Abstract
Background and Objectives:
Cell therapy provides an effective strategy for the treatment of an impaired liver. Human umbilical cord blood progenitor cells have the potential to differentiate into hepatocytes. Progenitor cells transplanted into the spleen could migrate directly into the liver through portal circulation. To track migration of human umbilical cord blood progenitor cells in cirrhotic rat liver after intrasplenic transplantation and to prove the possibility similar behavior of human umbilical cord blood nucleated cells in humans.
Methods and Results:
Umbilical cord blood samples from full-term deliveries will be collected after obtaining an informed consent from the mother. The collection procedure will be conducted after completion of delivery and will not interfere with the normal obstetric procedures. Adult male Sprague Dawley rats were subjected to liver cirrhosis by intraperitoneal injection of thioacetamide. Cirrhotic rats were treated with human umbilical cord blood nucleated cells by intra-splenic transplantation. Migration of intrasplenic transplanted human umbilical cord blood cells to the liver was successfully documented with Immunohistochemistry. The liver and spleen from recipient animals were removed. Histopathological and immunohistochemical analysis were performed 20 weeks after intrasplenic injection of the cells. Intrasplenically injected cells migrate to the liver of recipient animals.
Conclusions:
Human cord blood nucleated cells have the potential to differentiate into hepatocytes and substantially improve the histology and function of the cirrhotic liver in rats. Relocation into liver after intrasplenic transplantation could be detected by immunohistochemistry. Transdifferentiated cells could be efficiently stained with antihuman hepatocytes.
Cell transplantation provides an effective strategy for the treatment of an impaired liver or liver failure. When compared with orthotopic liver transplantation, stem cell transplantation has the advantages of lower cost, lower risk, and simpler manipulation of the procedure. Autologous cell transplantation helps prevent immunologic rejection, which is always a problem for orthotopic liver transplantation, and it bears great potential usefulness as a transient therapy before liver transplantation (1).
Exploitation of high regeneration and differentiation of stem cells may solve this problem. Transdifferentiation of different kinds of stem cells into hepatocytes has been previously demonstrated with embryonic stem cells, hepatoblasts (mostly in fetal liver), hepatic oval cells (mostly in mature liver), pancreatic progenitor cells, bone marrow hematopoietic stem cells, and mesenchymal stem cells (MSCs) (2–5). Bone marrow-derived stem cells exhibited better prospects in terms of practical applicability as surrogates of hepatocytes for transplantation in MSCs. Both in vitro and in vivo, studies appeared to support bone MSCs (BMSCs) as being more potent in hepatocytic trans-differentiation (6–9).
In the late 1980s and early 1990s, physicians began to recognize that blood from the human Umbilical cord and placenta was a rich source of stem cells. This tissue supports the developing fetus during pregnancy, is delivered along with the baby, and, is usually discarded. Since the first successful umbilical cord blood transplants in children with Fanconi anemia, the collection and therapeutic use of these cells has grown quickly (10). There have been suggestions that umbilical cord blood contains stem cells that have the capability of developing cells of multiple germ layers (multipotent) or even all germ layers, e.g., endoderm, ectoderm, and mesoderm (11).
The cells can be delivered into the recipient using one of the following routes; systemic, intraperitoneal, intrahepatic or intrasplenic.
Peripheral infusion is an easy and convenient way of stem cells delivery, with less invasive and non-traumatic, without the need of advanced medical equipment, therefore most stem cell implantations are done via this strategy (12).
However, as a systemic administration, there are concerns of side effects, such as fever and immune reaction. Moreover, systemic intravenous delivery of stem cells may cause entrapment of donor cells in the lungs (13). Pretreatment with the vasodilator sodium nitroprusside may reduce the number of cells entrapped (14).
The peritoneal cavity has long been used as a favorable site for therapeutic cell transplantation, with the advantage that large volume of cells can be implanted, technically easy to perform, less traumatic and less invasive, and no concern of organ embolism (15). In addition, in the circumstances of liver disease, proliverproliferation growth factors and cytokines produced from transplanted cells can be drained directly to the portal venous system, thus enhancing liver regeneration (15).
Intrahepatic injection of stem cells is another route of local administration. When fractionated human mesenchymal stem cells, CD34+cells (5), where directly xenografted to allylalcohol (AA) treated rat liver by intrahepatic injection, human hepatocyte like cells, evidenced by positive human AFP, albumen, cytokeratin (8), cytokeratin (18) were observed in recipient livers with mesenchymal stem cell fractions (16). In the congenital absence of albumin in Nagase analbuminemic rats (NARs), liver infusion of allogenic bone marrow stem cells (BMSCs), eight weeks later, the serum albumin levels demonstrated significantly higher levels to the control group. On the mean time, the hepatocyte-like cells expressing albumin in the liver of NARs were detected indicating that BMSCs can differentiate into hepatocyte-like cells when infused intrahepatically (16).
Direct hepatic infusion of stem cells would be favorable for the cells repopulating in the liver, however, the procedure is complicated and invasive, severe trauma may occur, in addition, in diseased liver, the microenvironment of the liver may not be amenable for the stem cells to re-populate and function (17). Stem cells infused from portal vein may reside in the periportal areas and repopulate quickly compared to the peripheral systemic infusion (18). In a clinical setting, intraportal infusion of autologous BMSCs subsequent to portal venous embolization of the right liver segments, used to expand the left lateral hepatic segments, results showed a 2.5 folds increased mean proliferation rates of the left lateral segments compared to the conrol group. These data highlights the therapeutic potential of intraportal injection of autologous BMSCs for accelerating hepatic regeneration (19).
Stem cells infusion via portal vein may have some disadvantages. Firstly, the procedure is complicated and invasive. Secondly, it may cause portal hypertension and further liver damage. Finally, transplanted cells may migrate to systemic veins and cause embolism of other organs, such as lung and brain (20).
The intraportal method of administration has obvious advantages, however, the liver carries the inherent disadvantage that is a vital organ and can’t be removed if problems arise following transplantation, in this regard, the spleen has unique anatomic affinity with the liver, when the diseased liver is not suitable for stem cells transplantation, intrasplenic transplantation may retain the cells in the spleen and implement the functions. Blood in spleen directly drains to liver, so in intrasplenic transplantation, the factors and cytokines drains to the remnant liver, this maintains the factors in high concentration in portal system and in remnant liver, so enhances the remnant liver regeneration (20). Intrasplenic transplantation has long been used as a route of cell delivery for liver diseases. Intrasplenic transplantation of hepatocytes increased survival of the totally hepatectomized rats (21). Intrasplenic transplantation of human fetal immortal hepatocytes could prolong survival of 90% hepatectomized rats (22).
However, because splenic vein directly drains to the portal vein, some stem cells transplanted in spleen may reside in spleen, majority of the cells will migrate to liver via portal vein system, this can cause portal vein embolism. Previous reports showed that cells intrasplenically transplanted can cause impairment of hepatic microcirculation and hepatic function, and cause complications in the early transplantation (23). Hence, certain methods have been developed to prevent the intrasplenic transplanted cells from migrating to the systemic circulation, such as the temporary complete occlusion of splenic vessel during infusion may reduce embolization, sudden death and portal hypertension (23).
Since both fulminant and chronic liver failure may require replacement of more than 10% of functional liver, the cell mass required for transplantation into the liver will be significantly higher.
The liver cell mass is restored primarily through division of the majority of mature hepatocytes. A stimulus to regeneration such as two-thirds partial hepatectomy promotes increases in carbamyl phosphate synthetase I activity with subsequent liver hypertrophy. This early experience suggests that this therapeutic approach has the potential of enhancing and accelerating hepatic regeneration in a clinical setting (24). Much of the excitement in stem cell research centers on embryonic stem cells because of their high plasticity, but this approach remains controversial for ethical reasons (25). The injected embryonic stem cells formed teratomas when injected subcutaneously in non obese diabetic/severe combined immunodeficient (NOD/SCID) mice (26). However, adult stem cells from bone marrow (BM) are well characterized and have long been used therapeutically (27).The BM compartment is largely made up of hematopoietic stem cells (HSC) and committed progenitor cells, non-circulating stromal cells (called mesenchymal stem cells (MSC)) that have the ability to develop into mesenchymal lineages (28). HSC are adult stem cells and can be identified by their ability to differentiate into all blood cell types and reconstitute the hematopoietic system in a host that has been lethally myelo ablated (29). It was previously thought that adult stem cells were lineage restricted, but recent studies demonstrated that BM-derived progenitors in addition to haematopoiesis also participate in regeneration of ischemic myocardium, damaged skeletal muscle and neurogenesis (30).
Six-week old male Sprague Dawley rats were used. Liver cirrhosis was induced by intraperitoneal injection of thioacetamide (TAA) 20 mg/kg body weight, twice weekly for 12 weeks. TAA was dissolved in 0.9% NaCl and was injected intraperitoneally according to Horri et a1., 1993 (31). At the end of the 12 weeks, biochemical analysis for liver function tests as well as histopathological analysis of the liver was done to confirm induction of cirrhosis.
Collection of UCB: Human cord blood was obtained from Department of Obstetrics and Gynecology, Ain Shams University and El Galaa teaching hospitals after informed consent obtained from mothers. At the end of the normal delivery process and after the complete separation of the placenta, blood was collected under complete aseptical technique and was transported immediately to the laboratory. Separation procedures were usually performed within 2–3 hours from collection.
Isolation of nucleated cells from UCB: Fifty ml of umbilical cord blood was taken from each sample (n=46) and diluted with an equal volume of phosphate buffer solution (PBS). The diluted umbilical cord blood was layered over an equal volume of Ficoll-Paque (1.077 g/mL-Gibco). Nucleated cells (NCs) were recovered from the gradient interface and washed twice with PBS after centrifugation at 1200 rpm/min for 20 minutes.
The animals were anesthetized using ether inhalation and abdominal incision was done, the spleen was exposed, the cells were injected into the spleen parenchyma over approximately 10∼15 seconds. Using a 26-gauge syringe, cells were injected at a dose of 5×105 cell/ rat in 0.5 ml PBS. The control group received PBS instead of cells. The abdominal incision was closed and the animals were monitored closely until recovery. Eight weeks post-transplantation and before scarification, blood samples were drawn from the inner plexus of the eye and liver function tests were performed. The animals were sacrificed, the liver and spleen were excised, and tissue pieces were taken for histopathology and immunohistochemical studies.
Rat liver and spleen were dissected; several random strips from each lobe were fixed in 10% formalin and embedded in paraffin. Tissue sections 4um thick were taken from each rat and organ. For histopathological examination, sections were stained with H&E as well as Masson trichrome stain for detection of fibrosis. To investigate the hepatic differentiation of cord blood cells injected into the spleen of cirrhotic rats and their liver engraftment, we traced UCB cells by immunostaining analysis with antihuman hepatocyte antibody (Clone OCHIE 5; DACO) as the primary antibody which reacts only with human hepatocytes but not with rat hepatocytes. The sections were deposited on charged glass slides. Slides were dried completely and deparaffinized and rehydrated in xylene and grades of alcohol, the slides were exposed to epitope retrieval by placing them in boiling water containing 0.01 ml/L sodium citrate buffer (PH6) for 15 min. Followed by quenching of endogenous peroxidase with 1% hydrogen peroxide (H202) for 10 minutes. The primary antibody was applied for 24h in humid chamber. The primary antibody was detected using ABC complex and developed with DAB as substrate the slides were counterstained with hematoxylin, mounted in DPX and covered with cover slips.
In our study mortalities between animals during the induction of cirrhosis reached 60%. There was elevation of the liver enzymes and impairment of the synthetic functions of the liver represented by the significant decrease in serum albumin and the significant rise of total bilirubin and INR of cirrhotic; these results confirm the injurious effect of Thioacetamide on hepatocytes rats. Gross examination of livers retrieved after animal sacrifice showed yellow firm, shrunken liver with nodular surface, cut section showed yellow cirrhotic nodules. Histopathological examination revealed distorted architecture, ductular proliferation and regenerative parenchymatous nodules surrounded by fibrous connective tissue septa Fig. 1A. In this study, we injected nucleated cells derived from UCB into the spleen of immunocompetent rats after induction of cirrhosis without immunosupression. The histological sections didn’t show significant inflammatory aggregates indicating that graft was not rejected. No apoptosis was detected indicating that implanted cells survived in the recipient tissues. Histopathologic examination also showed significantly decreased fibrosis and marked liver regeneration as well as better restoration of architecture as shown by H&E (Fig. 1B).
Liver sections of transplanted animals were stained positive for anti-human hepatocyte antibodies (Fig. 2A–C), which stains only human hepatocytes, while none of the control animals showed such staining (Fig. 2D). Sections of spleens of the recipient animals showed no human hepatocytes by immunostaining (Fig. 2E, F) indicating that UCBSCs had migrated to the liver and engrafted, multiplied and differentiated into human hepatocytes.
Cell therapy can be defined as ≪the use of living cells to restore, maintain or enhance the function of tissues and organs≫ (32).Such a therapeutic strategy was put forward as an alternative to orthotopic liver transplantation (OLT), which requires major surgery and is limited by the availability of donors. Indeed, it was shown that significant clinical results can be obtained with the transplantation of isolated hepatocytes corresponding to as little as 1–5% of the total hepatocyte mass (33). The procedure seems relatively safe, provided portal pressure and/or portal flow are monitored during cell infusion in order to prevent vascular thrombosis (34).
Hepatocyte transplantation has recently been used as an alternative to OLT in patients with liver-based congenital metabolic disorders, such as Crigler-Najjar disease, Alpha 1-antitrypsin deficiency, glycogen storage disease type Ia, Ornithine transcarbamoylase deficiency, and the deficiency of factor VII.
The main factor limiting the practice of hepatocyte transplantation is again the availability of liver grafts for cell isolation. Moreover, the metabolic effects of cell trans-plantation seem to be fading with time, a problem which can partially be solved by repeated hepatocyte infusions, but which probably indicates the progressive loss of the terminally-differentiated exogenous cells. In theory, both problems could be solved by replacing the hepatocyte with stem or precursor cells, provided they can be isolated from a more affordable source (35).
Stem cells present in adult BM and UCB are undifferentiated long-lived cells that have the ability to proliferate extensively and maintain the ability to differentiate into multiple cell types including bone cells, cartilage cells, fat cells, tendon cells, muscle cells, marrow stromal cells, astrocytes, etc. Potential liver-cell progenitors have been identified from BM, peripheral blood, UCB, fetal liver, adult liver and embryonic stem cells (36). Stem cells are present in UCB and possess several typical traits. Clonal culture of fluorescence-activated cell sorter CD34+ UCB and BM cells revealed a higher incidence of colony-forming cells with greater proliferation capacity in UCB than BM CD34+ cells. UCB CD34+ cells also demonstrated a higher secondary plating efficiency than BM cells. Rats transplanted with UCB mononuclear cells showed significantly higher levels of chimerism than those transplanted with BM mononuclear cells. Recipients of UCB transplants from HLA-identical siblings have a lower incidence of acute and chronic graft versus-host disease than recipients of BM transplants from HLA-identical siblings. CD34+ UCB cells are rich fractions in hepatic progenitor cells, and transdifferentiation from UCB cells into hepatocytes and cell fusion simultaneously occur (37). In a primary culture system supplemented with growth/differentiation factors, about 50% of UCB cells in 21-day cultures express ALB, and ALB+ cells co express hepatocyte lineage markers. ALB-expressing cells are able to proliferate in the culture system. In the cell transplantation model of liver-injured SCID mice, inoculated UCB cells develop into functional hepatocytes in the liver, which release human ALB into the sera of the recipient mice. Thus, human UCB is a source of transplantable hepatic progenitor cells (38).
It was reported that human UCB cells can migrate into NOD-SCID liver and become mature hepatocytes. No cell fusion can be detected in any of the human cells found in mouse liver. UCB contains an array of different immune cells, some of which probably play a role in the lowered levels of GvHD manifest byUCB, compared with BM transplantation, and some of which may influence the survival and engrafting capability of CB HSC (10). One of the advantages of UCB is the immaturity of the immune system at birth. This should decrease the alloreactive potential of the lymphocytes within a cord blood graft, and thereby reduce the incidence and severity of GVHD after HLA-matched or mismatched transplants (39, 40). The spleen has unique anatomic affinity with the liver, when the diseased liver is not suitable for stem cells transplantation, intrasplenic transplantation may retain the cells in the spleen and implement the functions in this study, we injected nucleated cells derived from UCB into the spleen of immunocompetent rats after induction of cirrhosis without immunosupression and the results were astonishing, that examined liver sections didn’t show significant inflammatory aggregates indicating graft rejection and no mortalities were detected between transplanted animals. Histopathologic examination showed decreased fibrosis with liver regeneration and better restoration of architecture. Liver sections of transplanted animals were stained positive for antihuman hepatocyte antibodies, which stains only human hepatocytes, while none of the control animals showed such staining. Sections of spleens of the transplanted animals showed no human hepatocytes by immunostaining indicating that UCBSCs had migrated to the liver and engrafted, multiplied and differentiated into human hepatocytes which deny the concept of hepatization of the spleen which this study was trying to explore and these results are in agreement with those obtained by many authors (20–22, 40).
References
1. Allen KJ, Soriano HE. Liver cell transplantation: the road to clinical application. J Lab Clin Med. 2001. 138:298–312.


2. Petersen BE, Bowen WC, Patrene KD, Mars WM, Sullivan AK, Murase N, Boggs SS, Greenberger JS, Goff JP. Bone marrow as a potential source of hepatic oval cells. Science. 1999. 284:1168–1170.


3. Lagasse E, Connors H, Al-Dhalimy M, Reitsma M, Dohse M, Osborne L, Wang X, Finegold M, Weissman IL, Grompe M. Purified hematopoietic stem cells can differentiate into hepatocytes in vivo. Nat Med. 2000. 6:1229–1234.


4. Avital I, Inderbitzin D, Aoki T, Tyan DB, Cohen AH, Ferraresso C, Rozga J, Arnaout WS, Demetriou AA. Isolation, characterization, and transplantation of bone marrow-derived hepatocyte stem cells. Biochem Biophys Res Commun. 2001. 288:156–164.


5. Schwartz RE, Reyes M, Koodie L, Jiang Y, Blackstad M, Lund T, Lenvik T, Johnson S, Hu WS, Verfaillie CM. Multipotent adult progenitor cells from bone marrow differentiate into functional hepatocyte-like cells. J Clin Invest. 2002. 109:1291–1302.


6. Wang PP, Wang JH, Yan ZP, Hu MY, Lau GK, Fan ST, Luk JM. Expression of hepatocyte-like phenotypes in bone marrow stromal cells after HGF induction. Biochem Biophys Res Commun. 2004. 320:712–716.


7. Lee KD, Kuo TK, Whang-Peng J, Chung YF, Lin CT, Chou SH, Chen JR, Chen YP, Lee OK. In vitro hepatic differentiation of human mesenchymal stem cells. Hepatology. 2004. 40:1275–1284.


8. Yamamoto N, Terai S, Ohata S, Watanabe T, Omori K, Shinoda K, Miyamoto K, Katada T, Sakaida I, Nishina H, Okita K. A subpopulation of bone marrow cells depleted by a novel antibody, anti-Liv8, is useful for cell therapy to repair damaged liver. Biochem Biophys Res Commun. 2004. 313:1110–1118.


9. Sato Y, Araki H, Kato J, Nakamura K, Kawano Y, Kobune M, Sato T, Miyanishi K, Takayama T, Takahashi M, Takimoto R, Iyama S, Matsunaga T, Ohtani S, Matsuura A, Hamada H, Niitsu Y. Human mesenchymal stem cells xenografted directly to rat liver are differentiated into human hepatocytes without fusion. Blood. 2005. 106:756–763.


10. Gluckman E, Rocha V, Chevret S. Results of unrelated umbilical cord blood hematopoietic stem cell transplant. Transfus Clin Biol. 2001. 8:146–154.


11. Laughlin MJ. Umbilical cord blood for allogeneic transplantation in children and adults. Bone Marrow Transplant. 2001. 27:1–6.


12. Barbash IM, Chouraqui P, Baron J, Feinberg MS, Etzion S, Tessone A, Miller L, Guetta E, Zipori D, Kedes LH, Kloner RA, Leor J. Systemic delivery of bone marrow-derived mesenchymal stem cells to the infarcted myocardium: feasibility, cell migration, and body distribution. Circulation. 2003. 108:863–868.


13. Gao J, Dennis JE, Muzic RF, Lundberg M, Caplan AI. The dynamic in vivo distribution of bone marrow-derived mesenchymal stem cells after infusion. Cells Tissues Organs. 2001. 169:12–20.


14. Liu ZC, Chang TM. Coencapsulation of hepatocytes and bone marrow stem cells: in vitro conversion of ammonia and in vivo lowering of bilirubin in hyperbilirubemia Gunn rats. Int J Artif Organs. 2003. 26:491–497.


15. Liu ZC, Chang TM. Transplantation of bioencapsulated bone marrow stem cells improves hepatic regeneration and survival of 90% hepatectomized rats: a preliminary report. Artif Cells Blood Substit Immobil Biotechnol. 2005. 33:405–410.


16. Sato Y, Araki H, Kato J, Nakamura K, Kawano Y, Kobune M, Sato T, Miyanishi K, Takayama T, Takahashi M, Takimoto R, Iyama S, Matsunaga T, Ohtani S, Matsuura A, Hamada H, Niitsu Y. Human mesenchymal stem cells xenografted directly to rat liver are differentiated into human hepatocytes without fusion. Blood. 2005. 106:756–763.


17. Hua L, Aoki T, Jin Z, Nishino N, Yasuda D, Izumida Y, Morohara K, Koizumi T, Shimizu Y, Murai N, Kusano M. Elevation of serum albumin levels in nagase analbuminemic rats by allogeneic bone marrow cell transplantation. Eur Surg Res. 2005. 37:111–114.


18. Avital I, Inderbitzin D, Aoki T, Tyan DB, Cohen AH, Ferraresso C, Rozga J, Arnaout WS, Demetriou AA. Isolation, characterization, and transplantation of bone marrow-derived hepatocyte stem cells. Biochem Biophys Res Commun. 2001. 288:156–164.


19. am Esch JS 2nd, Knoefel WT, Klein M, Ghodsizad A, Fuerst G, Poll LW, Piechaczek C, Burchardt ER, Feifel N, Stoldt V, Stockschläder M, Stoecklein N, Tustas RY, Eisenberger CF, Peiper M, Häussinger D, Hosch SB. Portal application of autologous CD133+ bone marrow cells to the liver: a novel concept to support hepatic regeneration. Stem Cells. 2005. 23:463–470.


20. Okumoto K, Saito T, Hattori E, Ito JI, Suzuki A, Misawa K, Sanjyo M, Takeda T, Sugahara K, Saito K, Togashi H, Kawata S. Expression of Notch signalling markers in bone marrow cells that differentiate into a liver cell lineage in a rat transplant model. Hepatol Res. 2005. 31:7–12.


21. Vogels BA, Maas MA, Bosma A, Chamuleau RA. Significant improvement of survival by intrasplenic hepatocyte transplantation in totally hepatectomized rats. Cell Transplant. 1996. 5:369–378.


22. Kobayashi N, Miyazaki M, Fukaya K, Noguchi H, Tanaka N, Namba M. Intrasplenic transplantation of immortalized human fetal hepatocytes prolongs the survival of 90% hepatectomized rats. Transplant Proc. 2000. 32:2365–2367.


23. Kim WH, Lee JH, Han SU, Wang HJ, Kim MW. Can clamping of splenic vessels prevent abrupt increase of portal vein pressure and migration of transplanted hepatocytes to the liver after intrasplenic hepatocyte transplantation? Hepatogastroenterology. 1998. 45:2425–2429.
24. Ryu KH. Liver stem cells derived from the bone marrow and umbilical cord blood. Int J Stem Cell. 2009. 2:97–101.


25. Sell S. Stem cells handbook. Totowa, NJ: Humana Press;2004.
26. Reubinoff BE, Pera MF, Fong CY, Trounson A, Bongso A. Embryonic stem cell lines from human blastocysts: somatic differentiation in vitro. Nat Biotechnol. 2000. 18:399–404.


27. Thomas ED. Bone marrow transplantation from the personal viewpoint. Int J Hematol. 2005. 81:89–93.


28. Bianco P, Riminucci M, Gronthos S, Robey PG. Bone marrow stromal stem cells: nature, biology, and potential applications. Stem Cells. 2001. 19:180–192.


29. Herzog EL, Chai L, Krause DS. Plasticity of marrow-derived stem cells. Blood. 2003. 102:3483–3493.


30. Orlic D, Kajstura J, Chimenti S, Jakoniuk I, Anderson SM, Li B, Pickel J, McKay R, Nadal-Ginard B, Bodine DM, Leri A, Anversa P. Bone marrow cells regenerate infarcted myocardium. Nature. 2001. 410:701–705.


31. Hori N, Okanoue T, Sawa Y, Mori T, Kashima K. Hemodynamic characterization in experimental liver cirrhosis induced by thioacetamide administration. Dig Dis Sci. 1993. 38:2195–2202.


34. Muraca M, Neri D, Parenti A, Feltracco P, Granato A, Vilei MT, Ferraresso C, Ballarin R, Zanusso GE, Giron G, Rozga J, Gerunda G. Intraportal hepatocyte transplantation in the pig: hemodynamic and histopathological study. Transplantation. 2002. 73:890–896.


35. Stéphenne X, Najimi M, Sibille C, Nassogne MC, Smets F, Sokal EM. Sustained engraftment and tissue enzyme activity after liver cell transplantation for argininosuccinate lyase deficiency. Gastroenterology. 2006. 130:1317–1323.


36. Tang XP, Zhang M, Yang X, Chen LM, Zeng Y. Differentiation of human umbilical cord blood stem cells into hepatocytes in vivo and in vitro. World J Gastroenterol. 2006. 12:4014–4019.


37. Tanabe Y, Tajima F, Nakamura Y, Shibasaki E, Wakejima M, Shimomura T, Murai R, Murawaki Y, Hashiguchi K, Kanbe T, Saeki T, Ichiba M, Yoshida Y, Mitsunari M, Yoshida S, Miake J, Yamamoto Y, Nagata N, Harada T, Kurimasa A, Hisatome I, Terakawa N, Murawaki Y, Shiota G. Analyses to clarify rich fractions in hepatic progenitor cells from human umbilical cord blood and cell fusion. Biochem Biophys Res Commun. 2004. 324:711–718.
38. Kakinuma S, Tanaka Y, Chinzei R, Watanabe M, Shimizu-Saito K, Hara Y, Teramoto K, Arii S, Sato C, Takase K, Yasumizu T, Teraoka H. Human umbilical cord blood as a source of transplantable hepatic progenitor cells. Stem Cells. 2003. 21:217–227.


39. Rocha V, Wagner JE Jr, Sobocinski KA, Klein JP, Zhang MJ, Horowitz MM, Gluckman E. Graft-versus-host disease in children who have received a cord-blood or bone marrow transplant from an HLA-identical sibling. Eurocord and International Bone Marrow Transplant Registry Working Committee on Alternative Donor and Stem Cell Sources. N Engl J Med. 2000. 342:1846–1854.


Fig. 1.
(A) cirrhotic nodules surrounded by thick fibrous tissue septa (Masson trichrome ×200). (B) Thin resolved septa and regeneration of hepatocytes 8 weeks post-transplant (HE stain ×100).
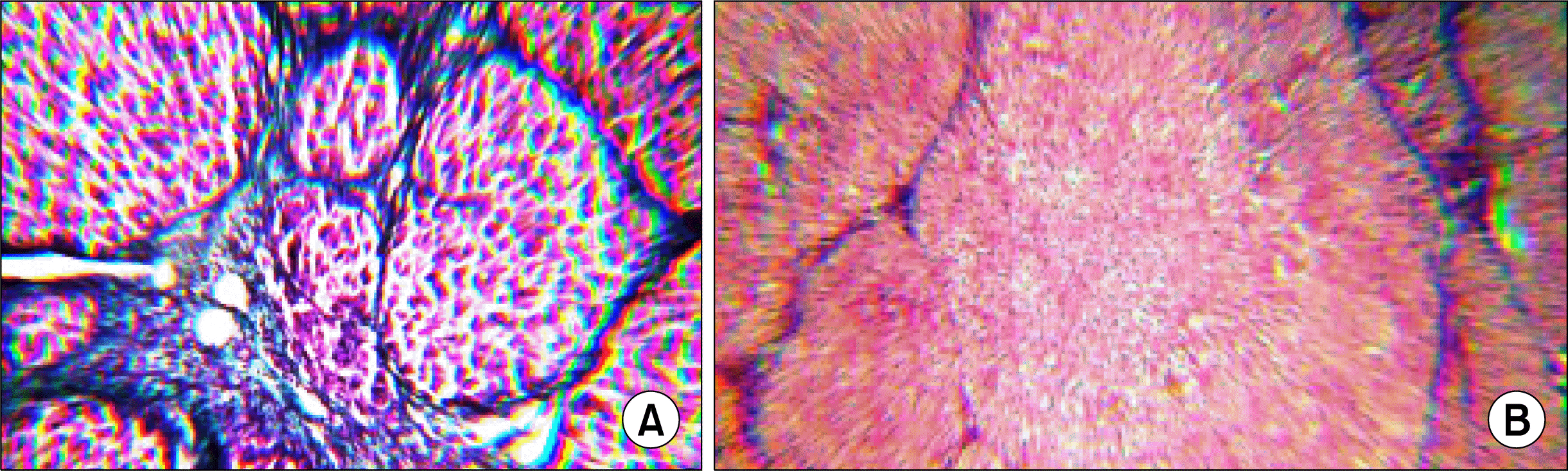
Fig. 2.
(A–C) Liver sections from recipient animals showing positive staining cells for human hepatocytes ABC technique X-200. (D) Liver section of control group (injected with PBS instead of cells) showing negative immunostaining. (E, F) Sections of spleen of the recipient animals showing negative immunostaining (H&E and ABC complex X-100).
