drug Target. 2008; 16:605–610. DOI: 10.1080/10611860802229978. PMID: 18686132.


74. Chen Y, Liang H. Applications of quantum dots with up-converting luminescence in bioimaging. J Photochem Photobiol B. 2014; 135:23–32. DOI: 10.1016/j.jphotobiol.2014.04.003. PMID: 24792570.


75. Zhao Y, Liu S, Li Y, Jiang W, Chang Y, Pan S, Fang X, Wang YA, Wang J. Synthesis and grafting of folate-PEG-PAMAM conjugates onto quantum dots for selective targeting of folate-receptor-positive tumor cells. J Colloid Interface Sci. 2010; 350:44–50. DOI: 10.1016/j.jcis.2010.05.035. PMID: 20624622.


76. Pathakoti K, Hwang HM, Xu H, Aguilar ZP, Wang A. In vitro cytotoxicity of CdSe/ZnS quantum dots with different surface coatings to human keratinocytes HaCaT cells. J Environ Sci (China). 2013; 25:163–171. DOI: 10.1016/S1001-0742(12)60015-1. PMID: 23586311.


77. Hughes GA. Nanostructure-mediated drug delivery. Balogh LP, editor. Nanomedicine. Singapore: Pan Stanford Publishing;2017. p. 47–72.


78. Aulenta F, Hayes W, Rannard S. Dendrimers: a new class of nanoscopic containers and delivery devices. Eur Polym J. 2003; 39:1741–1771. DOI: 10.1016/S0014-3057(03)00100-9.


79. Najlah M, D’Emanuele A. Crossing cellular barriers using dendrimer nanotechnologies. Curr Opin Pharmacol. 2006; 6:522–527. DOI: 10.1016/j.coph.2006.05.004. PMID: 16890022.


80. Oliveira JM, Salgado AJ, Sousa N, Mano JF, Reis RL. Dendrimers and derivatives as a potential therapeutic tool in regenerative medicine strategies--a review. Prog Polym Sci. 2010; 35:1163–1194. DOI: 10.1016/j.progpolymsci.2010.04.006.


81. Pillai O, Panchagnula R. Polymers in drug delivery. Curr Opin Chem Biol. 2001; 5:447–451. DOI: 10.1016/S1367-5931(00)00227-1. PMID: 11470609.


82. Pissuwan D, Valenzuela SM, Cortie MB. Therapeutic possibilities of plasmonically heated gold nanoparticles. Trends Biotechnol. 2006; 24:62–67. DOI: 10.1016/j.tibtech.2005.12.004. PMID: 16380179.


83. Mahmoudi M, Sant S, Wang B, Laurent S, Sen T. Superparamagnetic iron oxide nanoparticles (SPIONs): development, surface modification and applications in chemotherapy. Adv Drug Deliv Rev. 2011; 63:24–46. DOI: 10.1016/j.addr.2010.05.006. PMID: 20685224.


84. Kodama R. Magnetic nanoparticles. J Magn Magn Mater. 1999; 200:359–372. DOI: 10.1016/S0304-8853(99)00347-9.


85. Neuberger T, Schöpf B, Hofmann H, Hofmann M, von Rechenberg B. Superparamagnetic nanoparticles for biomedical applications: possibilities and limitations of a new drug delivery system. J Magn Mater. 2005; 293:483–496. DOI: 10.1016/j.jmmm.2005.01.064.


86. Syková E, Jendelová P. Migration, fate and in vivo imaging of adult stem cells in the CNS. Cell Death Differ. 2007; 14:1336–1342. DOI: 10.1038/sj.cdd.4402140. PMID: 17396130.


87. Magrez A, Kasas S, Salicio V, Pasquier N, Seo JW, Celio M, Catsicas S, Schwaller B, Forró L. Cellular toxicity of carbon-based nanomaterials. Nano Lett. 2006; 6:1121–1125. DOI: 10.1021/nl060162e. PMID: 16771565.


88. Zhang BT, Zheng X, Li HF, Lin JM. Application of carbon-based nanomaterials in sample preparation: a review. Anal Chim Acta. 2013; 784:1–17. DOI: 10.1016/j.aca.2013.03.054. PMID: 23746402.


89. Scida K, Stege PW, Haby G, Messina GA, García CD. Recent applications of carbon-based nanomaterials in analytical chemistry: critical review. Anal Chim Acta. 2011; 691:6–17. DOI: 10.1016/j.aca.2011.02.025. PMID: 21458626. PMCID: PMC3088727.


90. Cha C, Shin SR, Annabi N, Dokmeci MR, Khademhosseini A. Carbon-based nanomaterials: multifunctional materials for biomedical engineering. ACS Nano. 2013; 7:2891–2897. DOI: 10.1021/nn401196a. PMID: 23560817. PMCID: PMC3648999.


91. Lasic DD. Novel applications of liposomes. Trends Biotechnol. 1998; 16:307–321. DOI: 10.1016/S0167-7799(98)01220-7. PMID: 9675915.


92. Fenske DB, Chonn A, Cullis PR. Liposomal nanomedicines: an emerging field. Toxicol Pathol. 2008; 36:21–29. DOI: 10.1177/0192623307310960. PMID: 18337218.


93. Lyakhovich A, Lleonart ME. Bypassing mechanisms of mitochondria-mediated cancer stem cells resistance to chemo-and radiotherapy. Oxid Med Cell Longev. 2016; 2016:1716341. DOI: 10.1155/2016/1716341. PMID: 26697128. PMCID: PMC4677234.
94. Hong IS, Jang GB, Lee HY, Nam JS. Targeting cancer stem cells by using the nanoparticles. Int J Nanomedicine. 2015; 10:251–260. PMID: 26425092. PMCID: PMC4583536.
95. Abetov D, Mustapova Z, Saliev T, Bulanin D, Batyrbekov K, Gilman CP. Novel small molecule inhibitors of cancer stem cell signaling pathways. Stem Cell Rev. 2015; 11:909–918. DOI: 10.1007/s12015-015-9612-x. PMID: 26210995.


96. He L, Gu J, Lim LY, Yuan ZX, Mo J. Nanomedicine-mediated therapies to target breast cancer stem cells. Front Pharmacol. 2016; 7:313. DOI: 10.3389/fphar.2016.00313. PMID: 27679576. PMCID: PMC5020043.


97. Wang Z, Tan J, McConville C, Kannappan V, Tawari PE, Brown J, Ding J, Armesilla AL, Irache JM, Mei QB, Tan Y, Liu Y, Jiang W, Bian XW, Wang W. Poly lactic-co-glycolic acid controlled delivery of disulfiram to target liver cancer stem-like cells. Nanomedicine. 2017; 13:641–657. DOI: 10.1016/j.nano.2016.08.001. PMCID: PMC5364371.


98. Estey T, Piatigorsky J, Lassen N, Vasiliou V. ALDH3A1: a corneal crystallin with diverse functions. Exp Eye Res. 2007; 84:3–12. DOI: 10.1016/j.exer.2006.04.010. PMID: 16797007.


99. Ginestier C, Hur MH, Charafe-Jauffret E, Monville F, Dutcher J, Brown M, Jacquemier J, Viens P, Kleer CG, Liu S, Schott A, Hayes D, Birnbaum D, Wicha MS, Dontu G. ALDH1 is a marker of normal and malignant human mammary stem cells and a predictor of poor clinical outcome. Cell Stem Cell. 2007; 1:555–567. DOI: 10.1016/j.stem.2007.08.014. PMID: 18371393. PMCID: PMC2423808.


100. Lu B, Huang X, Mo J, Zhao W. Drug delivery using nanoparticles for cancer stem-like cell targeting. Front Pharmacol. 2016; 7:84. DOI: 10.3389/fphar.2016.00084. PMID: 27148051. PMCID: PMC4828437.


101. Li D, Zhang T, Gu W, Li P, Cheng X, Tong T, Wang W. The ALDH1+ subpopulation of the human NMFH-1 cell line exhibits cancer stem-like characteristics. Oncol Rep. 2015; 33:2291–2298. DOI: 10.3892/or.2015.3842. PMID: 25760144.


102. Liu SY, Zheng PS. High aldehyde dehydrogenase activity identifies cancer stem cells in human cervical cancer. Oncotarget. 2013; 4:2462–2475. DOI: 10.18632/oncotarget.1578. PMID: 24318570. PMCID: PMC3926841.


103. Shao C, Sullivan JP, Girard L, Augustyn A, Yenerall P, Rodriguez-Canales J, Liu H, Behrens C, Shay JW, Wistuba II, Minna JD. Essential role of aldehyde dehydrogenase 1A3 for the maintenance of non-small cell lung cancer stem cells is associated with the STAT3 pathway. Clin Cancer Res. 2014; 20:4154–4166. DOI: 10.1158/1078-0432.CCR-13-3292. PMID: 24907115. PMCID: PMC4438754.


104. Yue L, Huang ZM, Fong S, Leong S, Jakowatz JG, Charruyer-Reinwald A, Wei M, Ghadially R. Targeting ALDH1 to decrease tumorigenicity, growth and metastasis of human melanoma. Melanoma Res. 2015; 25:138–148. DOI: 10.1097/CMR.0000000000000144. PMID: 25643237.


105. Vira D, Basak SK, Veena MS, Wang MB, Batra RK, Srivatsan ES. Cancer stem cells, microRNAs, and therapeutic strategies including natural products. Cancer Metastasis Rev. 2012; 31:733–751. DOI: 10.1007/s10555-012-9382-8. PMID: 22752409.


106. Yip NC, Fombon IS, Liu P, Brown S, Kannappan V, Armesilla AL, Xu B, Cassidy J, Darling JL, Wang W. Disulfiram modulated ROS-MAPK and NFκB pathways and targeted breast cancer cells with cancer stem cell-like properties. Br J Cancer. 2011; 104:1564–1574. DOI: 10.1038/bjc.2011.126. PMID: 21487404. PMCID: PMC3101904.


107. Chen D, Cui QC, Yang H, Dou QP. Disulfiram, a clinically used anti-alcoholism drug and copper-binding agent, induces apoptotic cell death in breast cancer cultures and xenografts via inhibition of the proteasome activity. Cancer Res. 2006; 66:10425–10433. DOI: 10.1158/0008-5472.CAN-06-2126. PMID: 17079463.


108. Kast RE, Boockvar JA, Brüning A, Cappello F, Chang WW, Cvek B, Dou QP, Duenas-Gonzalez A, Efferth T, Focosi D, Ghaffari SH, Karpel-Massler G, Ketola K, Khoshnevisan A, Keizman D, Magné N, Marosi C, McDonald K, Muñoz M, Paranjpe A, Pourgholami MH, Sardi I, Sella A, Srivenugopal KS, Tuccori M, Wang W, Wirtz CR, Halatsch ME. A conceptually new treatment approach for relapsed glioblastoma: coordinated undermining of survival paths with nine repurposed drugs (CUSP9) by the International Initiative for Accelerated Improvement of Glioblastoma Care. Oncotarget. 2013; 4:502–530. DOI: 10.18632/oncotarget.969. PMID: 23594434. PMCID: PMC3720600.


109. Hothi P, Martins TJ, Chen L, Deleyrolle L, Yoon JG, Reynolds B, Foltz G. High-throughput chemical screens identify disulfiram as an inhibitor of human glioblastoma stem cells. Oncotarget. 2012; 3:1124–1136. DOI: 10.18632/oncotarget.707. PMID: 23165409. PMCID: PMC3717950.


110. Wang W, McLeod HL, Cassidy J. Disulfiram-mediated inhibition of NF-kappaB activity enhances cytotoxicity of 5-fluorouracil in human colorectal cancer cell lines. Int J Cancer. 2003; 104:504–511. DOI: 10.1002/ijc.10972. PMID: 12584750.


111. Guo X, Xu B, Pandey S, Goessl E, Brown J, Armesilla AL, Darling JL, Wang W. Disulfiram/copper complex inhibiting NFkappaB activity and potentiating cytotoxic effect of gemcitabine on colon and breast cancer cell lines. Cancer Lett. 2010; 290:104–113. DOI: 10.1016/j.canlet.2009.09.002. PMID: 19782464.


112. Liu P, Brown S, Goktug T, Channathodiyil P, Kannappan V, Hugnot JP, Guichet PO, Bian X, Armesilla AL, Darling JL, Wang W. Cytotoxic effect of disulfiram/copper on human glioblastoma cell lines and ALDH-positive cancer-stem-like cells. Br J Cancer. 2012; 107:1488–1497. DOI: 10.1038/bjc.2012.442. PMID: 23033007. PMCID: PMC3493777.


113. Zöller M. CD44: can a cancer-initiating cell profit from an abundantly expressed molecule? Nat Rev Cancer. 2011; 11:254–267. DOI: 10.1038/nrc3023. PMID: 21390059.


114. Al-Hajj M, Wicha MS, Benito-Hernandez A, Morrison SJ, Clarke MF. Prospective identification of tumorigenic breast cancer cells. Proc Natl Acad Sci U S A. 2003; 100:3983–3988. DOI: 10.1073/pnas.0530291100. PMID: 12629218. PMCID: PMC153034.


115. Dalerba P, Dylla SJ, Park IK, Liu R, Wang X, Cho RW, Hoey T, Gurney A, Huang EH, Simeone DM, Shelton AA, Parmiani G, Castelli C, Clarke MF. Phenotypic characterization of human colorectal cancer stem cells. Proc Natl Acad Sci U S A. 2007; 104:10158–10163. DOI: 10.1073/pnas.0703478104. PMID: 17548814. PMCID: PMC1891215.


116. Deonarain MP, Kousparou CA, Epenetos AA. Antibodies targeting cancer stem cells: a new paradigm in immunotherapy? MAbs. 2009; 1:12–25. DOI: 10.4161/mabs.1.1.7347. PMID: 20046569. PMCID: PMC2715180.


117. Gener P, Gouveia LP, Sabat GR, de Sousa Rafael DF, Fort NB, Arranja A, Fernández Y, Prieto RM, Ortega JS, Arango D, Abasolo I, Videira M, Schwartz S Jr. Fluorescent CSC models evidence that targeted nanomedicines improve treatment sensitivity of breast and colon cancer stem cells. Nanomedicine. 2015; 11:1883–1892. DOI: 10.1016/j.nano.2015.07.009. PMID: 26238079.


118. Arabi L, Badiee A, Mosaffa F, Jaafari MR. Targeting CD44 expressing cancer cells with anti-CD44 monoclonal antibody improves cellular uptake and antitumor efficacy of liposomal doxorubicin. J Control Release. 2015; 220:275–286. DOI: 10.1016/j.jconrel.2015.10.044. PMID: 26518722.


119. Aires A, Ocampo SM, Simões BM, Josefa Rodríguez M, Cadenas JF, Couleaud P, Spence K, Latorre A, Miranda R, Somoza Á, Clarke RB, Carrascosa JL, Cortajarena AL. Multifunctionalized iron oxide nanoparticles for selective drug delivery to CD44-positive cancer cells. Nanotechnology. 2016; 27:065103. DOI: 10.1088/0957-4484/27/6/065103. PMID: 26754042.


120. Ni M, Xiong M, Zhang X, Cai G, Chen H, Zeng Q, Yu Z. Poly(lactic-co-glycolic acid) nanoparticles conjugated with CD133 aptamers for targeted salinomycin delivery to CD133+ osteosarcoma cancer stem cells. Int J Nanomedicine. 2015; 10:2537–2554. PMID: 25848270. PMCID: PMC4386781.
121. Qi X, Yu D, Jia B, Jin C, Liu X, Zhao X, Zhang G. Targeting CD133(+) laryngeal carcinoma cells with chemotherapeutic drugs and siRNA against ABCG2 mediated by thermo/pH-sensitive mesoporous silica nanoparticles. Tumour Biol. 2016; 37:2209–2217. DOI: 10.1007/s13277-015-4007-9. PMID: 26353857.


122. Li Y, Zhang T. Targeting cancer stem cells by curcumin and clinical applications. Cancer Lett. 2014; 346:197–205. DOI: 10.1016/j.canlet.2014.01.012. PMID: 24463298.


123. Lim KJ, Bisht S, Bar EE, Maitra A, Eberhart CG. A polymeric nanoparticle formulation of curcumin inhibits growth, clonogenicity and stem-like fraction in malignant brain tumors. Cancer Biol Ther. 2011; 11:464–473. DOI: 10.4161/cbt.11.5.14410. PMID: 21193839. PMCID: PMC3087900.


124. Mumm JS, Kopan R. Notch signaling: from the outside in. Dev Biol. 2000; 228:151–165. DOI: 10.1006/dbio.2000.9960. PMID: 11112321.


125. Tang SN, Fu J, Nall D, Rodova M, Shankar S, Srivastava RK. Inhibition of sonic hedgehog pathway and pluripotency maintaining factors regulate human pancreatic cancer stem cell characteristics. Int J Cancer. 2012; 131:30–40. DOI: 10.1002/ijc.26323. PMID: 21796625. PMCID: PMC3480310.


126. Fan X, Matsui W, Khaki L, Stearns D, Chun J, Li YM, Eberhart CG. Notch pathway inhibition depletes stem-like cells and blocks engraftment in embryonal brain tumors. Cancer Res. 2006; 66:7445–7452. DOI: 10.1158/0008-5472.CAN-06-0858. PMID: 16885340.


127. Mamaeva V, Rosenholm JM, Bate-Eya LT, Bergman L, Peuhu E, Duchanoy A, Fortelius LE, Landor S, Toivola DM, Lindén M, Sahlgren C. Mesoporous silica nanoparticles as drug delivery systems for targeted inhibition of Notch signaling in cancer. Mol Ther. 2011; 19:1538–1546. DOI: 10.1038/mt.2011.105. PMID: 21629222. PMCID: PMC3149161.


128. Mamaeva V, Niemi R, Beck M, Özliseli E, Desai D, Landor S, Gronroos T, Kronqvist P, Pettersen IK, McCormack E, Rosenholm JM, Linden M, Sahlgren C. Inhibiting notch activity in breast cancer stem cells by glucose functionalized nanoparticles carrying γ-secretase inhibitors. Mol Ther. 2016; 24:926–936. DOI: 10.1038/mt.2016.42. PMID: 26916284. PMCID: PMC4881775.


129. Yang ZF, Ngai P, Ho DW, Yu WC, Ng MN, Lau CK, Li ML, Tam KH, Lam CT, Poon RT, Fan ST. Identification of local and circulating cancer stem cells in human liver cancer. Hepatology. 2008; 47:919–928. DOI: 10.1002/hep.22082. PMID: 18275073.


130. Bakalova R, Ohba H, Zhelev Z, Ishikawa M, Baba Y. Quantum dots as photosensitizers? Nat Biotechnol. 2004; 22:1360–1361. DOI: 10.1038/nbt1104-1360. PMID: 15529155.


131. Ruiz i Altaba A, Sánchez P, Dahmane N. Gli and hedgehog in cancer: tumours, embryos and stem cells. Nat Rev Cancer. 2002; 2:361–372. DOI: 10.1038/nrc796. PMID: 12044012.


132. Rubin LL, de Sauvage FJ. Targeting the Hedgehog pathway in cancer. Nat Rev Drug Discov. 2006; 5:1026–1033. DOI: 10.1038/nrd2086. PMID: 17139287.


133. Kiesslich T, Berr F, Alinger B, Kemmerling R, Pichler M, Ocker M, Neureiter D. Current status of therapeutic targeting of developmental signalling pathways in oncology. Curr Pharm Biotechnol. 2012; 13:2184–2220. DOI: 10.2174/138920112802502114. PMID: 21605074.


134. Chenna V, Hu C, Pramanik D, Aftab BT, Karikari C, Campbell NR, Hong SM, Zhao M, Rudek MA, Khan SR, Rudin CM, Maitra A. A polymeric nanoparticle encapsulated small-molecule inhibitor of Hedgehog signaling (NanoHHI) bypasses secondary mutational resistance to Smoothened antagonists. Mol Cancer Ther. 2012; 11:165–173. DOI: 10.1158/1535-7163.MCT-11-0341. PMID: 22027695. PMCID: PMC3256300.


135. Xu Y, Chenna V, Hu C, Sun HX, Khan M, Bai H, Yang XR, Zhu QF, Sun YF, Maitra A, Fan J, Anders RA. Polymeric nanoparticle-encapsulated hedgehog pathway inhibitor HPI-1 (NanoHHI) inhibits systemic metastases in an orthotopic model of human hepatocellular carcinoma. Clin Cancer Res. 2012; 18:1291–1302. DOI: 10.1158/1078-0432.CCR-11-0950. PMID: 21868763. PMCID: PMC3233659.


136. Verma RK, Yu W, Singh SP, Shankar S, Srivastava RK. Anthothecol-encapsulated PLGA nanoparticles inhibit pancreatic cancer stem cell growth by modulating sonic hedgehog pathway. Nanomedicine. 2015; 11:2061–2070. DOI: 10.1016/j.nano.2015.07.001. PMID: 26199979.


137. Moses H, Barcellos-Hoff MH. TGF-beta biology in mammary development and breast cancer. Cold Spring Harb Perspect Biol. 2011; 3:a003277. DOI: 10.1101/cshperspect.a003277. PMID: 20810549. PMCID: PMC3003461.
138. Bellam N, Pasche B. Tgf-beta signaling alterations and colon cancer. Cancer Treat Res. 2010; 155:85–103. DOI: 10.1007/978-1-4419-6033-7_5. PMID: 20517689.
139. Wu K, Ding J, Chen C, Sun W, Ning BF, Wen W, Huang L, Han T, Yang W, Wang C, Li Z, Wu MC, Feng GS, Xie WF, Wang HY. Hepatic transforming growth factor beta gives rise to tumor-initiating cells and promotes liver cancer development. Hepatology. 2012; 56:2255–2267. DOI: 10.1002/hep.26007. PMID: 22898879.


140. Ischenko I, Liu J, Petrenko O, Hayman MJ. Transforming growth factor-beta signaling network regulates plasticity and lineage commitment of lung cancer cells. Cell Death Differ. 2014; 21:1218–1228. DOI: 10.1038/cdd.2014.38. PMID: 24682004. PMCID: PMC4085528.


141. Gao J, Zhu Y, Nilsson M, Sundfeldt K. TGF-β isoforms induce EMT independent migration of ovarian cancer cells. Cancer Cell Int. 2014; 14:72. DOI: 10.1186/s12935-014-0072-1. PMID: 25278811. PMCID: PMC4180856.


142. Liu Z, Bandyopadhyay A, Nichols RW, Wang L, Hinck AP, Wang S, Sun LZ. Blockade of autocrine TGF-β signaling inhibits stem cell phenotype, survival, and metastasis of murine breast cancer cells. J Stem Cell Res Ther. 2012; 2:1–8. DOI: 10.4172/2157-7633.1000116. PMID: 23482850. PMCID: PMC3593047.


143. Mishra L, Shetty K, Tang Y, Stuart A, Byers SW. The role of TGF-beta and Wnt signaling in gastrointestinal stem cells and cancer. Oncogene. 2005; 24:5775–5789. DOI: 10.1038/sj.onc.1208924. PMID: 16123810.


144. Amin R, Mishra L. Liver stem cells and tgf-Beta in hepatic carcinogenesis. Gastrointest Cancer Res. 2008; 2(4 Suppl):S27–S30. PMID: 19343145. PMCID: PMC2661545.
145. Gomez-Casal R, Bhattacharya C, Ganesh N, Bailey L, Basse P, Gibson M, Epperly M, Levina V. Non-small cell lung cancer cells survived ionizing radiation treatment display cancer stem cell and epithelial-mesenchymal transition phenotypes. Mol Cancer. 2013; 12:94. DOI: 10.1186/1476-4598-12-94. PMID: 23947765. PMCID: PMC3751356.


146. Meng H, Zhao Y, Dong J, Xue M, Lin YS, Ji Z, Mai WX, Zhang H, Chang CH, Brinker CJ, Zink JI, Nel AE. Two-wave nanotherapy to target the stroma and optimize gemcitabine delivery to a human pancreatic cancer model in mice. ACS Nano. 2013; 7:10048–10065. DOI: 10.1021/nn404083m. PMID: 24143858. PMCID: PMC3878438.


147. Zuo ZQ, Chen KG, Yu XY, Zhao G, Shen S, Cao ZT, Luo YL, Wang YC, Wang J. Promoting tumor penetration of nanoparticles for cancer stem cell therapy by TGF-β signaling pathway inhibition. Biomaterials. 2016; 82:48–59. DOI: 10.1016/j.biomaterials.2015.12.014. PMID: 26751819.


148. Tsai YS, Chen YH, Cheng PC, Tsai HT, Shiau AL, Tzai TS, Wu CL. TGF-β1 conjugated to gold nanoparticles results in protein conformational changes and attenuates the biological function. Small. 2013; 9:2119–2128. DOI: 10.1002/smll.201202755. PMID: 23335450.


149. Singh VK, Saini A, Chandra R. The implications and future perspectives of nanomedicine for cancer stem cell targeted therapies. Front Mol Biosci. 2017; 4:52. DOI: 10.3389/fmolb.2017.00052. PMID: 28785557. PMCID: PMC5520001.


150. Zhao Y, Alakhova DY, Kabanov AV. Can nanomedicines kill cancer stem cells? Adv Drug Deliv Rev. 2013; 65:1763–1783. DOI: 10.1016/j.addr.2013.09.016. PMID: 24120657. PMCID: PMC4174448.


151. Youn YS, Bae YH. Perspectives on the past, present, and future of cancer nanomedicine. Adv Drug Deliv Rev. 2018; 130:3–11. DOI: 10.1016/j.addr.2018.05.008. PMID: 29778902.


152. Tabassum N, Verma V, Kumar M, Kumar A, Singh B. Nanomedicine in cancer stem cell therapy: from fringe to forefront. Cell Tissue Res. 2018; 374:427–438. DOI: 10.1007/s00441-018-2928-5. PMID: 30302547.


Fig. 1
CSCs resistance to treatment can be obtained by, expression of ABC drug pumps, increased expression of anti-apoptotic proteins, resistance to DNA damaging agents, and slow cycle kinetics.
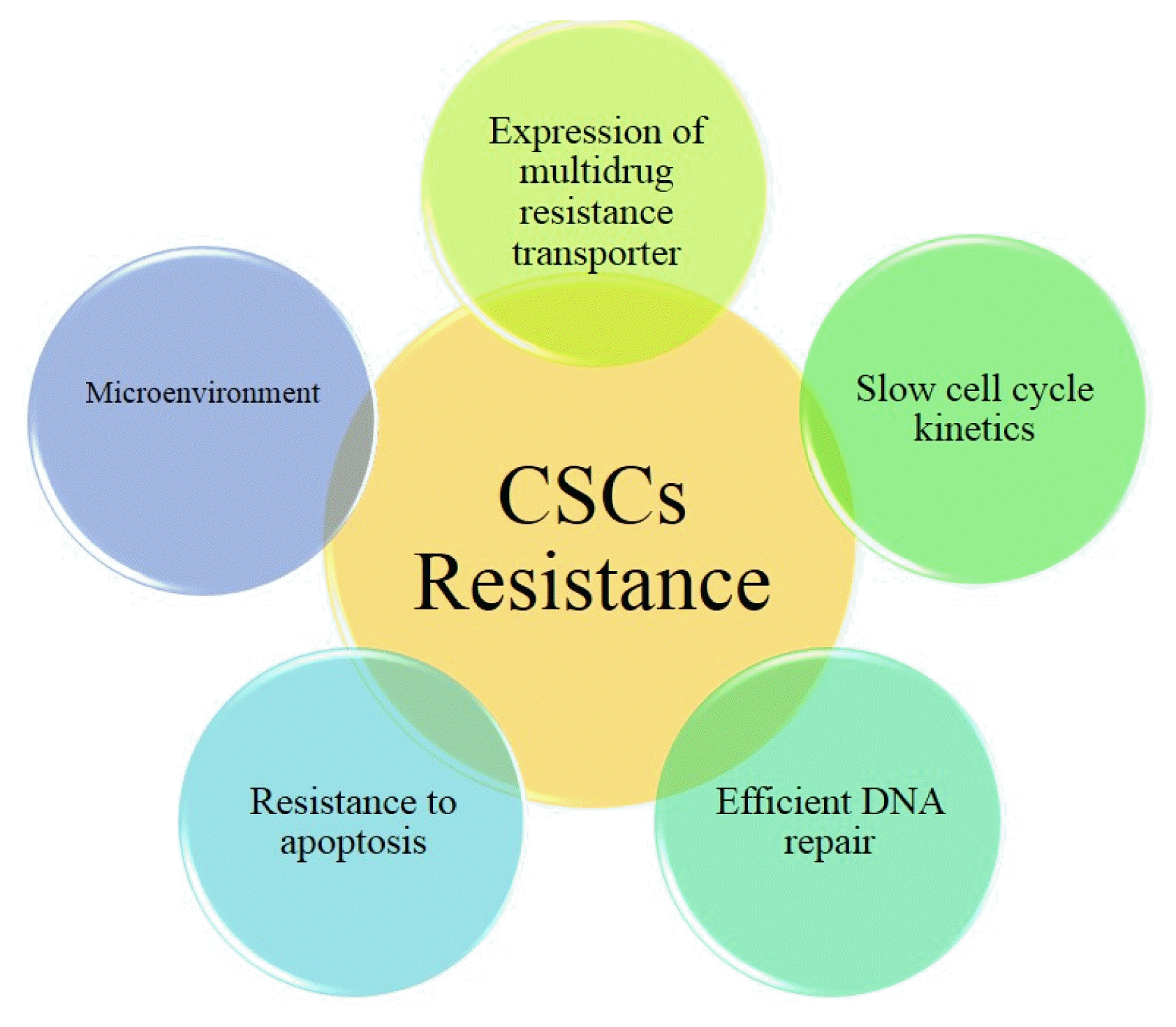
Table 1
Cancer stem cell markers in a variety of solid tumors
Type of cancers | Markers | References |
---|---|---|
Leukemia | CD34+/CD38−/CD96 | (10, 15, 16) |
Breast cancer | CD44+/ESA+/CD24−/ALDH1 | (10, 12, 17) |
Brain cancer | CD133+ | (18, 19) |
Multiple myelomas | CD138− | (20, 21) |
Pancreatic cancer | CD44+/CD24+/ESA+ | (6, 22) |
Colon cancer | CD133+ | (23) |
Liver cancer | CD133+/CD90+ | (24, 25) |
Prostate cancer | CD44+/CD133+ | (26, 27) |
Lung cancer | CD133+ | (28) |
Ovarian cancer | CD133+/CD44+/CD117+/CD24+ | (29–31) |
Table 2
Various nanomaterials for therapeutic applications
Nanomaterial | Ligand | Target | References |
---|---|---|---|
Quantum dot | HER2/neusiRNA | SKBR3 | (60) |
Quantum dot/Chitosan | DNA aptamer | A2780/AD | (61) |
Quantum dot | HER2; RNase A | MGC-803 | (62) |
PAMAM | Tf and Tm | glioma Dual-targeting | (63) |
PAMAM | Biotin | HeLa | (64) |
SPIONs-αv β3 | Anti αv antibody RGD peptides | αv β3 Integrin Breast cancer | (65) |
SPIONs-PEG-Ab | Monoclonal Ab A7 | Colorectal carcinoma | (66) |
Gold nanoparticle | Folic acid | Ovarian cancer | (67) |
Gold nanoparticle | Bombesinpeptides | Human prostate cancer cells | (68) |
Gold nanoparticle | Transferrin | Hs578T (breast cancer) and a nonmalignant cell lines (3T3) | (69) |
MWCNT | Folate (biologically active targeting) and iron (magnetically passive) targeting | HeLa | (70) |
SWCNT | Hyaluronic acid Salinomycin Gastric | cancer stem cells (CSCs) | (71) |
Graphene oxide | Rituxan (CD201 antibody) | CEM.NK T-cell and Raji B-cell | (72) |
Nanoliposome | Anti-HER2 | HER2- overexpressing breast cancer | (73) |