Abstract
The wide use of pesticides for agriculture, domestic and industrial purposes and evaluation of their subsequent effect is of major concern for public health. Human exposure to these contaminants especially bone marrow with its rapidly renewing cell population is one of the most sensitive tissues to these toxic agents represents a risk for the immune system leading to the onset of different pathologies. In this experimental protocol we have developed a mouse model of pesticide(s) induced hypoplastic/aplastic marrow failure to study quantitative changes in the bone marrow hematopoietic stem cell (BMHSC) population through flowcytometric analysis, defects in the stromal microenvironment through short term adherent cell colony (STACC) forming assay and immune functional capacity of the bone marrow derived cells through cell mediated immune (CMI) parameter study. A time course dependent analysis for consecutive 90 days were performed to monitor the associated changes in the marrow’s physiology after 30th, 60th and 90th days of chronic pesticide exposure. The peripheral blood showed maximum lowering of the blood cell count after 90 days which actually reflected the bone marrow scenario. Severe depression of BMHSC population, immune profile of the bone marrow derived cells and reduction of adherent cell colonies pointed towards an essentially empty and hypoplastic marrow condition that resembled the disease aplastic anemia. The changes were accompanied by splenomegaly and splenic erythroid hyperplasia. In conclusion, this animal model allowed us a better understanding of clinico-biological findings of the disease aplastic anemia following toxic exposure to the pesticide(s) used for agricultural and industrial purposes.
Chronic aplastic marrow failure or, as it is more commonly termed, aplastic anemia is defined as pancytopenia accompanied by a hypocellular bone marrow. Laboratory and clinical findings have implicated an immunologic pathophysiology of the disease (1). In approximately one half of the cases, the syndrome becomes manifest after chronic exposure to toxic drugs or chemicals (2–6). Aplastic anemia has also long been linked with toxic exposure to pesticide(s). Reported findings from early studies included surprising associations of aplastic anemia with agricultural and house hold pesticide exposure in the developing countries (7, 8).
Usage of pesticides in the ecosystem leads to the development of various types of morphological and behavioral changes in individuals (9). The degree of toxicity depends upon the nature of pesticides and their environmental concentrations. Pesticides can be characterized on the basis of their function as insecticidal, herbicidal, fungicidal etc and also on the basis of their chemical nature, i.e. organophosphates and organochlorides (10, 11). Potentially hazardous environmental toxicants like pesticides display a broad spectrum of biological effects, being toxic not only to the target organisms but also to humans. There is substantial experimental and epidemiological evidence that many pesticides in widespread use, around the world are potential immunosuppressants (12–14). Experimental studies on human cell cultures and laboratory animals provided strong evidence that many pesticides are immuno-toxic (15, 16). Recent studies have also found that pesticides can induce changes in the immune system structure and function (17). Pesticides from both the organophosphate and organochloride groups have found to affect the developing immune system by depressing the proliferation and differentiation of the immune system parent cells. They also reduced the humoral and cell mediated immune responses in vivo (18–20). Pesticide induced immunosuppression might be substantially increasing the vulnerability to common diseases like pneumonia, gastro-enteritis and measles in the developing countries.
Despite the presence of several epidemiological studies, no systematic study has yet been carried out to show the effect of toxic exposure of these pesticides on the bone marrow physiology, hematopoietic stem cell population, stromal microenvironment and the immune functional potential of the bone marrow derived cells.
In this present study, we have established an animal model of hypoplastic marrow failure following chronic pesticide exposure in mice. Furthermore, we have showed the stage specific depression in the BMHSC population as shown by flowcytometric analysis and defects in the stromal microenvironment as evidenced by decrease in the short term adherent cell colony (STACC) formation. The toxic effect was associated with the decrease in the immune functional capacity of the bone marrow derived cells as documented through the cell mediated immune (CMI) parameter study. In conclusion, the experimental status produced by the pesticide induction in mice represented an animal model of human aplastic anemia in man with prolonged impairment of stem cell self-renewal and defects in the stromal microenvironment of bone marrow.
12 weeks old inbred Swiss albino mice of both sexes (30 in number) were housed at the animal house of the institute. The animals were kept in cages-6 animals per cage in a room at controlled room temperature (22±2°C). During the course of the study the animals were fed on a diet consisting of 25.0% protein, 10.0% fibre, 5.0% fat, 9.0% minerals and access to water ad libitum, under standard conditions with a 12 hour light dark period. The procedures followed were in agreement with the approved guide for the care and use of laboratory animals.
In a 90 days range finding study, we have divided adult mice into a) an experimental group receiving inhalation and dermal exposure of 5% aqueous solution of a mixture of common agricultural use pesticides from different organophosphate and organochloride origin (mainly includes Alphamethrin, Cypermethrin, Profenofos, Chloropyrofos) for 6-hours/day and 5-days/week up to 90 days (LD50 of 10% aqueous solution has been found to be 20 days) and b) a control group receiving inhalation and dermal exposure of only aqueous solution without any pesticide contamination for the same period of time. Body weights and water consumption were monitored throughout the study.
In the initial part of the study, mice were randomly selected for the peripheral blood hemogram in a group of six which looked pale or ill. In a time dependent observation, 30th, 60th and 90th day after the first exposure, approximately 300 μl of blood was collected by tail vein puncture using heparinized vials. Total and differential count of white blood cell (WBC), red blood cell (RBC) count, reticulocyte, platelet, neutrophil counts and hemoglobin percentage were taken for the detection of whole hemogram profile. In the initial part of the study, mice were randomly selected for the peripheral blood hemogram.
On 30th, 60th and 90th day after the first date of exposure, animals were sacrificed in a group of six at a time to isolate long bones (femur, tibia, and fibula). Bone marrow was taken out by flushing with RPMI-1640 media using 26 gauge needle syringe and the cells were mixed well with repeated pipeting. RBCs were then depleted using RBC lysing solution (BD Biosciences, USA). The cells were collected after passing through cell strainer and washed in phosphate buffered saline (PBS) and finally transferred to RPMI-1640 and the cell distribution was noted by counting the cells for each of the control and experimental groups of animals.
Monoclonal antibodies against Sca-1, c-kit and mouse IgG isotype controls were obtained from BD-Biosciences, USA. Approximately 1×106 bone marrow cells were incubated for 30 minutes at 37°C in the dark with 2 μl of phycoerythrin (PE) labeled anti mouse Sca1 mAb and 2 μl of fluorescence isothyocyanate (FITC) conjugated c-kit mAb and then washed twice in PBS to wash off the excess fluorescence. Stained cells were analyzed with BD-FACS Calibur (Becton Dickenson, USA) using cell quest pro software.
For the short term adherent cell (STAC) culture, the bone marrow derived cells were suspended in RPMI-1640 at a concentration of 4×106 cells/ml of media, supplemented with 15% fetal bovine serum (FBS), 100 U/ml penicillin, 100 U/ml streptomycin and 0.01% (V/V) mercaptoethanol. Four 35×10 mm Petri dishes each for the experimental and control groups were plated with 3 ml of media containing 4×106 cells and placed in a CO2 incubator at 37°C and 5% CO2 atmosphere. At every 72 hours interval the media was drained off from each Petri dish and fresh media supplemented with 20% fetal bovine serum (FBS) and 0.01% (V/V) mercaptoethanol, added for the maintenance of the culture. After 16 days the numbers of colonies were scored using an inverted microscope.
The functional efficacy of the bone marrow derived cells isolated as above has been carried out in each group as follows.
Spontaneous E-rosette (21): Briefly 0.25 ml of 1% S-RBC was allowed to react with 0.25 ml of 4×106 cells /ml effectors (Bone marrow derived cells) at 37°C for 15 minutes, and finally centrifuged and kept at low temperature (4°C) overnight.
Nitroblue tetrazolium (NBT) reduction assay: As described earlier (Law et al., 2001) effector: target ratio was maintained at 10 : 1 in the presence of NBT- which is reduced to blue formazan as phagocytosis proceeds. The preparation was incubated for 18 hours at 37ºC in 4% CO2 humidified atmosphere. Finally the reaction was stopped by adding 0.1N chilled HCl and pellet was extracted with boiling pyridine for the reduced blue formazan to appear in the supernatant. The intensity of colour assayed spectrophotometrically at 530 nm provided a direct measure of the extent of phagocytosis. Results were expressed as a percentage (%).
Cytotoxicity assay: The Effector: Target ratio has been maintained at 10 : 1. Approximately 1×106 bone marrow cells were incubated with 1×105 tumour cells (Sarcoma-180) for 18 hours at 37°C. Finally, after 18 hours of incubation equal volume of cell suspension was mixed with equal volume of 0.4% trypan blue dye solution and the tumour cell viability was determined by counting them on hemocytometer chamber.
Statistical analysis of results was performed using Students’t-test of the standard deviation from the mean of different data. All results were evaluated statistically by applying the SPSS-PC package (Version 9.0, SPSS, Chicago, Illinois, USA). A probability of p<0.01 was considered statistically significant.
The normal weight gain pattern was not observed in the pesticide exposed mice group throughout the experimental period. After 90 days the averaged weight of the animals were 21±2.75 gms compared to the normal controls (26.0±2.0 gms) (Fig. 1). Hair loss and skin lesions were visible after 30 days of exposure which were recovered substantially after 50∼70 days. Mice with pesticide exposure showed large erythroid spleens (Fig. 2B) compared to the normal counterpart (Fig. 2A) and retardation of general body growth and maturation.
In order to ascertain the clinical status of the disease, the hemogram of the peripheral blood including hemoglobin percentage, reticulocyte, WBC, neutrophil and platelet counts were determined following standard laboratory techniques. The results (Table 1) showed a moderate depression in the hemoglobin level with uniformly reduced corpuscular counts including WBCs, RBCs and neutrophils in the experimental group of animals compared to the normal. The average reticulocyte counts were significantly low (0.43%) in the experimental group throughout the course of the study, compared to the normal (0.89%).
Phenotypic characterization of Sca-1+c-kit+ population in bone marrow derived cells, indicated a significant lowering in the primitive stem cell population in the initial phase (30 days post exposure) of the pesticide exposure with a value of 1.46% (Fig. 3B) Sca-1+c-kit+ cells compared to the normal value of 9.33% (Fig. 3A). In the later phase (60th to 90th days post exposure), further, the percentage of Sca-1+c-kit+ cells within the bone marrow decreased severely at the end of 60th day (0.68%) (Fig. 3C) and 90th day (0.69%) (Fig. 3D). The primitive Sca-1+ c-kit+ population within the bone marrow of pesticide exposed animals showed an overall scanty profile throughout the time course of the experiment compared to the normal (Fig. 4).
Marrow cellularity for the experimental group of animals was always less than that of controls throughout the study. Short term adherent cell colony (STACC) formation per femur in pesticide exposed animals (Fig. 5B) was always depressed compared to the normal (Fig. 5A). The quantitative chart of STACC (Fig. 6) showed that, colony forming ability of the bone marrow derived cells decreased significantly with the increase of the days of exposure. In the initial phase (30 days post exposure), the experimental group of animals showed 67% reduction in the number of colonies formed compared to the normal control group. After 60th day, the reduction in the number of colony was more severe with a value of 79%. By 90th day the experimental animals showed 92% reduction in the STACC compared to the normal counterpart. The mean STACC for the experimental group was only 21% of the control value throughout the study.
The cells isolated from the bone marrow of experimental and control groups of animals were assayed for immunological efficacy from three different aspects a) spontaneous E-rosette formation b) phagocytic capacity c) cytotoxic efficacy.
Spontaneous E-rosette formation: Bone marrow derived cells from pesticide exposed aplastic anemic mice showed a significant decrease in spontaneous rosette forming capacity compared to that found in normal control mice (p<0.001) (Fig. 7A). The decrease became more significant with the progression of time.
Phagocytic capacity: Phagocytic capacity as observed by NBT reduction test revealed a decreased efficacy in experimental group of mice compared to the normal counterpart (p<0.001) (Fig. 7B). The decrease became highly significant after 90 days post pesticide exposure.
Cytotoxic efficacy: As evident from the data (Fig. 7C) the cytotoxic efficacy of the bone marrow derived cells were found to be highly reduced (p<0.005) in pesticide exposed experimental group of mice compared to that of normal mice.
Usage of potentially hazardous environmental toxicants like pesticides lead to development of various types of morphological, physiological and biochemical changes in various mammalian cells which suppress the normal immune response to invading pathogens (22). Improper usage of pesticides resulted in neurological and hematological complications in individuals. The degree of immunotoxicity depends upon the nature of pesticides, their environmental concentration and factors such as temperature, humidity, pH etc.
The present study represented an effort to develop an animal model of chronic aplastic marrow failure following long term pesticide exposure in swiss albino mice. We have used a 5% aqueous mixture of common agriculturally used pesticides for the potential induction of hypoplasia in the experimental animals. The disease progression was monitored by peripheral blood hemogram. Mice with chronic pesticide exposure generally showed markedly erythroid spleen which signifies the increase in extra-medullary hematopoiesis in aplastic anemia. The physiological and morphological changes in the bone marrow was associated with quantitative depression in the number of pluripotent stem cells (Sca1+c-kit+ cells) and significant decrease in the short term adherent cell (STAC) colony formation. The immune functional capacity of the bone marrow derived cells showed deterioration in the pesticide exposed group of animals.
During the disease progression the bone marrow failure got reflected in the peripheral blood pancytopenia that includes neutropenia, thrombocytopenia and declined hemoglobin level along-with moderate to severe decrease in the number of reticulocytes. In the initial phase (30 days post exposure) of the study the peripheral blood showed moderate depression in the blood cell and the depression was significantly increased after 60th and 90th days of pesticide exposure. The scanty peripheral blood profile reflected the bone marrow failure characterized by damage of primitive stem cell (Sca1+c-kit+ cells) population that has long term repopulating (LTR) activity. Here we wanted to show the quantitative damage done to this primitive population during different stages of the disease progression. During the initial phase (30 days post exposure) of the study, the percentage of sca1+c-kit+ cells (1.46%) was significantly affected compared to the normal (9.33%). In the latter phase (60th to 90th days post exposure), the percentage of sca1+c-kit+ cells declined which (0.68% and 0.69%) indicated a permanent and prolonged defect in the stem cell proliferation leading to severe marrow failure and death (23–25).
The pesticide induced bone marrow damage can affect the hematopoietic stem cell (HSC) population or the adherent stromal cell population or may affect the both (26–28). Short term adherent cell colony formation (STACC) per femur was used as a quantitative assay to show the defects in the adherent stromal cell population which played vital role in hematopoiesis and probably the representative of the in vivo microenvironment, after chronic pesticide exposure. The correlation between defects in the adherent cells with bone marrow failure was first indicated by Hays et al and Anderson et al, who showed that confluent adherent cells derived from marrow failure mice failed to support stem cells as well as adherent cells from normal mice. For effective hematopoiesis it is essential to have an intact microenvironment (stromal cells) upon which the HSC self-renew and differentiate. Therefore, the failure of HSC in aplastic anemia can be the result of damage of the adherent stromal cells. The functional role of stromal cells was investigated in vitro through colony forming assay. Short term colony forming ability by the adherent stromal cell population was profoundly depressed in pesticide exposed group of animals throughout the time course of the experiment. With the progression of the disease the colony forming ability was almost 92% decreased compared to the normal at the end of 90th days post pesticide exposure. The quantitative depression in the primitive sca1+c-kit+ HSC population is dependent on this adherent stromal cell damage and the stromal damage plays an important role in limiting the hematopoiesis. More likely, pesticide affects stromal cells, as measured by STACC assay, and hematopoietic stem cells, as documented through flowcytometric analysis, in the same degree. The marrow failure may have occured because of an imbalance in the replication and differentiation between the marrow stem and stromal progenitor cells. Pesticides apparently damage the hematopoietic stem and stromal cells to cause impairment in the hematopoiesis and thereby leading to severe bone marrow failure and death of many of the animals.
The functional efficacies of the bone marrow derived cells were found to be significantly depressed in terms of E-rosetting, phagocytic capacity and cytotoxic efficacy compared to the normal through out the due course of the experiment. Decreased E-rosetting capacity, deficient phagocytic capacity and reduced cytotoxic efficacy can account for both a quantitative and qualitative deterioration of the bone marrow cells under the condition of severe bone marrow suppression following chronic pesticide exposure.
In conclusion, pesticide damages the hematopoietic stem and stromal cell population both quantitatively and qualitatively to induce impairment in the normal hematopoiesis and thus ultimately leads to severe bone marrow failure with concurrent prevalance of the disease aplastic anemia.
ACKNOWLEDGMENTS
We are thankful to the Department of Science and Technology, Govt. of India and Department of Science and Technology, Govt. of West Bengal for their sponsorship. We also thankful to the Director, Calcutta School of Tropical Medicine for providing us space for research.
References
2. Chatterjee S, Basak P, Das P, Das M, Pereira JA, Dutta RK, Chaklader M, Chaudhuri S, Law S. Primitive Sca-1 positive bone marrow HSC in mouse model of aplastic anemia: a comparative study through flowcytometric analysis and scanning electron microscopy. Stem Cell Int. 2010. 1–7.


3. Chatterjee S, Basak P, Das M, Das P, Pereira JA, Dutta RK, Chaklader M, Chaudhuri S, Law S. Kinetic impairment of hematopoietic stem cells in experimentally induced leukemia and aplastic anemia: an inverse correlation. Journal of Stem Cells. 2009. 4:179–189.
4. Chen J. Animal models for acquired bone marrow failure syndrome. Clin Med Res. 2005. 3:102–108.
5. Fitchen JH, Cline MJ. Effect of granulopoietic stress in mice with “latent” bone marrow failure. Exp Hematol. 1980. 8:788–794.
6. Fleming LE, Timmeny W. Aplastic anemia and pesticides. an etiologic association? J Occup Med. 1993. 35:1106–1116.
7. Sanchez-Medal L, Castanedo JP, Garcia-Rojas F. Insecticides and aplastic anemia. N Engl J Med. 1963. 269:1365–1367.


8. Wang HH, Grufferman S. Aplastic anemia and occupational pesticide exposure: a case control study. J Occup Med. 1981. 23:364–366.
9. Nakao S, Feng X, Sugimori C. Immune pathophysiology of aplastic anemia. Int J Hematol. 2005. 82:196–200.


10. Jamil K, Shaik AP, Mahboob M, Krishna D. Effect of organophosphorus and organochlorine pesticides (monocrotophos, chloropyriphos, dimethoate and endosulfan) on human lymphocyte cultures in vitro. Drug Chem. Toxicol. 2004. 27:133–144.


11. Whitney KD, Seidler FJ, Slotkin TA. Developmental neurotoxicity of chlorpyrifos: cellular mechanism. Toxicol Appl Pharmacol. 1995. 134:53–62.
12. Broughton A, Thrasher JD, Madison R. Chronic health effects and immunological alterations associated with exposure to pesticides. Comments on Toxicol. 1990. 4:59–71.
13. Repetto R, Baliga SS. Pesticide and immunosuppression: the risks to public health. Health Policy Plann. 1997. 12:97–106.
14. Vojdani A, Ghoneum M, Brautbar N. Immune alteration associated with exposure to toxic chemicals. Toxicol Ind Health. 1992. 8:239–254.


15. Botnick LE, Hannon EC, Hellman S. A long lasting proliferative defect in the hematopoietic stem cell compartment following cytotoxic agents. Int J Radiat Oncol Biol Phys. 1979. 5:1621–1625.


16. Wells MS, Nerland DE. Hematotoxicity and concentration-dependent conjugation of phenol in mice following inhalation exposure to benzene. Toxicol Lett. 1991. 56:159–166.


17. Twomey JJ, Douglass CC, Sharkey O Jr. The monocytopenia of aplastic anemia. Blood. 1973. 41:187–195.


18. Rugman FP, Cosstick R. Aplastic anemia associated with organochlorine pesticides: Case reports and review of evidence. J Clin Pathol. 1990. 43:98–101.


19. Dempster AM, Synder CA. Kinetics of granulocytes and erythroid progenitor cells are affected differently by short-term, low-level benzene exposure. Arch. Toxicol. 1991. 65:556–561.


20. Loge JP. Aplastic anemia following exposure to benzene hexachloride (lindane). JAMA. 1965. 193:104–114.


21. Kinchington D, Ng T. Separation and maintenance of primary T and B-lymphocytes. Pollard JW, Walker JM, editors. Basic Cell Culture Protocols. Totowa New Jersey: Humana Press;1997. 94–95.


22. Thomas PT, Busse WW, Kerkvliet NI, Luster Ml, Munson AE, Murray M, Roberts D, Robinson M, Silkworth J, Sjoblad R, Smialowicz R. Immunologic effects of Pesticides. In: the effects of pesticides on human health. Advances in Modern Environmental Toxicology. 18:Baker SR, Wilkinson CF, editors. Princeton, NJ: Princeton Scientific Publishing Co;1990. 261–295.
23. Morley A, Trainer K, Blake J. A primary stem cell lesion in experimental chronic hypoplastic marrow failure. Blood. 1975. 45:681–688.


24. Kubota K, Mizoguchi H, Miura Y, Kano S, Takaku F. Experimental hypoplastic marrow failure in the mouse. Exp Hematol. 1978. 6:791–800.
25. Boggs DR, Boggs SS. Editorial hypothesis: the pathogenesis of aplastic anemia: a defective pluripotent hematopoietic stem cell with inappropriate balance of differentiation and self-replication. Blood. 1976. 48:71–76.


26. Fried W, Kedo A, Barone J. Effects of cyclophosphamide and busulfan on spleen colony-forming units and on hematopoietic stroma. Cancer Res. 1977. 37:1205–1209.
27. McManus PM, Weiss L. Busulfan-induced chronic bone marrow failure: changes in cortical bone, marrow stromal cells, and adherent cell colonies. Blood. 1984. 64:1036–1041.


28. Knospe WH, Husseini SG, Chiu KM, Fried W. Immunologically mediated aplastic anemia in mice: evidence of hematopoietic stromal injury and injury to hematopoietic stem cells. Exp Hematol. 1994. 22:573–581.
Fig. 1.
Weight gain pattern of the pesticide exposed group of mice, showed significant difference compared to the normal mice throughout the experimental. At about 90th day, the average weight of the pesticide exposed mice was 21.5±2.75 gms compared to the normal controls (26.0±2.0 gms).
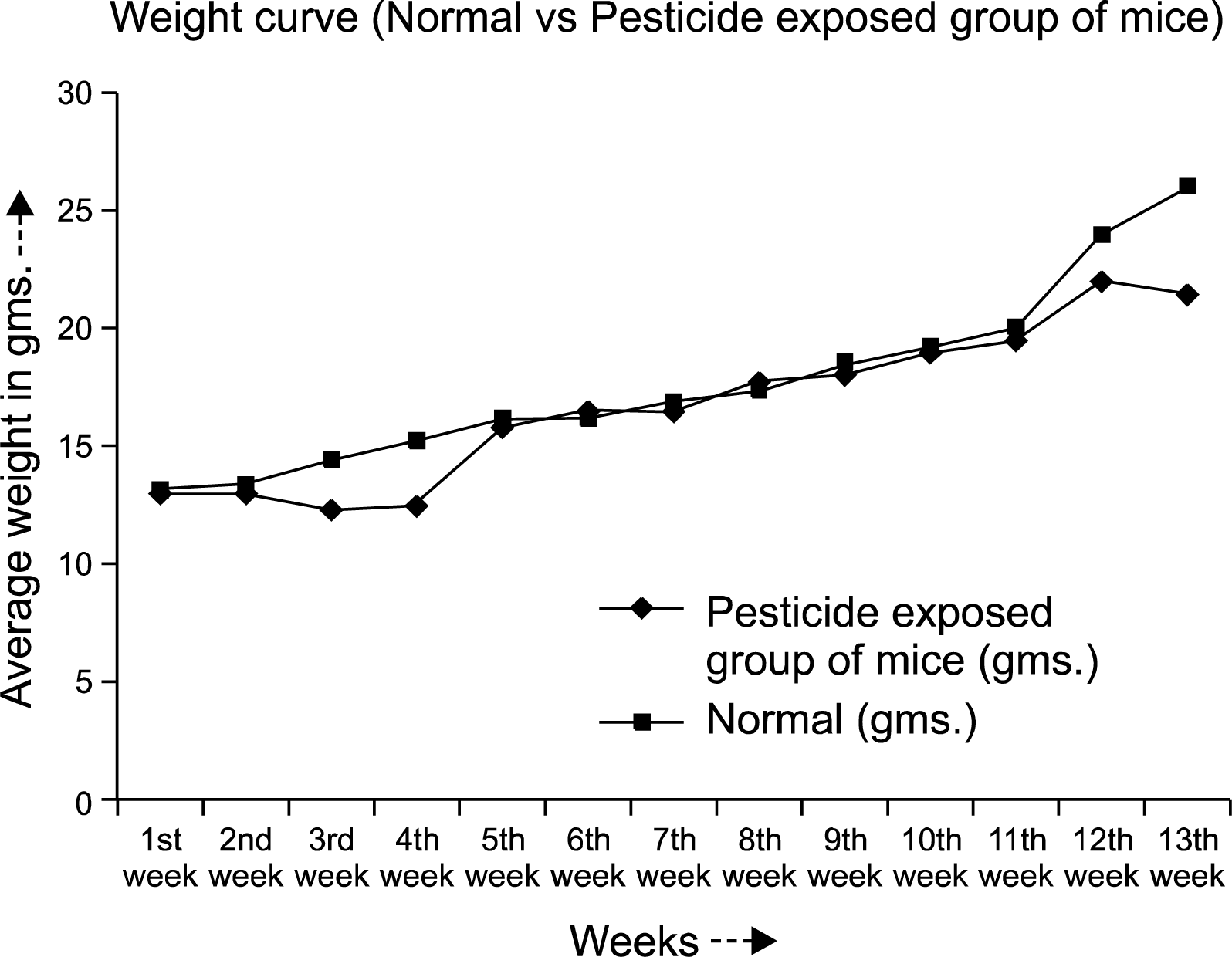
Fig. 2.
(A) Spleen from the normal control group of mice. (B) Large, hyper-erythroid spleen from the pesticide exposed animals indicates the increase in extramedullary hematopoiesis in the stressed condition.
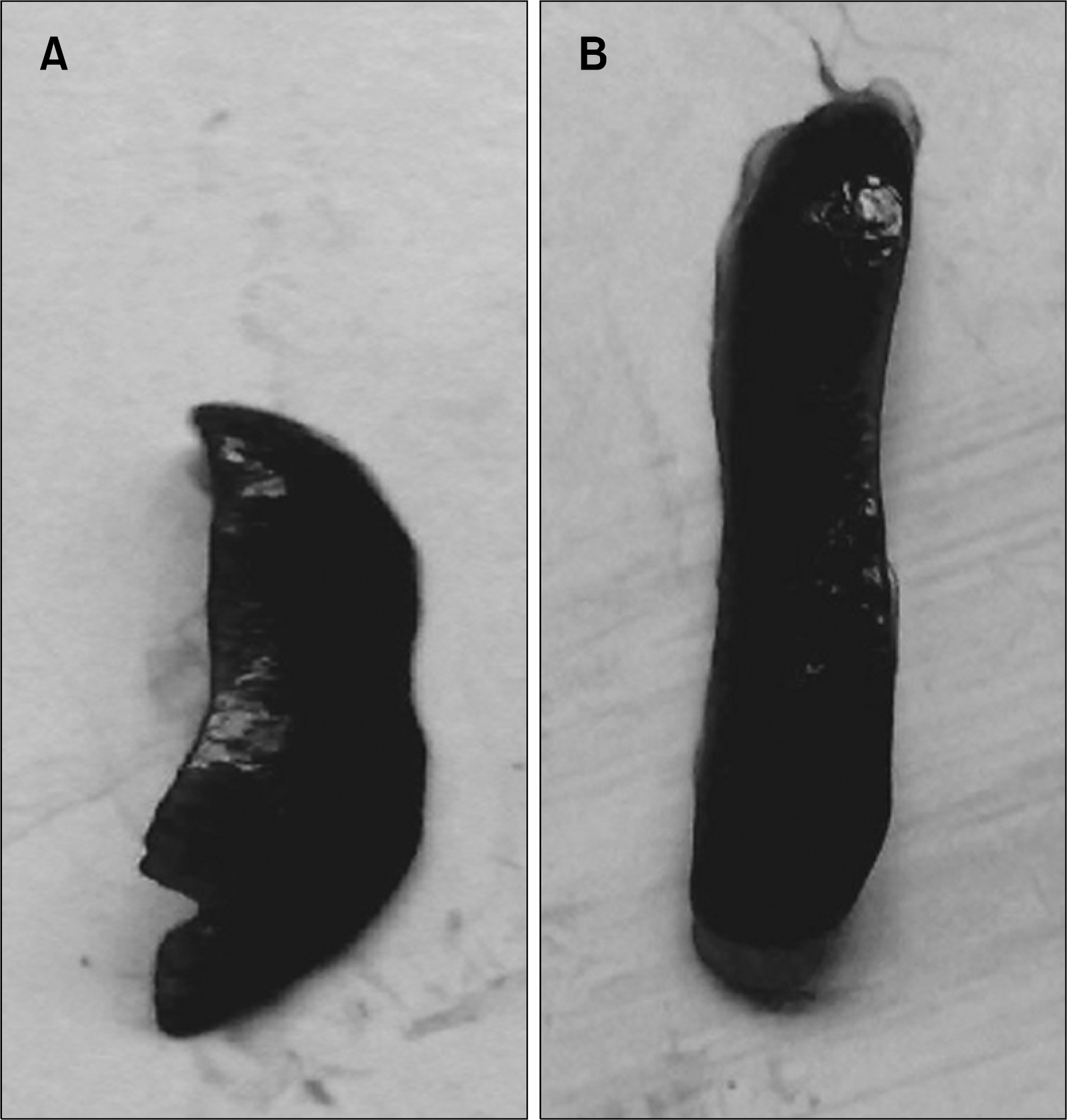
Fig. 3.
(A) Phenotypic characterization of Sca-1 and c-kit receptor expression from the normal mouse bone marrow cells. The normal bone marrow contains 9.33% dual positive (Sca1+c-kit+) cells. (B) Phenotypic characterization of Sca-1 and c-kit expression by the bone marrow cells from 30 days post pesticide exposed group of mice. Severe reduction in the percentage of Sca1+c-kit+ (1.46%) cells has been observed in this group of animals. (C) Phenotypic characterization of Sca-1 and c-kit receptor expression by the bone marrow cells from 60 days post pesticide exposed group of mice. The bone marrow from this group of animals showed further decrease in the Sca1+c-kit+ (0.68%) population. (D) Phenotypic characterization of Sca-1 and c-kit receptor expression by the bone marrow cells from 90 days post pesticide exposed group of mice. Severe reduction in the percentage of Sca1+c-kit+ (0.69%) cells resembled the previous group.
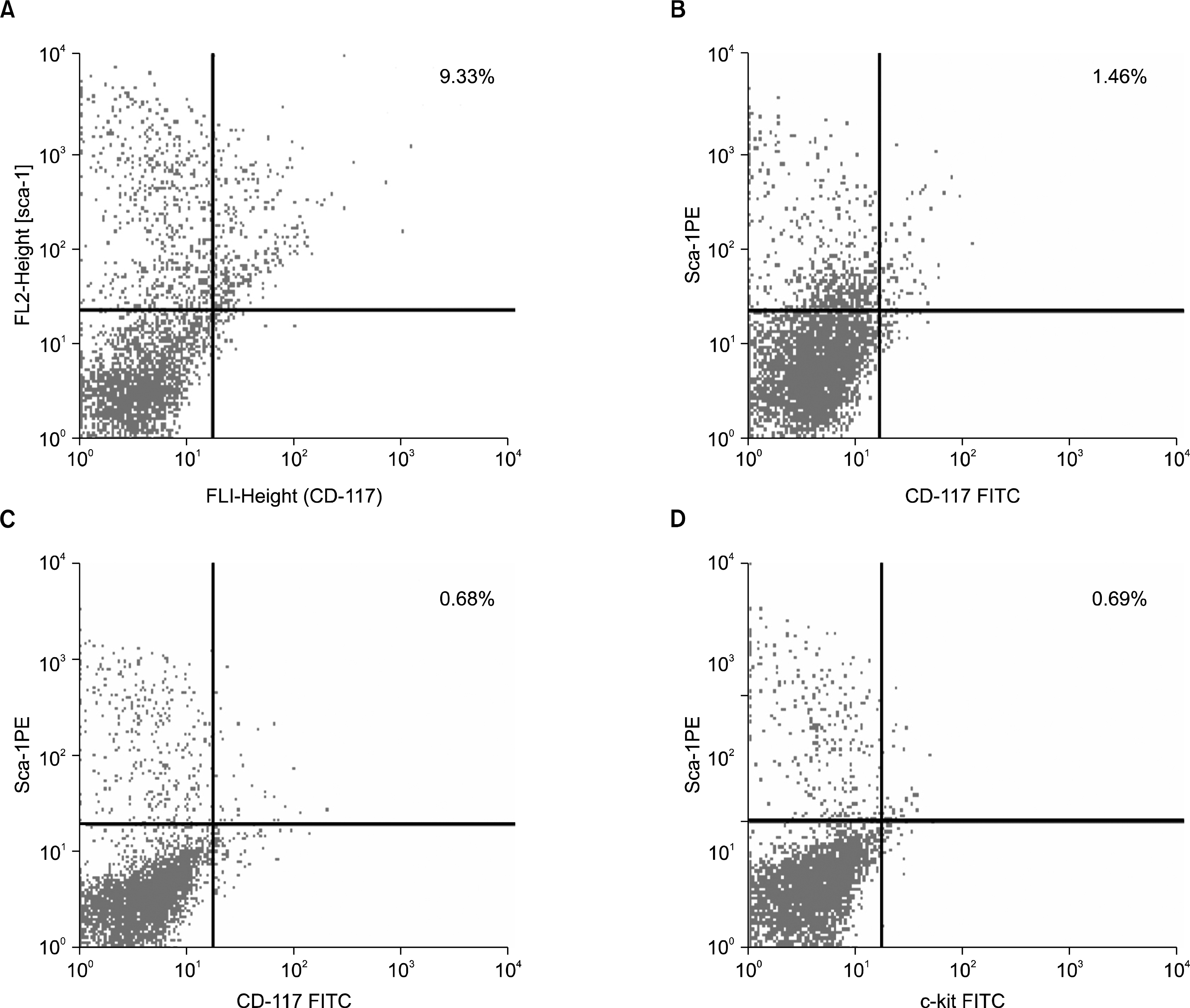
Fig. 4.
The quantitative distribution chart of Sca1+c-kit+ cells within the bone marrow of the normal and pesticide exposed animals at different time course of the experiment, expressed as percent mean.

Fig. 5.
(A) The short term adherent cell colony (STACC) derived from normal bone marrow adherent cell culture at day 16. Colonies were colorless and compact. (B) Absence of prominent adherent cell colony after 16 days of bone marrow adherent cell culture from the pesticide exposed group of mice. This image has been taken from the 90 days post pesticide exposed group of mice. However very low number of small colonies can be identified in that group.
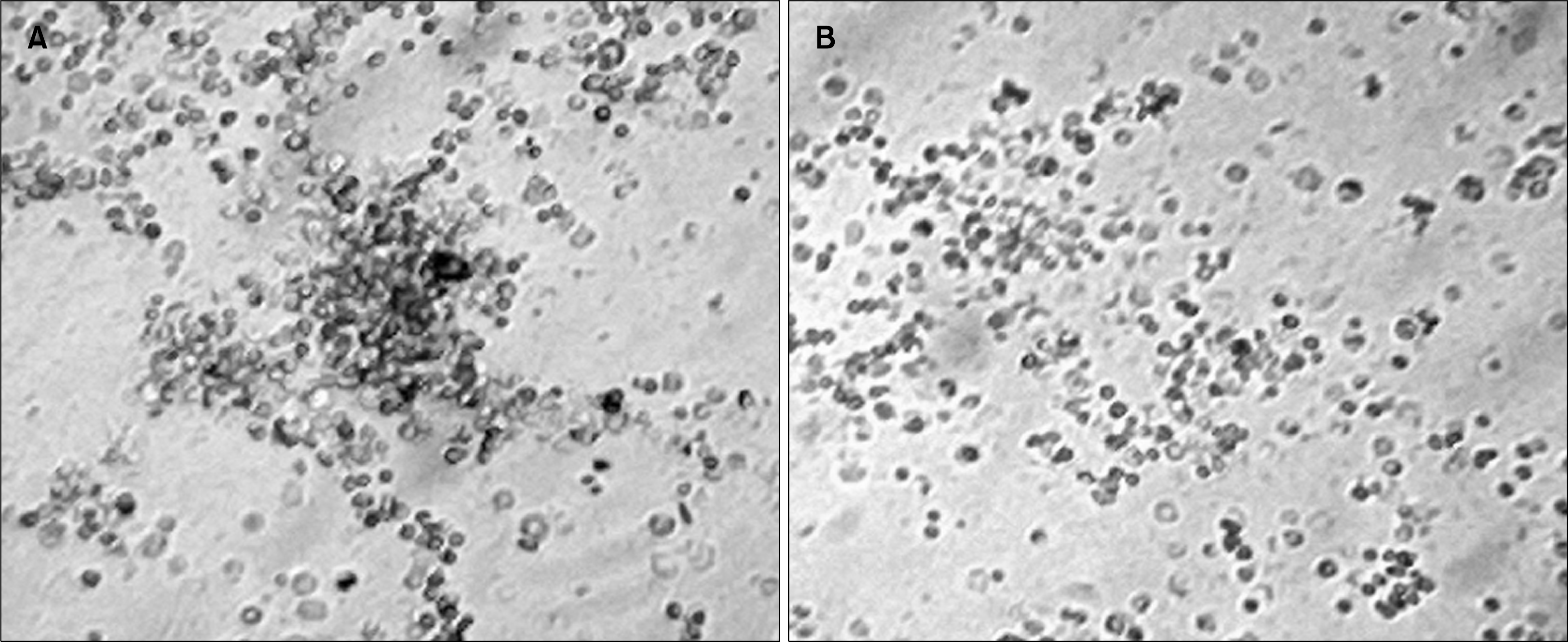
Fig. 6.
The quantitative distribution chart of STACC data from normal and pesticide exposed animals at different time course of the study, expressed in - no. of colonies/10,000 cells.
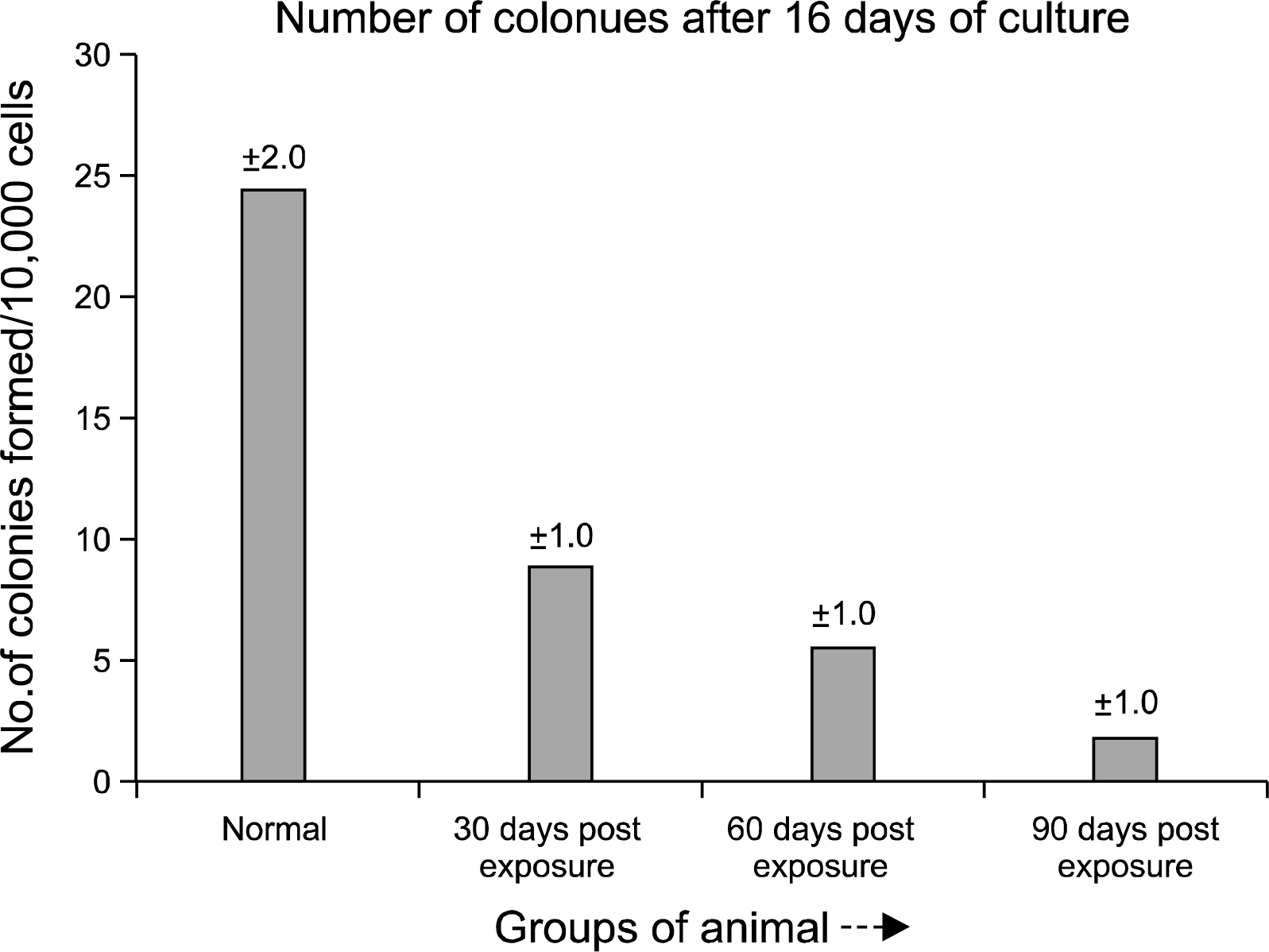
Fig. 7.
(A) Spontaneous E-rosette forming capacity of bone marrow derived cells (BMC) from the different pesticide exposed groups of mice compared with the normal. The pesticide exposed groups suffered a significant decrease in the E-rosette forming capacity compared to the normal. The 90 days post pesticide exposed group suffered maximum damage to the immune cells in terms of E-rosette forming ability. (B) The phagocytic capacity of the bone marrow derived cells (BMC) from different pesticide exposed groups of animals compared to the normal. The pesticide exposed groups showed a significant reduction in the phagocytic capacity compared to the normal control group. (C) Cytotoxic efficacy of the bone marrow derived cells (BMC) from different pesticide exposed groups of animals compared with the normal. The pesticide exposed groups showed a significant reduction in the cytotoxic efficacy compared to the normal healthy group.
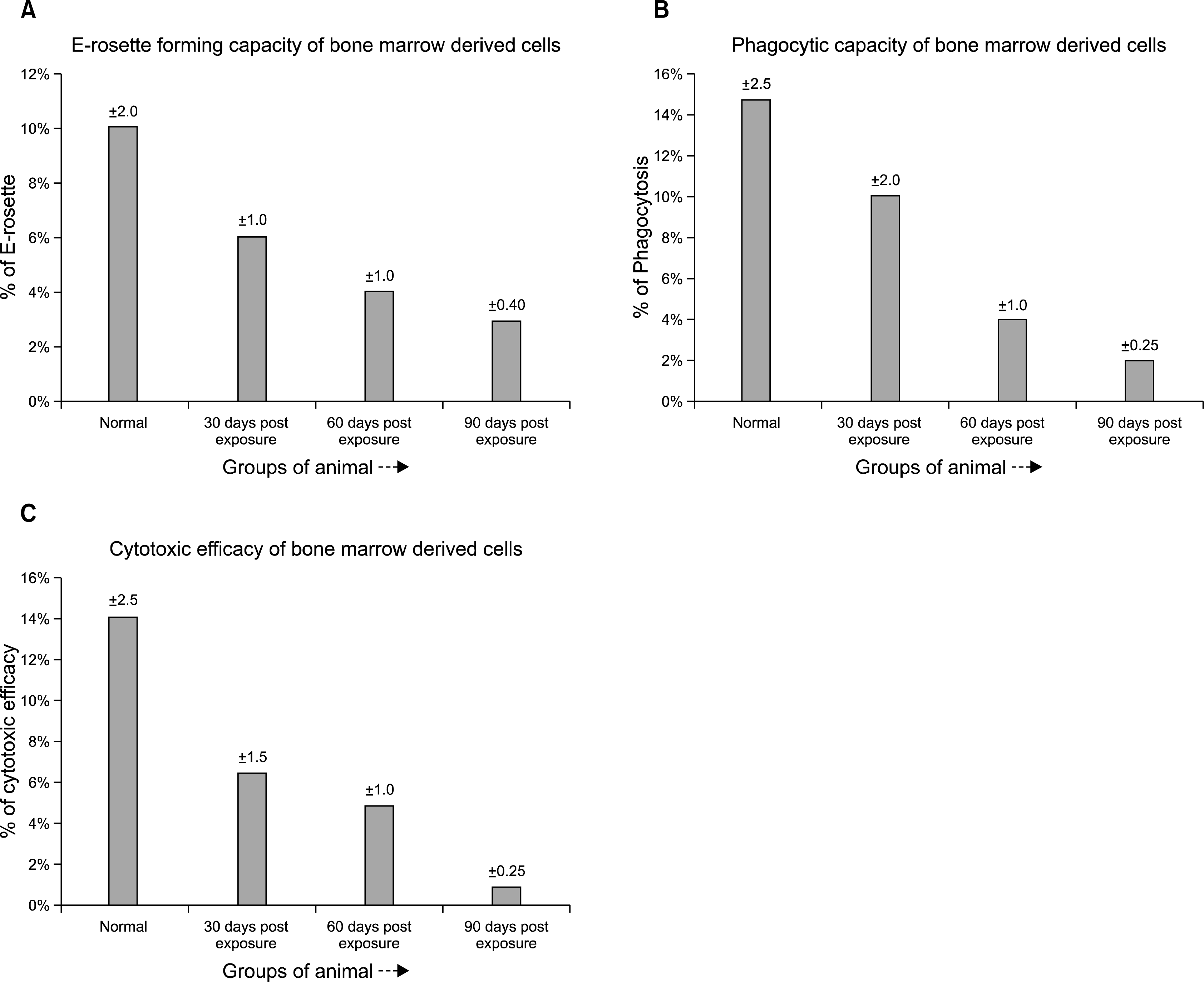
Table 1.
Peripheral blood hemogram of the pesticide exposed group of mice showed uniformly reduced corpuscular counts such as white blood cells (WBC), red blood cells (RBC) and platelets at 30th and 90th days after pesticide exposure and moderate decrease in the hemoglobin level compared to the normal control group. Reticulocyte and neutrophil counts were also significantly low