Abstract
Background and Objectives:
In the last few years, treatment protocols using mesenchymal stem cells (MSC) in various experimental models and human diseases have been investigated. MSCs are on the focus of stem cell research, since they are considered as a type of adult stem cells with low toxicity and acceptable side effects profile and they can be administered autologously. In addition several studies have revealed significant immunomodulatory properties of MSCs and a potential for transdifferentiation, including neural differentiation, both in vivo and in vitro. Magnetic resonance imaging (MRI) is a non-invasive technique that can be used to track labeled cells and evaluate their migration ability in various clinical settings.
Methods and Results:
In this study we investigated whether such labeling of MSCs with the commercially used para-magnetic material, Feridex, has any negative effect on the above mentioned functional properties of MSCs. We labeled human mesenchymal stem cells (hMSC) with poly-L-lysine coated Feridex® and evaluated their cellular differentiation and immunomodulatory properties, in vitro. In comparison with unlabeled cells, labeled hMSC exhibited normal adipogenic and osteogenic differentiation, but decreased chondrogenic differentiation. Regarding neural differentiation, labeled and unlabeled cells were similar in their ability to express neural-like and glial like surface proteins. Finally, both labeled and unlabeled MSCs exhibited a dose-dependent, significant blocking effect on the proliferation of healthy donors lymphocytes following mitogen stimulation.
Conclusions:
These findings indicate that labeling with Feridex does not affect the immunomodulatory, nor the neural transdifferentiation potential of MScs and therefore, Feridex may be used for the tracking of this type of stem cells in clinical applications, without compromising their major functional properties.
Mesenchymal stem cells (MSC) represent the second major stem cells population in the bone marrow reservoire. These cells are described as non-hematopoietic stromal cells and their classic role is to support the process of hematopoiesis and hematopoietic stem cells (HSC) engraftement and to give rise to cells of the mesodermal lineage such as, osteoblasts, adipocytes and chondrocytes (1). MSCs do not have a specific surface marker profile, but it is widely accepted that they are negative for CD34, CD45 and CD14 and positive for CD29, CD73, CD90, CD105 and CD166. Several in vitro and in vivo (in animal models) studies have shown that MSCs can differentiate into neural like and glial-like cells (2–4), carrying therefore the potential for neuroregeneration. MSCs were additionally found to possess significant immunomodulating properties (5).
Animal studies have indicated a therapeutic potential of MSCs in a variety of disease such as: stroke, multiple sclerosis, myocardial infraction and GVHD (2, 6, 7). MSCs were shown to engraft and migrate into the injured/inflamed organs or sites and to exert immunomodulatory and neurotrophic/neuroprotective effects (2, 8–11). Since the first and main question relevant for clinical applications of MSCs is whether the transplanted cells will reach at significant numbers the affected organs/sites, it seems essential to explore non-invasive and sensitive methods to track the implanted cells. One of these methods is by using MR imaging-based techniques. Indeed, lately, magnetic labeling with supermagnetic iron oxide particles (SPIO) (which makes cells visible in the MRI), of different stem cells types, including MSCs, has been applied (12–15). One of the FDA-approved SPIOs is Feridex© which incorporates into the cells cytoplasma (16). Feridex labeled MSCs were successfully used in clinical studies. However, it is still unknown whether this labeling with Feridex, may compromise in any way the revenant biological properties of MSC’s.
In this study we evaluated whether labeling of hMSC with the SPIO, Feridex, affects their main functional properties. For this we used MSCs prepared from MS patients and tested in vitro their morphology, surface markers profile and their immunomodulatory and neuronal differentiation abilities, following staining with Feridex, as compared to unlabeled hMSC.
Aspirated bone marrow inoculum from multiple sclerosis patients, participating in our phase I/II clinical trial (NIH registration number: NCT00781872), was proceeded according to classical methods for mesenchymal stem cells isolation (17). Briefly, a purified mesenchymal stem cells culture was prepared using plastic adherence method in filtered sterilized low glucose-DMEM medium supplemented with 10% of Foetal Bovine Serum (FBS), 1% L-Glutamine and 1% L-Pen-Strep-Nystatin (PSN) solution (all from Biological Industries, Israel).
hMSC cultures were incubated for 48 hours prior to use with 0.25 l/ml Feridex© (11.3 mg/ml, Berlex, USA), and 375 ng/ml of the cationic polymer poly-L-lysine (PLL, Sigma, Israel) in low-glucose DMEM containing 10% FBS, 1% L-Glutamine and 1% PSN.
Flow cytometry: hMSC with and without Feridex were harvested with Trypsin/EDTA Solution (Biological Industries, Israel), divided into polystyrene fluorescence activated cell sorter (FACS) tubes, and labeled with anti human CD45-FITC, anti human CD90-PE and anti human CD105-PE (Beckman Coulter, Israel) for 45 minutes in the dark. Fluorescence data were collected from 30,000 cells and measurements and analysis were performed using a Beckman Coulter FACS machine.
Immunoflurecense: hMSC with and without PLL-coated Feridex were cultured on non-treated coverslips (NUNC Inc., USA) for 48 hours, washed (×3) with PBS and stained with labeled anti human CD45-FITC (Beckman Coulter, Israel), anti human CD90-Texas Red and anti human CD105-Texax Red (Santa Cruz Biotechnology, USA). Fluorescence was evaluated under florescence microscope.
To induce hMSC to differentiate into various cell phenotypes, 2×105 cells were plated in culture dishes and allowed to reach confluence. Osteogenic differentiation medium, consisting of 10% Eagle minimum essential medium/alpha medium and fetal bovine serum, supplemented with 50-mg/mL ascorbic acid, 10 mM β-glycerol-phosphate, and 108Mdexamethasone (all from Sigma, Israel), was exchanged twice a week for 3 weeks. For adipocytic differentiation, commercially adipogenic differentiation kit was used according to the manufacture instructions (STEMCELL Technologoies, Canada). Chondrogenic differentiation was induced by culturing the cells with media containing MEM-alpha, 5% FBS, 1% L-Glutamine, 1% non-essential amino acids, 1% non-essential vitamins, 1% PSN (all from Biological Industries, Israel) and 10 ng/ml TGF-β3 (Sigma, Israel) for 14 days with medium exchange twice a week.
For neural-glial differentiation, hMSC were seeded on fibronectin coated (1 μg/ml, Sigma, Israel) 24-well plates and cultured in Eagle minimum essential medium/alpha medium enriched with 7% FBS, 1% glutamine, 1% vitamins, 1% PSN solution, 20 ng/mL brain-derived neurotrophic factor (Peprotech, Israel), 10 ng/mL fibroblast growth factor-2 (Peprotech, Israel), 20 ng/mL fibroblast growth factor 8 (Peprotech, Israel), 10 ng/ml nerve growth factor (Peprotech, Israel) and 10 ng/ml nurotrophin-4 (Peprotech, Israel). The cells were cultured under these conditions for 18 day. Then the culture media were changed while being depleted of FBS and cultured for more 5∼7 days.
Freshly prepared Perls’ reagent by dissolving 1 gram potassium cyanide in 42ml deionized water+8 ml of 37.5% HCl. For nuclear fast red (NFR) counterstain, 0.1 gram of NFR was dissolved in 100 ml deionized water+5 gram of aluminium sulfate (all from Sigma, Israel). Cells for staining were washed (×3) with PBS and incubated 30 minutes in the dark, washed again (×3) with PBS and incubated with NFR counterstain for 5 minutes, They were then rinsed (×3) in deionized water, dried overnight in dark and embedded in Permount with coverslip.
To detect osteoblastic differentiation, NBT/BCIP staining which shows the alkaline phosphatase activity was used. NBT/BCIP solution (Sigma, Israel) was added to the cells for 30 min at 37°C in a 5% CO2 incubator. After incubation, cells were washed with PBS (×3) and fixed with 4% PFA. To detect adipogenic differentiation, 10 mg/mL oil red-O (Sigma, Israel) was added and left for 20 minutes at room temperature. The cells were washed 3 times with PBS and fixed with PFA 4%, for 20 minutes. For the detection of chondrogenic differentiation, alcian blue staining was used. The cells were then washed with PBS and fixated with 4%. Then, the cells were rinsed with alcian blue solution, pH 2.5 (Sigma, Israel) for 30 min at RT and washed in running tap water for 2 min and in distilled water for another 2 min. Extracellular matrix components secreted by chondrocytes were then stained blue.
The medium was aspirated and the cells were washed gently with 0.05% Tween 20 diluted in PBS and then fixed with 4% fresh PFA (Sigma, Israel) for 20 minutes at room temperature. To stain the intracellular components, the cells were permeabilized with 0.1% Triton X-100 (Sigma, Israel) for 10 minutes. For blocking nonspecific binding, the cells were rinsed with 5% bovine serum albumin in PBS for 60 minutes at room temperature on a slowly rotated plate. Then, they were washed 3 times with 0.05% Tween 20 (Sigma, Israel) diluted in PBS, and incubated with the following primary antibodies: anti human myelin associated protein 2 (MAP-2), anti human β-tubulin type III, anti human glial fibrillary acidic protein (GFAP), and anti human Galactocerebrosidase (GalC), (all from Chemicon, USA) diluted to the required concentrations with buffer containing 1% bovine serum albumin (Sigma, Israel). After washing, the cells were incubated with goat anti mouse fluorescein isothiocyanate-conjugated and goat anti rabbit tetramethylrhodamine isothiocyanate-conjugated secondary antibodies (Jackson Immunoresearch, USA) diluted in bovine serum albumin buffer, 1%, on a slowly rotated plate for 45 minutes in the dark at room temperature. The cells were mounted on slides with mounting solution and examined under fluorescence microscope. Some of the slides stained for GalC were mounted with mounting media containing DAPI that stains nuclear DNA in blue.
Lymphocytes were isolated from healthy blood donors using standard Ficol separation gradient. All cultures were carried out in triplicates in 96-well, flat-bottom, microtiter plates. The assay was carried out by seeding 2×105 cells/well in 0.2 ml of RPMI medium supplemented with 5% fetal calf serum, 1% L-glutamine, and 1% PSN (all from biological industries, Israel). To the lymphocytes, 10×103 and 30×103 hMSC (with and without Feridex) were added. 3H-thymidine (Amersham, UK) incorporation was determined in response to PHA (4 μg/ml, Sigma, Israel). The cultures were incubated for 48 hours in a humidified atmosphere of 5.0% carbon dioxide at 37°C and then pulsed for 16 hours with 3H-thymidine (1 μCi/well). Cells were harvested on fiberglass filters (Whatman, USA) using a multiharvester and the radioactivity was counted.
hMSCs (Fig. 1A), could be effectively labeled by Feridex and showed in vitro positive staining with Prussian blue (Fig. 1B). Naïve hMSC and Feridex-labeled hMSCs (Fig. 2A) were similarly stained for the markers CD45, CD90 and CD105. Both were shown to express the typical hMSC markers profile including negativity for CD45 and positive staining for CD90 and CD105 (Fig. 2A). Immuno-histochemical analysis showed negative staining for CD45 and positive staining for CD90 and CD105 with preservation of the typical for hMSC, fibroblast-like shape (Fig. 3).
To prove their mesodermal nature, we cultured hMSC in adipogenic and osteogenic media. When induced to differentiate into osteoblasts (using medium containing osetegenic differentiation factors: see methods), both unlabeled and labeled cells exhibited alkaline phosphatase activity as visualized by NBT-BCIP staining (Fig. 4A, B). Adipogenesis was evident morphologically by their transformation into rounded cells containing lipid vesicles, visualized by oil red O. Both naïve and labeled cells showed adipogenic differentiation and formation of lipid vacuoles (Fig. 4C, D). Chondrogenic differentiation was tested by using alcian blue staining. After classical culture with chondrogenic media (including TGF-beta-3), only the naïve and not the Feridex-labeled hMSC were stained blue, indicative of chondrogenic differentiation (Fig. 4E, F).
Naïve and Feridex-labeled hMSC were cultured on fibronectin-coated slides with neural differentiation media for 3 weeks. The differentiation media was changed twice a week while changing the differentiation factors’ coktail weekly under a controlled and specified protocol (as detailed in Materials and Methods). After 3 weeks the cells were stained for the neural and glial markers, tubulin-beta-III, MAP2, GFAP and GalC (Fig. 5). Both unlabeled and labeled hMSCs were able to differentiate into neural-like cells as demonstrated by positive staining with Tubulin-beta-III (20∼30% of cells) and MAP2 (30∼50% of the cells) (Fig. 5). Astrocytic-like cells was identified by the positive GFAP staining (30∼50% cells) (Fig. 5). Similar staining, at low percentages, was found in both unlabeled and labeled hMSCs, for the oligodendrocytic cells marker GalC (1∼5% of the cells), DAPI staining for the DNA was used in (Fig. 5H) in order to clarify the differentiated cell morphology.
The well known ability of hMSCs to suppress lymphocytes proliferation was tested in a co-culture system. Both naïve and Feridex-labeled hMSC were cultured with peripheral blood lymphocytes obtained from a healthy donor. Three experimental controls were used: (1) lymphocytes were cultured with medium without PHA (presented in Fig. 6 as LYM+MED) (2) lymphocytes that were cultured with medium containing PHA (presented in Figure 6 as LYM+PHA) and (3) Lymphocytes cultured in the presence of mesenchymal stem cells in the absence of stimulation mitogen. Lymphocytes cultured without mitogen did not show any proliferation. In the positive control, lymphocytes cultured with media containing mitogen show high proliferative ability. Lymphocytes cultured with hMSC in the absence of mitogen did not show any proliferation. Two doses of hMSC were used: 10×103 cells and 30×103 cells, mixed with 20×104 lymphocytes under mitogen (PHA) induction. It was found that both types of cells were able to suppress the proliferation of lymphocytes in a similar dose dependent manner (Fig. 6). There was a minor and not statistically significant difference, in favor of the naïve hMSC, in terms of their proliferation blocking effect.
In this study we have shown that labeling mesenchymal stem cells with supermagnetic iron particles (PLL-coated Feridex) does not alter their surface markers profile and typical morphological features. Following Feridex-labeling, their osteogenic and adipogenic differentiation potential was preserved whereas the chondrogenic differentiation was compromised. Most importantly, the neural differentiation ability of hMSC and their immunomodulation properties were not significantly affected.
Based on their potential to behave as stem cells (self-renewal, multipotency and transdifferentiation), the fact that they can be isolated from every adult and administered autologously (a patient can serve as donor for him/her self) and their low toxicity and acceptable adverse events profile, hMSCs have been lately on the focus of the research in the field of regenerative medicine. Their newly demonstrated immunomodulatory effects have additionally contributed to this. hMSCs have been therefore, suggested as a possible future therapeutic intervention in, neurodegenerative and immune-related diseases, and several clinical trials in such indications, are ongoing (www.clinicaltrials.gov).
A largely debatable matter, which is crucially important for the clinical applications of hMSC and stem cells in general, is to define the most suitable way of administration in patients and to investigate whether by the various routes suggested, the transplanted cells can reach (at significant proportions) the affected organs or sites. In order to investigate this migration potential of the hMSC in neurological conditions, labeling and tracking of the cells with non-invasive means, seems of critical importance. To this direction, MR techniques have been tried following the labeling of the cells with supermagnetic particles, which make them visible in the MRI. For intracellular magnetic labeling, the FDA-approved Feridex coated with poly-L-lysine (PLL) has been used in various experimental and clinical settings. The PLL coats the Feridex® and binds to the cells membrane, followed by endocytosis of the magnetic particles.
Studies in animal models of stroke and spinal cord injury (18, 19). utilized the magnetic labeling of the transplanted MSC with Feridex, in order to track them in vivo. In the study by Jendelova et al. (18), MSC were labeled with SPIO and grafted into rats with a cortical photo-chemical lesion MSCs were either grafted intracerebrally into the contralateral hemisphere or injected intravenously (20). The implanted cells were visible on MR images as an hypointense area at the injection site and in the lesion. The hypointense signal persisted for more than 50 days. Prussian blue staining and transmission electron microscopy (TEM) confirmed the presence of iron-oxide nanoparticles inside the cells. In a rat model of spinal cord injury (19), SPIO-labeled MSCs, injected i.v. were visualized by MRI as non-homogeneity in the tissue texture with a hyperintenese signal at the area of the injury.
Labeling with SPIO has been tried with similar efficacy in different stem cells types such as embroyinc stem cells (14, 18) and neural stem/precursor cells (21). In a model of experimental autoimmune encephalomyelitis (EAE) (21). intracerebroventricularly (ICV) transplantated SPIO-labeled neural precursor cells (NPC) responded to inflammatory cues, migrated in white matter tracts and differentiated into the glial lineages, in a similar fashion as unlabeled cells. SPIO-labeling did not affect the survival and pluripotency of NPCs, in vitro. Furthermore, labeled NPCs inhibited lymph node cell proliferation in vitro, similarly to non-labeled cells, suggesting a preserved immunomodulatory function.
Following the evidence of successful tracking of the transplanted cells with Feridex, the question that is logically raised is whether this labeling compromises the functional properties of MSCs. Few previous studies were conducted in order to study the effects of SPIO-labeling on functional properties of hMSC. In the study by Kostura et. al, hMSC were labeled with poly-L-lysine (PLL)-coated Feridex and with bare Feridex (16). As compared with unlabeled controls, labeled hMSC exhibited an unaltered viability, proliferated similarly, and underwent normal adipogenic and osteogenic differentiation. However, there was a marked inhibition of chondrogenesis. The blocking of chondrogenic activity was mediated by the Feridex, rather than by the transfection agent (PLL).
In the study of Arbab et al. (12), the effect of ferum-oxides-poly-l-lysine (PLL) complex for magnetic cell labeling on the long-term viability, function, metabolism, and iron utilization of mammalian cells, was evaluated. It was found that intracytoplasmic nanoparticles were stained with Prussian blue when the ferumoxides-PLL complex following magnetically labeling of the human mesenchymal stem and HeLa cells. The long-term viability, growth rate, and apoptotic indexes of the labeled cells were unaffected by the endosomal incorporation of SPIO, as compared with the nonlabeled cells. In nondividing human mesenchymal stem cells, endosomal iron nanoparticles could be detected after 7 weeks; however, in rapidly dividing cells, intra-cellular iron had disappeared by five to eight divisions. A nonsignificant transient increase in reactive oxygen species production was observed in the human mesenchymal stem and HeLa cell lines, following labeling.
hMSC can be also isolated from other than bone marrow, tissues such as cord blood, adipose tissue and peripheral blood (22–24). hMSC obtained from umbilical cord blood could be labeled with SPIO with high efficiency without significant change in viability and apoptosis (25).
In our experiments, Feridex incorporation into bone marrow hMSC was very high (90∼100 %), within 48 hrs of incubation. Feridex-labeling did not affect the proliferation and expansion ability of the hMSC in culture (data not shown). The labeled cells were found to preserve their fibroblast-like spindle shape morphology. Both labeled and unlabeled hMSC exhibited a similar surface markers profile in cytometric analysis (negativity for the hematopoietic marker, CD45 and positivity for the mesenchymal markers CD90 and CD105). The difference observed, between the classical mesodermal differentiation, (which was not affected by labeling) and the compromised ability for chondrogenic differentiation is not novel and has been earlier shown by others (16), suggesting an interference of the intracellular chondrogenic differentiation cascade, by Feridex. However, other studies showed conflicting results, indicating that chondrogenisis is preserved following labeling by Feridex (12).
The most relevant for clinical uses in neurodegenerative and neuroinflammatory diseases, property of MSC is their neuro-differentiation potential and their immunomodulatory effects. In our study we have demonstrated that at least in vitro, these functions are not compromised. Several studies and our previous works, have shown the neural differentiation ability of MSC both in vitro and in vivo (2–4, 26, 27). This trans-differentiation could be induced and stimulated with different methods, either by chemical induction or by the use of growth factors. Such neural differentiation could be highly relevant for neurological diseases like multiple sclerosis, neurodegenaration and stroke. In animal studies, it was shown by us and others that MSC could induce strong neurotrophic and neuroprotective effects in the murine models of MS (EAE), Parkinson’s disease, spinal trauma and ALS (2, 28–30). In our previous studies, we have shown that murine and human MSCs can differentiate into neural-like and glial-like cells under specific conditioning by growth factors (2). Here, we applied the same conditions to the Feridex® labeled cells. We found, that these cells kept there potential to acquire the neural and glial morphology in vitro.
The immunomodulating properties of hMSC are mostly relevant for immune-mediated diseases such as multiple sclerosis and GVHD. In our study, labeled-hMSC retained to a large extent the ability to suppress lymphocytes proliferation following stimulation with the mitogen PHA, in a dose-dependent manner.
In conclusion, hMSC can be labeled easily with SPIO while retaining their morphological, immunohistological, immunomodulatory and differentiation properties. These data support, that hMSC can be labeled and tracked by MRI without the concern of losing their basic biological properties.
ACKNOWLEDGMENTS
This study was supported by a fellowship grant from the European Committee for Treatment and Research in Multiple Sclerosis (ECTRIMS) and the by the Eshkol grant from the Israeli Ministry of Science.
References
1. Pittenger MF, Mackay AM, Beck SC, Jaiswal RK, Douglas R, Mosca JD, Moorman MA, Simonetti DW, Craig S, Marshak DR. Multilineage potential of adult human mesenchymal stem cells. Science. 1999. 284:143–147.


2. Kassis I, Grigoriadis N, Gowda-Kurkalli B, Mizrachi-Kol R, Ben-Hur T, Slavin S, Abramsky O, Karussis D. Neuroprotection and immunomodulation with mesenchymal stem cells in chronic experimental autoimmune encephalomyelitis. Arch Neurol. 2008. 65:753–761.


3. Sanchez-Ramos J, Song S, Cardozo-Pelaez F, Hazzi C, Stedeford T, Willing A, Freeman TB, Saporta S, Janssen W, Patel N, Cooper DR, Sanberg PR. Adult bone marrow stromal cells differentiate into neural cells in vitro. Exp Neurol. 2000. 164:247–256.


4. Woodbury D, Schwarz EJ, Prockop DJ, Black IB. Adult rat and human bone marrow stromal cells differentiate into neurons. J Neurosci Res. 2000. 61:364–370.


5. Uccelli A, Moretta L, Pistoia V. Immunoregulatory function of mesenchymal stem cells. Eur J Immunol. 2006. 36:2566–2573.


6. Akiyama Y, Radtke C, Kocsis JD. Remyelination of the rat spinal cord by transplantation of identified bone marrow stromal cells. J Neurosci. 2002. 22:6623–6630.


7. Chen J, Li Y, Wang L, Lu M, Zhang X, Chopp M. Therapeutic benefit of intracerebral transplantation of bone marrow stromal cells after cerebral ischemia in rats. J Neurol Sci. 2001. 189:49–57.


8. Bartholomew A, Sturgeon C, Siatskas M, Ferrer K, McIntosh K, Patil S, Hardy W, Devine S, Ucker D, Deans R, Moseley A, Hoffman R. Mesenchymal stem cells suppress lymphocyte proliferation in vitro and prolong skin graft survival in vivo. Exp Hematol. 2002. 30:42–48.


9. van Gelder M, van Bekkum DW. Treatment of relapsing experimental autoimmune encephalomyelitis in rats with allogeneic bone marrow transplantation from a resistant strain. Bone Marrow Transplant. 1995. 16:343–351.
10. Zappia E, Casazza S, Pedemonte E, Benvenuto F, Bonanni I, Gerdoni E, Giunti D, Ceravolo A, Cazzanti F, Frassoni F, Mancardi G, Uccelli A. Mesenchymal stem cells ameliorate experimental autoimmune encephalomyelitis inducing T-cell anergy. Blood. 2005. 106:1755–1761.


11. Arbab AS, Wilson LB, Ashari P, Jordan EK, Lewis BK, Frank JA. A model of lysosomal metabolism of dextran coated superparamagnetic iron oxide (SPIO) nanoparticles: implications for cellular magnetic resonance imaging. NMR Biomed. 2005. 18:383–389.


12. Arbab AS, Wilson LB, Ashari P, Jordan EK, Lewis BK, Frank JA. A model of lysosomal metabolism of dextran coated superparamagnetic iron oxide (SPIO) nanoparticles: implications for cellular magnetic resonance imaging. NMR Biomed. 2005. 18:383–389.


13. Ben-Hur T, Idelson M, Khaner H, Pera M, Reinhartz E, Itzik A, Reubinoff BE. Transplantation of human embryonic stem cell-derived neural progenitors improves behavioral deficit in Parkinsonian rats. Stem Cells. 2004. 22:1246–1255.


14. Syková E, Jendelová P. Magnetic resonance tracking of implanted adult and embryonic stem cells in injured brain and spinal cord. Ann N Y Acad Sci. 2005. 1049:146–160.


15. Tang Z, Lin N, Fang H, Zhu K, Liu Y, Zhong Y, Lin J, Kang Z, Xu R. Retracted Article: MRI tracking of the fate of intravascularly injected and SPIO-Labeled rat mesenchymal stem cells in the livers of rats with hepatic fibrosis. Dig Dis Sci. 2010. 55:2122.


16. Kostura L, Kraitchman DL, Mackay AM, Pittenger MF, Bulte JW. Feridex labeling of mesenchymal stem cells inhibits chondrogenesis but not adipogenesis or osteogenesis. NMR Biomed. 2004. 17:513–517.


17. Lennon DP, Caplan AI. Isolation of human marrow-derived mesenchymal stem cells. Exp Hematol. 2006. 34:1604–1605.


18. Jendelová P, Herynek V, Urdzíková L, Glogarová K, Kroupová J, Andersson B, Bryja V, Burian M, Hájek M, Syková E. Magnetic resonance tracking of transplanted bone marrow and embryonic stem cells labeled by iron oxide nano-particles in rat brain and spinal cord. J Neurosci Res. 2004. 76:232–243.


19. Urdzíková L, Jendelová P, Glogarová K, Burian M, Hájek M, Syková E. Transplantation of bone marrow stem cells as well as mobilization by granulocyte-colony stimulating factor promotes recovery after spinal cord injury in rats. J Neurotrauma. 2006. 23:1379–1391.


20. Jendelová P, Herynek V, DeCroos J, Glogarová K, Andersson B, Hájek M, Syková E. Imaging the fate of implanted bone marrow stromal cells labeled with super-paramagnetic nanoparticles. Magn Reson Med. 2003. 50:767–776.


21. Cohen ME, Muja N, Fainstein N, Bulte JW, Ben-Hur T. Conserved fate and function of ferumoxides-labeled neural precursor cells in vitro and in vivo. J Neurosci Res. 2010. 88:936–944.
22. Bernacki SH, Wall ME, Loboa EG. Isolation of human mesenchymal stem cells from bone and adipose tissue. Methods Cell Biol. 2008. 86:257–278.


23. Laitinen A, Laine J. Isolation of mesenchymal stem cells from human cord blood. Curr Protoc Stem Cell Biol. 2007. Chapter 2::Unit 2A.3.


24. Kassis I, Zangi L, Rivkin R, Levdansky L, Samuel S, Marx G, Gorodetsky R. Isolation of mesenchymal stem cells from G-CSF-mobilized human peripheral blood using fibrin microbeads. Bone Marrow Transplant. 2006. 37:967–976.


25. Ju S, Teng G, Zhang Y, Ma M, Chen F, Ni Y. In vitro labeling and MRI of mesenchymal stem cells from human umbilical cord blood. Magn Reson Imaging. 2006. 24:611–617.


26. Bossolasco P, Cova L, Calzarossa C, Rimoldi SG, Borsotti C, Deliliers GL, Silani V, Soligo D, Polli E. Neuro-glial differentiation of human bone marrow stem cells in vitro. Exp Neurol. 2005. 193:312–325.
27. Dezawa M, Kanno H, Hoshino M, Cho H, Matsumoto N, Itokazu Y, Tajima N, Yamada H, Sawada H, Ishikawa H, Mimura T, Kitada M, Suzuki Y, Ide C. Specific induction of neuronal cells from bone marrow stromal cells and application for autologous transplantation. J Clin Invest. 2004. 113:1701–1710.


28. Kim H, Kim HY, Choi MR, Hwang S, Nam KH, Kim HC, Han JS, Kim KS, Yoon HS, Kim SH. Dose-dependent efficacy of ALS-human mesenchymal stem cells transplantation into cisterna magna in SOD1-G93A ALS mice. Neurosci Lett. 2010. 468:190–194.


Fig. 1.
Labeling of hMSCs with Feridex. (A) naïve hMSC isolated on day 14 after BM seeding, showing the classical fibroblast-like spindle shaped of the cells. (B) Positive Prussian Blue staining of hMSC cultured with Feridex for 48 hrs.
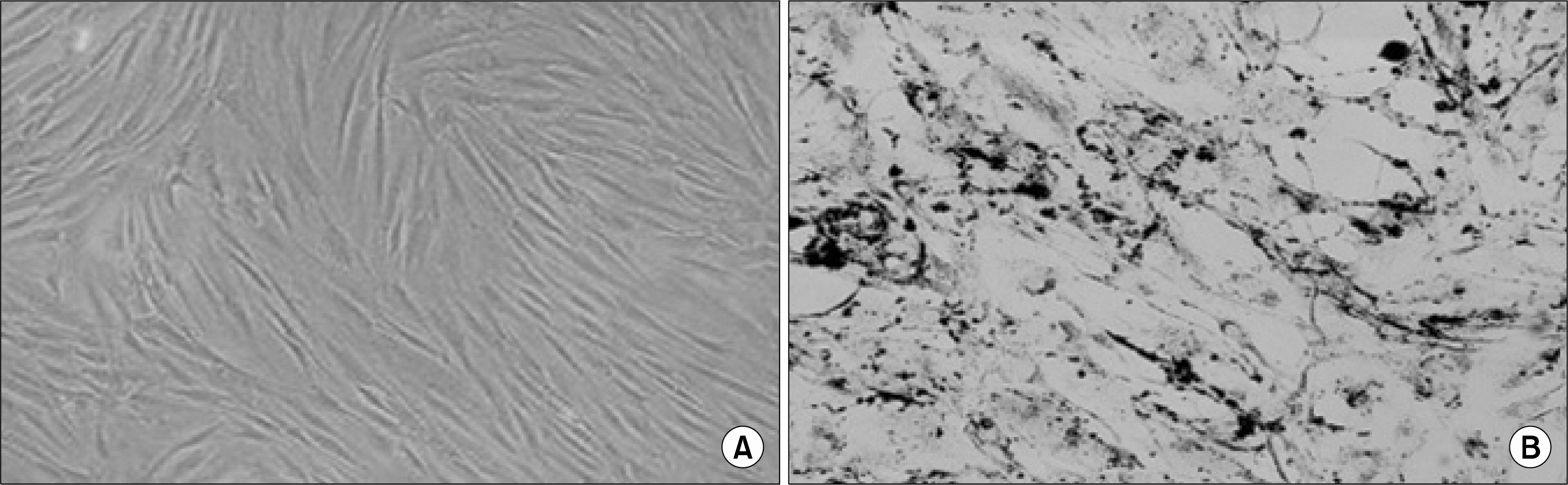
Fig. 2.
Flowcytometry analysis of hMSC. (A) Naïve hMSC isolated on day 14 after BM seeding showing negative staining for the hematopoietic marker CD45 and positive staining for the mesenchymal markers CD90 and CD105. (B) hMSC labeled with Feridex for 48 hrs. showing the same negative staining for the hematopoietic marker CD45 and positive staining for the mesenchymal markers CD90 and CD105.
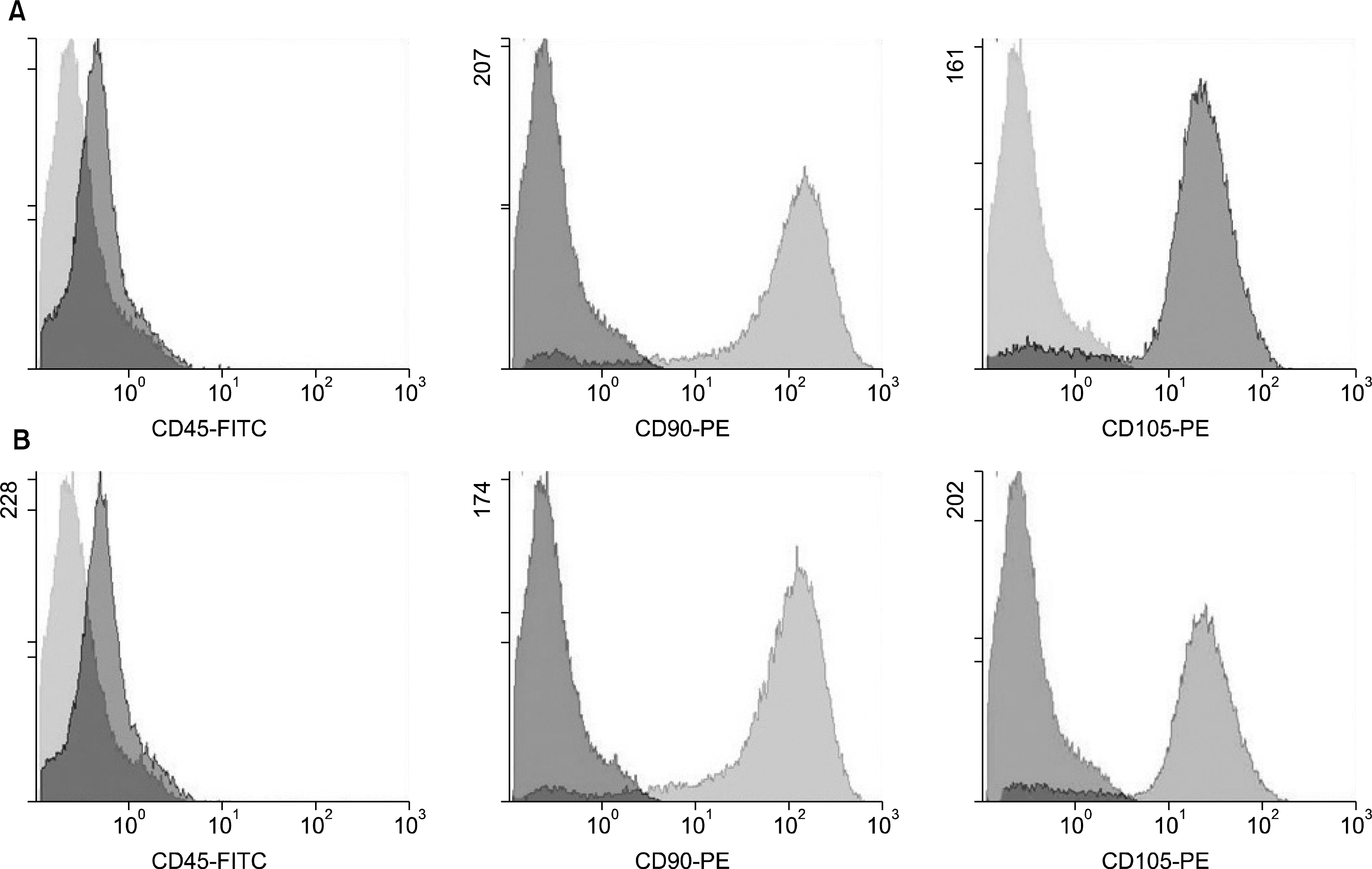
Fig. 3.
Immunostaining analysis of naïve and Feridex-labeled hMSC. Naïve and Feridex-labeled hMSC had the classical spindle-shaped fibroblast-like morphology (A∼F). Both unlabeled and Feridex-labeled cells showed negative staining for the hematopoietic marker CD45 (A, B) and highly positive staining for CD90 (C, D) and CD105 (E, F).

Fig. 4.
Mesodermal differentiation of naïve and Feridex-labeled hMSC. Naïve and labeled hMSC (A, B respectively) differentiated to osteogenic structures as shown by the NBT/BCIP (blue-purple color) staining which detects alkaline phosphatase activity. Oil-red-O staining showed that un-labeled and labeled cells (C, D respectively) formed adipocytic structures. Alcian blue staining that detects chondrogenic matrix formation showed that un-labeled hMSC formed such structures (E), whereas labeled-hMSC were unable to form chondrogenic matrix (F).

Fig. 5.
Neural differentiation of unlabeled and Feridex-labeled hMSC. Naïve and labeled cells exhibited neural-like cell morphology as seen by the positive staining for MAP2 (A, B respectively) and Tubulin-beta-III (C, D) following culture with a coktail of growth factors (as described in Methods). Astrocytic-like morphology was evaluated utilizing GFAP staining that was positive in both un-labeled cells (E) as well for the Feridex-labeled cells (F). Naïve and labeled cells showed also sparse oligodendrocytic differentiation as presented by the staining for GalC (G, H, respectively). DAPI staining was used to stain the nuclear DNA for the oligodendrocytic differentation (H).
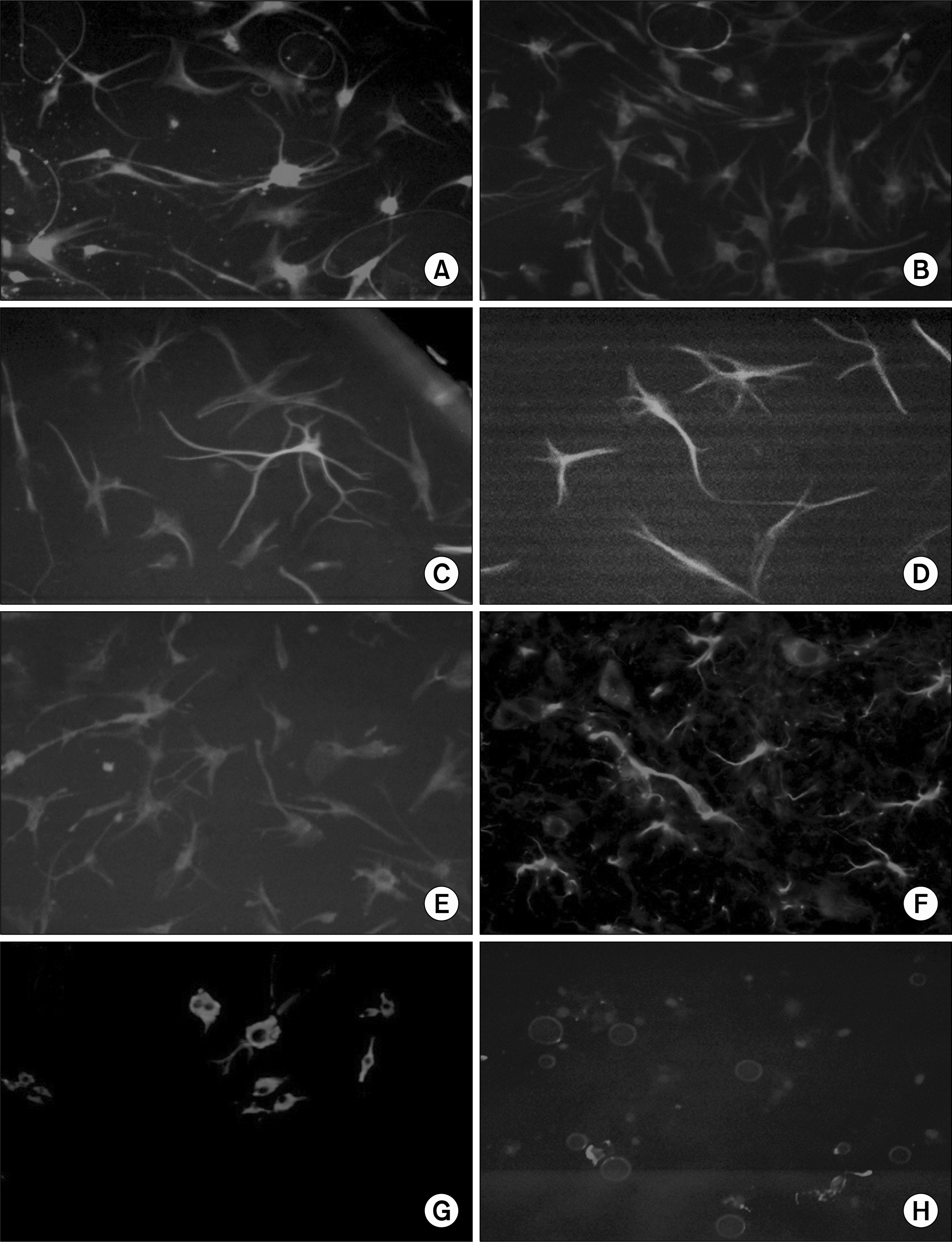
Fig. 6.
Immunomodulatory effects of unlabeled and Feridex-labeled MSCs: Effects on lymphocytic proliferation. The controls of the experiment show that lymphocytes cultured with medium or cultured with hMSC (both without the presence of PHA) did not show any proliferation effects. Lymphocytes cultured in the presence of PHA showed typical lymphocyte proliferation. Naïve and labeled hMSC were added in two doses (10×103 & 30×103) in cultures of naïve human lymphocytes, obtained from donors. Both unlabeled and Feridex-labeled hMSCs equally suppressed the proliferation of lymphocytes induced by PHA mitogen in a dose-dependent manner (MED=Medium, LYM=Lymphocytes).
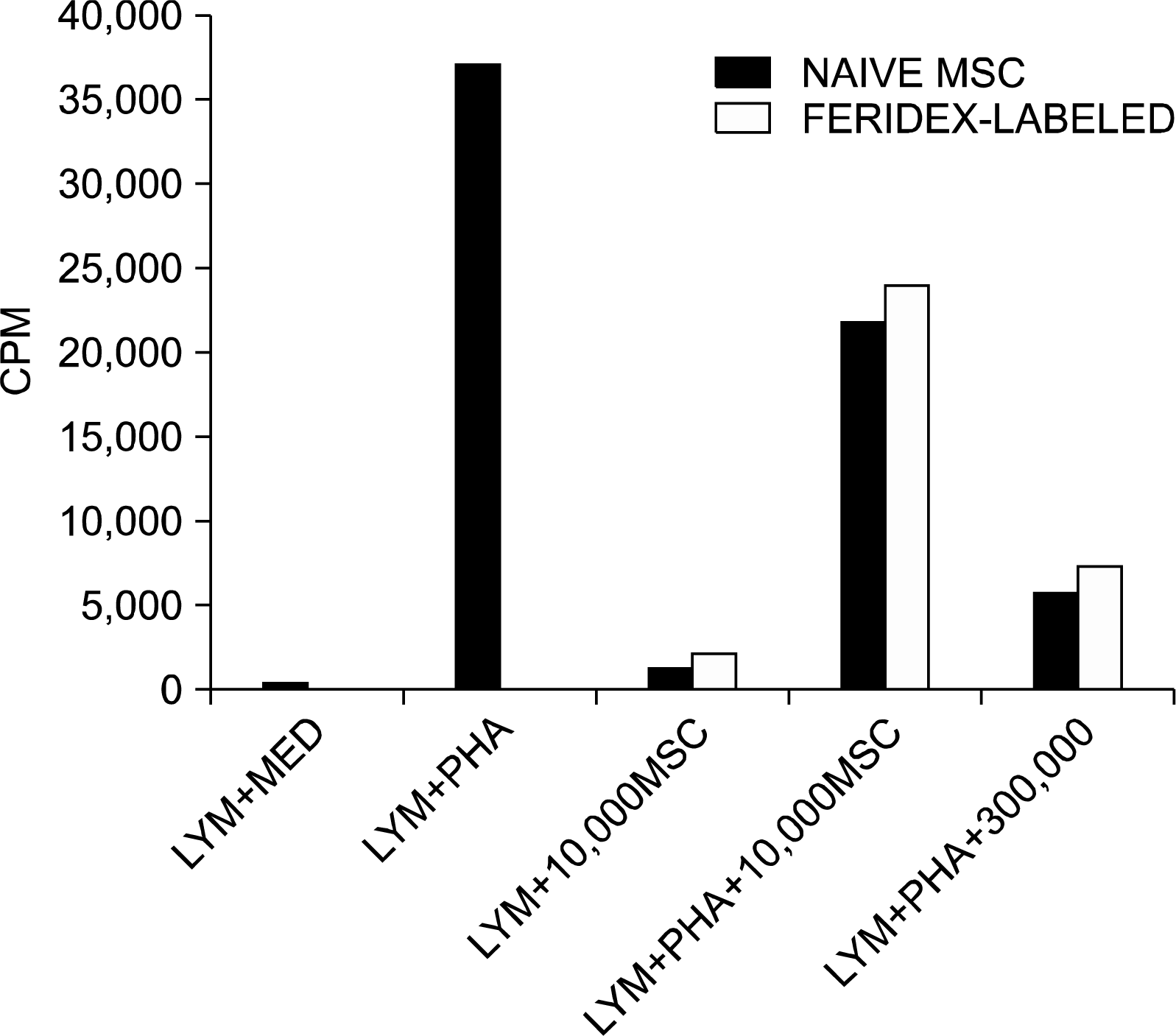