Abstract
The peroxisome proliferator-activated receptors (PPARs) are ligand-activated transcription factors that belong to the nuclear receptor family. It is well known that PPARs function as regulators of lipid and lipoprotein metabolism and glucose homeostasis, as well as influence cellular proliferation, differentiation and apoptosis. However, the role of the PPARs with regard to embryonic stem (ES) cells remains unknown. We will review the function of the PPARδ, one of the three PPAR isoforms, α, δ (also called β/δ), and γ, in ES cells and its role in embryo development. In addition, pluripotent mouse ES cells can be expanded in large numbers in vitro due to the process of symmetrical self-renewal. Here we describe how PPARδ sustains ES cell proliferation.
Peroxisome proliferator-activated receptors (PPARs) are ligand-activated transcription factors that belong to the nuclear receptor superfamily (1). They have been identified in a variety of different species including the xenopus, mouse, rat, and humans (2). After the isolation of PPARα (NR1C1), as the receptor mediating peroxisome proliferation in rodent hepatocytes in 1990 (3), two related isotypes, PPAR δ (NR1C2; also called PPARβ/δ) and PPARδ (NR1C3) located on chromosomes 15, 17 and 6 in the mouse and chromosomes 22, 6 and 3 in human, were identified (4, 5). Once activated by their respective ligands, the PPARs control the transcription rate of a large panel of genes implicated in various physiological functions, including adipogenesis, lipid and glucose homeostasis, inflammation, cell proliferation, differentiation, and carcinogenesis (2, 6–8). PPARs heterodimerize with the retinoid X receptors and modulate gene expression of target genes containing peroxisome proliferators-responsive elements in response to ligand activation (9, 10).
The three isoforms of PPARs display distinct physiological and pharmacological activity that is dependent on their target genes and their tissue distribution (11, 12). PPARα, activated by polyunsaturated fatty acids and leukotriene B4, is expressed in tissues with high fatty acid catabolism such as the liver, heart, brown adipose tissues, kidney, and intestine. PPARγ, mainly expressed in adipocytes, macrophages, placenta, and other tissues, is activated by specific fatty acid metabolites, such as 15-deoxyprostaglandin J2 (15d-PGJ2), and by thiazolidinediones. Both PPARα and PPARγ response genes are involved in lipid homeostasis. Therefore, it is not surprising that the main functions of PPARα and PPARγ are related to glucose and lipid homeostasis (13–15). On the other hand, the ubiquitous distribution of PPARδ, (although gut, kidney, and heart express higher levels than other tissues) makes it difficult to associate PPARδ with a specific biological function (14). Although PPARδ is the least studied PPAR, it has been reported that PPARδ is associated with a diverse range of functions. Indeed, PPARδ participates in many biological processes, including lipid and glucose metabolism (16–19), epidermal maturation and wound healing (20–22), muscle development and function (23–25), tumorigenesis (26–30), inflammation (31, 32), and cytoprotection (33). In addition, combining multiple research approaches, PPARδ has been implicated in embryo development including stem/progenitor cell proliferation (34, 35), embryo implantation (13, 36, 37), embryo organogenesis, and diabetic embryopathy (38, 39). Therefore, this review will focus on the role of PPARδ in the ES cells.
PPARδ has been the most elusive among the three PPAR subtypes. Due to its broad tissue distribution, it is difficult to identify a specific function for this receptor. PPARδ has a broad expression pattern in adult animals, and is detected very early during embryogenesis (40). Several studies have shown that PPARδ is activated by a large variety of ligands and is implicated in the developmental and metabolic regulation of several tissues. PPARδ activators include fatty acids (41, 42), triglycerides (43), the cyclooxygenase (COX) product, prostacyclin (42), the COX/prostacyclin synthase derived endocannabinoid metabolites (44), and all transretinoid acid (45). A number of synthetic PPARδ ligands have been described including GW0742X, GW2433, GW9578, L-783, 483, GW501516, L-796,449, L-165,041, and compound F (46–48). In addition, GW501516 and GW0742 activate PPARδ at very low concentrations both in vivo and in vitro with a 1,000-fold selectivity over the other PPAR sub-types (49).
A mouse knockout model, although difficult to generate due to highly penetrant lethality (21, 50), indicated a role for PPARδ during embryo implantation, as well as in myelination of the brain, lipid metabolism and adiposity, and epidermal cell proliferation (37, 50, 51). In addition, PPARδ has been linked to cell differentiation, inflammation, cell motility, and cell growth (9, 52). Recent studies suggest that PPARδ plays a role in cell growth. For example, the PPARδ expression is increased in colorectal cancer cells compared with normal colon epithelial cells (30, 53). Treatment of GW501516, a PPARδ ligand, increased the number and size of intestinal polyps (29). In addition, PPARδ has been implicated in the growth of several other cell types including vascular smooth muscle cells (54), preadipocytes (17, 55) and epithelial cells (9). It has recently become clear that PPARδ has a function in epithelial tissues; however, this role continues to be debated in reports with inconsistent findings. Indeed, some reports suggest that ligand activation of PPARδ potentiates cell growth (56), whereas other reports suggest that ligand activation of PPARδ attenuates cell growth (57). Activation of PPARδ by its agonist increases COX2 expression in human hepatocellular carcinoma cells (58). In addition, PPARδ has been shown to mediate prostaglandin E2 (PGE2)-induced cell growth (59). More importantly, several reports have suggested that PPARδ-mediated cell growth is induced by COX/prostaglandin (PG) signal pathways. Consequently, the interplay between the PPAR δ and cytosolic phospholipase A2 (cPLA2)/COX2/PGE2 signaling pathways acts as a positive regulator in cell growth.
Apart from cell growth, the fact that PPARδ plays an important role in embryonic development is supported by the observation that most PPARδ-deficient mice die early during embryonic development due to a placental defect. A recent study showed that the genetic loss of PPARδ signaling does not influence ovulation, fertilization or preimplantation (60). Mouse embryos express PPARδ detectable at the two-cell stage (61) or eight-cell stage (36), and throughout the preimplantation period. Mouse blastocysts also express PPARδ in the inner cell mass and the trophectoderm. On the embryonic cell level, prostacyclin or the PPARδ agonist increased the embryonic cell mass, indicating that PPARδ is essential for embryo development, blastocyst hatching and implantation (36).
The elucidation of PPARδ modulation of ES cells will provide new insights into embryonic development. In addition, research on how normal embryonic development is regulated will provide new clues as to how to maintain stem cells in culture. The description of the interaction among signal molecules is a key to our fundamental understanding of stem cell proliferation and its translation into therapeutic strategies. However, information regarding the potential role of PPARδ in physiological and/or developmental processes is very limited, although PPARδ is widely expressed in embryonic tissue. There is evidence that PPARδ can modulate stem and progenitor cell expansion and a differentiated phenotype. In neural stem cells, PPARδ contributes to the maintenance of the un-differentiated and proliferative status, by regulating both the genes involved in cell cycle control, as observed in other cell types (54, 62, 63), and inhibiting the activity of the other PPARs, which may be involved in cellular differentiation (64–66). In addition, the PPARδ agonist GW 501516 has been shown to be a promoter involved in the development of adenosquamous carcinomas with high expression of stem cell markers CK19 and Notch1, as well as Proliferin, a growth factor that mediates many of the effects of the stem cell markers such as Musashi1, in mammary cells (67). PPARδ is expressed in the crypt cells of the small intestine and negatively regulates Hedgehog signaling to block differentiation (68), a process that would be expected to promote transformation. The association of Wnt activation with stem cell expansion, activation of β-catenin/T-cell factor (TCF) signaling by 3-phosphoinositide-dependent protein kinase 1 (PDK1) and the identification of PPARδ responsive genes suggest a common mechanism for the tumor promoting action of PPAR δ agonists that may involve stem and progenitor cell proliferation (35).
For successful implantation and pregnancy, recent evidence suggests that the implantation timing of PG signaling resulting from cPLA2, COX2 or lysophosphatidic acid receptor 3 plays an important role in the subsequent developmental processes (69–72). However, the underlying mechanism and the molecular link between the critical steps are still unclear. A previous report provided evidence that PPARδ serves as a molecular link that coordinates multiple signaling pathways in mouse ES cell proliferation allowing their self-renewal (Fig. 1) (34). In that study, high glucose (25 mM) increased PPARδ gene expression rather than PPARα or PPARγ in the ES cells. In addition, the PPARδ agonist, L-165,041 increased ES cell proliferation, but the PPARδ antagonist, GW9662 or the PPARδ specific small interfering RNAs inhibited the effects of high glucose. Moreover, high glucose increased COX2 and PGE2 synthesis activating PPARδ, which increases cell cycle regulatory protein expression such as cyclins and cyclin dependent kinases (CDKs). It has been consistently shown that COX2-derived PGE2 and PGI2 mediate their function via PPARδ receptors during the early steps of decidualization in mice (73). Although the exact mechanisms involved in COX2-derived PG activation of PPARδ has not been completely elucidated, previous reports have suggested that the activity of PGE2 is mediated by possible activation of the EP2 receptor, which increases the cAMP levels (74–76) and PPARδ receptors. Previous work has shown that embryos from streptozotocin (STZ)-induced diabetic rats have diminished PGE2 content, although they can produce PGE2 in large amounts (77). One can speculate that arachidonic acid (AA) might be depleted if PGE2 generation and release is increased in the diabetic embryo in order to maintain the intracellular PGE2 levels (39). In addition, it has been suggested that ligand activation of PPARδ induces the expression of COX2 (56), which could theoretically promote cell growth and inhibit apoptosis through mechanisms that involve the production of prostaglandins. These data raise the possibility that impaired activation of PPARδ may alter the lipid signaling required for normal self-renewal of ES cells, which raises that possibility that PPARδ might be a putative target for the maintenance of ES cell characteristics. The results of a previous study suggested that the loss of PPARδ leads to reduction of the phosphorylation status of Akt and STAT3 in the trophoblast (60). Akt is a down-stream pathway of PPARδ signaling that is active during cell proliferation and survival (60); it was observed that PPARδ null mice were not able to progress through the normal developmental steps. The PI3K/Akt signaling pathway also has been implicated in ES cell self-renewal in studies of ES cells without PTEN (78). Thus, this observation supports the participation of PPARδ in ES cell proliferation and maintenance of self-renewal. In addition, several groups have shown that STAT3 is an important signal transducer and activator in the maintenance of pluripotency and the propagation of mouse ES cells (79–81). Although STAT3 phosphorylation with LIF was not influenced by the PPARδ −/− trophoblast, the STAT3 was not phosphorylated by the PPARδ agonist. This suggests that PPARδ activity not only plays a role in normal development but also is involved in ES cell proliferation and self-renewal. It has been shown that STAT3 could direct the expression of key regulators of the mitotic cycle in ES cells and stimulates their entry into the S phase (79). Thus, it is possible that PPARδ plays a role in inducing cell cycle molecules that are involved in ES cell proliferation. Gene expression profiling experiments will help gain insights into the mechanisms involved in PPARδ activity during the process of self-renewal.
Over the past few years, knowledge of the physiological activity of PPARδ has expanded. The study of PPARδ characteristics has added to improve understanding of cell physiology. PPARδ has been implicated in many cell processes, from the embryo to adult cells, and from cell proliferation to cell differentiation; it has been shown to be crucial for energy homeostasis. Until recently, because the function of PPARδ remained elusive, the therapeutic potential of PPARδ agonists for lipid and glucose metabolism, embryo development and wound healing has been tested in mice only. Our current understanding of PPAR δ has demonstrated that PPARδ also plays a critical role in ES cell proliferation. Future studies will likely clarify the physiological role of PPARδ in ES cell growth and differentiation.
ACKNOWLEDGMENTS
This research was supported by a Grant SC 2270 from the Stem Cell Research Center of the 21st Century Frontier Research Program and BK 21 project, funded by the Ministry of Education, Science and Technology, Republic of Korea.
References
1. Hihi AK, Michalik L, Wahli W. PPARs: transcriptional effectors of fatty acids and their derivatives. Cell Mol Life Sci. 2002. 59:790–798.


2. Michalik L, Desvergne B, Dreyer C, Gavillet M, Laurini RN, Wahli W. PPAR expression and function during vertebrate development. Int J Dev Biol. 2002. 46:105–114.
3. Issemann I, Green S. Activation of a member of the steroid hormone receptor superfamily by peroxisome proliferators. Nature. 1990. 347:645–650.


4. Borel V, Gallot D, Marceau G, Sapin V, Blanchon L. Placental implications of peroxisome proliferator-activated receptors in gestation and parturition. PPAR Res. 2008. 758–562.


5. Dreyer C, Krey G, Keller H, Givel F, Helftenbein G, Wahli W. Control of the peroxisomal β-oxidation pathway by a novel family of nuclear hormone receptors. Cell. 1992. 68:879–887.


6. Akiyama TE, Meinke PT, Berger JP. PPAR ligands: potential therapies for metabolic syndrome. Curr Diab Rep. 2005. 5:45–52.


7. Michalik L, Desvergne B, Wahli W. Peroxisome-proliferator-activated receptors and cancers: complex stories. Nat Rev Cancer. 2004. 1:61–70.


8. Rosen ED, Walkey CJ, Puigserver P, Spiegelman BM. Transcriptional regulation of adipogenesis. Genes Dev. 2000. 14:1293–1307.


9. Burdick AD, Kim DJ, Peraza MA, Gonzalez FJ, Peters JM. The role of peroxisome proliferator-activated receptor-β/ δ in epithelial cell growth and differentiation. Cell Signal. 2006. 18:9–20.


10. Mangelsdorf DJ, Thummel C, Beato M, Herrlich P, Schütz G, Umesono K, Blumberg B, Kastner P, Mark M, Chambon P, Evans RM. The nuclear receptor superfamily: the second decade. Cell. 1995. 83:835–839.


11. Bishop-Bailey D, Hla T, Warner TD. Bisphenol a diglycidyl ether (BADGE) is a PPARγ agonist in an ECV304 cell line. Br J Pharmacol. 2000. 131:651–654.


12. Buchan KW, Hassall DG. PPAR agonists as direct modulators of the vessel wall in cardiovascular disease. Med Res Rev. 2000. 20:350–366.


13. Huang JC. The role of peroxisome proliferator-activated receptors in the development and physiology of gametes and preimplantation embryos. PPAR Res. 2008. 732303.


14. Kersten S, Desvergne B, Wahli W. Roles of PPARs in health and disease. Nature. 2000. 405:421–424.


15. Desvergne B, Wahli W. Peroxisome proliferator-activated receptors: nuclear control of metabolism. Endocr Rev. 1999. 20:649–688.


16. Luquet S, Gaudel C, Holst D, Lopez-Soriano J, Jehl-Pietri C, Fredenrich A, Grimaldi PA. Roles of PPARδ in lipid absorption and metabolism: a new target for the treatment of type 2 diabetes. Biochim Biophys Acta. 2005. 1740:313–317.


17. Jehl-Pietri C, Bastie C, Gillot I, Luquet S, Grimaldi PA. Peroxisome-proliferator-activated receptor δ mediates the effects of long-chain fatty acids on post-confluent cell proliferation. Biochem J. 2000. 350:93–98.


18. Wang YX, Lee CH, Tiep S, Yu RT, Ham J, Kang H, Evans RM. Peroxisome-proliferator-activated receptor δ activates fat metabolism to prevent obesity. Cell. 2003. 113:159–170.


19. Oliver WR Jr, Shenk JL, Snaith MR, Russell CS, Plunket KD, Bodkin NL, Lewis MC, Winegar DA, Sznaidman ML, Lambert MH, Xu HE, Sternbach DD, Kliewer SA, Hansen BC, Willson TM. A selective peroxisome proliferator-activated receptor δ agonist promotes reverse cholesterol transport. Proc Natl Acad Sci U S A. 2001. 98:5306–5311.


20. Tan NS, Michalik L, Noy N, Yasmin R, Pacot C, Heim M, Fluhmann B, Desvergne B, Wahli W. Critical roles of PPAR β/δ in keratinocyte response to inflammation. Genes Dev. 2001. 15:3263–3277.
21. Michalik L, Desvergne B, Tan NS, Basu-Modak S, Escher P, Rieusset J, Peters JM, Kaya G, Gonzalez FJ, Zakany J, Metzger D, Chambon P, Duboule D, Wahli W. Impaired skin wound healing in peroxisome proliferator-activated receptor (PPAR)α and PPARβ mutant mice. J Cell Biol. 2001. 154:799–814.


22. Di-Poi N, Michalik L, Tan NS, Desvergne B, Wahli W. The anti-apoptotic role of PPARβ contributes to efficient skin wound healing. J Steroid Biochem Mol Biol. 2003. 85:257–265.
23. Cheng L, Ding G, Qin Q, Huang Y, Lewis W, He N, Evans RM, Schneider MD, Brako FA, Xiao Y, Chen YE, Yang Q. Cardiomyocyte-restricted peroxisome proliferator-activated receptor-δ deletion perturbs myocardial fatty acid oxidation and leads to cardiomyopathy. Nat Med. 2004. 10:1245–1250.


24. Luquet S, Lopez-Soriano J, Holst D, Fredenrich A, Melki J, Rassoulzadegan M, Grimaldi PA. Peroxisome proliferator-activated receptor δ controls muscle development and oxidative capability. FASEB J. 2003. 17:2299–2301.


25. Wang YX, Zhang CL, Yu RT, Cho HK, Nelson MC, Bayuga-Ocampo CR, Ham J, Kang H, Evans RM. Regulation of muscle fiber type and running endurance by PPAR δ. PLos Biol. 2004. 2:e294.
26. Hollingshead HE, Borland MG, Billin AN, Willson TM, Gonzalez FJ, Peters JM. Ligand activation of peroxisome proliferator-activated receptor-β/δ (PPARβ/δ) and inhibition of cyclooxygenase 2 (COX2) attenuate colon carcino-genesis through independent signaling mechanisms. Carcinogenesis. 2008. 29:169–176.


27. Daikoku T, Tranguch S, Chakrabarty A, Wang D, Khabele D, Orsulic S, Morrow JD, Dubois RN, Dey SK. Extracellular signal-regulated kinase is a target of cyclooxygenase-1-peroxisome proliferator-activated receptor-δ signaling in epithelial ovarian cancer. Cancer Res. 2007. 67:5285–5292.


28. Wang D, Wang H, Guo Y, Ning W, Katkuri S, Wahli W, Desvergne B, Dey SK, DuBois RN. Crosstalk between peroxisome proliferator-activated receptor δ and VEGF stimulates cancer progression. Proc Natl Acad Sci U S A. 2006. 103:19069–19074.


29. Gupta RA, Wang D, Katkuri S, Wang H, Dey SK, DuBois RN. Activation of nuclear hormone receptor peroxisome proliferator-activated receptor-δ accelerates intestinal adenoma growth. Nat Med. 2004. 10:245–247.


30. Gupta RA, Tan J, Krause WF, Geraci MW, Willson TM, Dey SK, DuBois RN. Prostacyclin mediated activation of peroxisome proliferator-activated receptor δ in colorectal cancer. Proc Natl Acad Sci U S A. 2000. 97:13275–13280.


31. Lee CH, Chawla A, Urbiztondo N, Liao D, Boisvert WA, Evans RM, Curtiss LK. Transcriptional repression of atherogenic inflammation: modulation by PPARδ. Science. 2003. 302:453–457.


32. Li AC, Binder CJ, Gutierrez A, Brown KK, Plotkin CR, Pattison JW, Valledor AF, Davis RA, Willson TM, Witztum JL, Palinski W, Glass CK. Differential inhibition of macrophage foam-cell formation and atherosclerosis in mice by PPARα, β/δ, and γ. J Clin Invest. 2004. 114:1564–1576.


33. Liou JY, Lee S, Ghelani D, Matijevic-Aleksic N, Wu KK. Protection of endothelial survival by peroxisome proliferator-activated receptor-δ mediated 14-3-3 upregulation. Arterioscler Thromb Vasc Biol. 2006. 26:1481–1487.


34. Kim YH, Han HJ. High-glucose-induced prostaglandin E2 and peroxisome proliferator-activated receptor δ promote mouse embryonic stem cell proliferation. Stem Cells. 2008. 26:745–755.


35. Glazer RI, Yuan H, Xie Z, Yin Y. PPARγ and PPARδ as modulators of neoplasia and cell fate. PPAR Res. 2008. 247379.
36. Huang JC, Wun WS, Goldsby JS, Wun IC, Noorhasan D, Wu KK. Stimulation of embryo hatching and implantation by prostacyclin and peroxisome proliferator-activated receptor δ activation: implication in IVF. Hum Reprod. 2007. 22:807–814.


37. Lim H, Gupta RA, Ma WG, Paria BC, Moller DE, Morrow JD, DuBois RN, Trzaskos JM, Dey SK. Cyclo-oxygenase-2-derived prostacyclin mediates embryo implantation in the mouse via PPARδ. Genes Dev. 1999. 13:1561–1574.


38. Braissant O, Wahli W. Differential expression of peroxisome proliferator-activated receptor-α, -β, and -γ during rat embryonic development. Endocrinol. 1998. 139:2748–2754.


39. Higa R, González E, Pustovrh MC, White V, apobianco E, Martínez N, Jawerbaum A. PPARδ and its activator PGI2 are reduced in diabetic embryopathy: involvement of PPAR δ activation in lipid metabolic and signalling pathways in rat embryo early organogenesis. Mol Hum Reprod. 2007. 13:103–110.


40. Braissant O, Foufelle F, Scotto C, Dauça M, Wahli W. Differential expression of peroxisome proliferator-activated receptors (PPARs): tissue distribution of PPAR-α,-β, and -γ in the adult rat. Endocrinol. 1996. 137:354–366.


41. Kliewer SA, Forman BM, Blumberg B, Ong ES, Borgmeyer U, Mangelsdorf DJ, Umesono K, Evans RM. Differential expression and activation of a family of murine peroxisome proliferator-activated receptors. Proc Natl Acad Sci U S A. 1994. 91:7355–7359.


42. Forman BM, Chen J, Evans RM. Hypolipidemic drugs, polyunsaturated fatty acids, and eicosanoids are ligands for peroxisome proliferator-activated receptors α and δ. Proc Natl Acad Sci U S A. 1997. 94:4312–4317.


43. Lee CH, Kang K, Mehl IR, Nofsinger R, Alaynick WA, Chong LW, Rosenfeld JM, Evans RM. Peroxisome proliferator-activated receptor δ promotes very low-density lipoprotein-derived fatty acid catabolism in the macrophage. Proc Natl Acad Sci U S A. 2006. 103:2434–2439.


44. Ghosh M, Wang H, Ai Y, Romeo E, Luyendyk JP, Peters JM, Mackman N, Dey SK, Hla T. COX-2 suppresses tissue factor expression via endocannabinoid-directed PPARδ activation. J Exp Med. 2007. 204:2053–2061.


45. Shaw N, Elholm M, Noy N. Retinoic acid is a high affinity selective ligand for the peroxisome proliferator-activated receptorβ/δ. J Biol Chem. 2003. 278:41589–41592.


46. Basséne CE, Suzenet F, Hennuyer N, Staels B, Caignard DH, Dacquet C, Renard P, Guillaumet G. Studies towards the conception of new selective PPARβ/δ ligands. Bioorg Med Chem Lett. 2006. 16:4528–4532.
47. Bishop-Bailey D, Wray J. Peroxisome proliferator-activated receptors: a critical review on endogenous pathways for ligand generation. Prostaglandins Other Lipid Mediat. 2003. 71:1–22.


48. Michalik L, Auwerx J, Berger JP, Chatterjee VK, Glass CK, Gonzalez FJ, Grimaldi PA, Kadowaki T, Lazar MA, O'Rahilly S, Palmer CN, Plutzky J, Reddy JK, Spiegelman BM, Staels B, Wahli W. International union of pharmacology. LXI. Peroxisome proliferator-activated receptors. Pharmacol Rev. 2006. 58:726–741.


49. Sznaidman ML, Haffner CD, Maloney PR, Fivush A, Chao E, Goreham D, Sierra ML, LeGrumelec C, Xu HE, Montana VG, Lambert MH, Willson TM, Oliver WR Jr, Sternbach DD. Novel selective small molecule agonists for peroxisome proliferator-activated receptor δ (PPARδ)-synthesis and biological activity. Bioorg Med Chem Lett. 2003. 13:1517–1521.


50. Barak Y, Liao D, He W, Ong ES, Nelson MC, Olefsky JM, Boland R, Evans RM. Effects of peroxisome proliferator-activated receptor δ on placentation, adiposity, and colorectal cancer. Proc Natl Acad Sci U S A. 2002. 99:303–308.


51. Peters JM, Lee SS, Li W, Ward JM, Gavrilova O, Everett C, Reitman ML, Hudson LD, Gonzalez FJ. Growth, adi-pose, brain, and skin alterations resulting from targeted disruption of the mouse peroxisome proliferator-activated receptor β (δ). Mol Cell Biol. 2000. 20:5119–5128.


52. Kopelovich L, Fay JR, Glazer RI, Crowell JA. Peroxisome proliferator-activated receptor modulators as potential chemopreventive agents. Mol Cancer Ther. 2001. 1:357–363.
53. He TC, Chan TA, Vogelstein B, Kinzler KW. PPARδ is an APC-regulated target of nonsteroidal anti-inflammatory drugs. Cell. 1999. 99:335–345.


54. Zhang J, Fu M, Zhu X, Xiao Y, Mou Y, Zheng H, Akinbami MA, Wang Q, Chen YE. Peroxisome proliferator-activated receptor δ is up-regulated during vascular lesion formation and promotes post-confluent cell proliferation in vascular smooth muscle cells. J Biol Chem. 2002. 277:11505–11512.


55. Hansen JB, Zhang H, Rasmussen TH, Petersen RK, Flindt EN, Kristiansen K. Peroxisome proliferator-activated receptor δ (PPARδ)-mediated regulation of preadipocyte proliferation and gene expression is dependent on cAMP signaling. J Biol Chem. 2001. 276:3175–3182.


56. Xu L, Han C, Wu T. A novel positive feedback loop between peroxisome proliferator-activated receptor-δ and prostaglandin E2 signaling pathways for human cholangiocarcinoma cell growth. J Biol Chem. 2006. 281:33982–33996.


57. Burdick AD, Bility MT, Girroir EE, Billin AN, Willson TM, Gonzalez FJ, Peters JM. Ligand activation of peroxisome proliferator-activated receptor-β/δ (PPARβ/δ) inhibits cell growth of human N/TERT-1 keratinocytes. Cell Signal. 2007. 19:1163–1171.


58. Glinghammar B, Skogsberg J, Hamsten A, Ehrenborg E. PPARδ activation induces COX-2 gene expression and cell proliferation in human hepatocellular carcinoma cells. Biochem Biophys Res Commun. 2003. 308:361–368.


59. Wang D, Wang H, Shi Q, Katkuri S, Walhi W, Desvergne B, Das SK, Dey SK, DuBois RN. Prostaglandin E2 promotes colorectal adenoma growth via transactivation of the nuclear peroxisome proliferator-activated receptor δ. Cancer Cell. 2004. 6:285–295.


60. Wang H, Xie H, Sun X, Tranguch S, Zhang H, Jia X, Wang D, Das SK, Desvergne B, Wahli W, DuBois RN, Dey SK. Stage-specific integration of maternal and embryonic peroxisome proliferator-activated receptor δ signaling is critical to pregnancy success. J Biol chem. 2007. 282:37770–37782.


61. Pakrasi PL, Jain AK. Evaluation of cyclooxygenase 2 derived endogenous prostacyclin in mouse preimplantation embryo development in vitro. Life Sci. 2007. 80:503–1507.


62. Cimini A, Benedetti E, Cristiano L, Sebastiani P, D'Amico MA, D'Angelo B, Di Loreto S. Expression of peroxisome proliferator-activated receptors (PPARs) and retinoic acid receptors (RXRs) in rat cortical neurons. Neuroscience. 2005. 30:325–337.


63. Shi Y, Hon M, Evans RM. The peroxisome proliferators-activated receptor δ an integrator of transcriptional repression and nuclear receptor signaling. Proc Natl Acad Sci U S A. 2002. 99:2613–2618.


64. Cimini A, Cristiano L, Benedetti E, D'Angelo B, Cerú MP. PPARs expression in adult mouse neural stem cells: modulation of PPARs during astroglial differentiaton of NSC. PPAR Res. 2007. 48242.


65. IJpenberg A, Tan NS, Gelman L, Kersten S, Seydoux J, Xu J, Metzger D, Canaple L, Chambon P, Wahli W, Desvergne B. In vivo activation of PPAR target genes by RXR homodimers. EMBO J. 2004. 23:2083–2091.


66. Feige JN, Gelman L, Michalik L, Desvergne B, Wahli W. From molecular action to physiological outputs: peroxisome proliferator-activated receptors are nuclear receptors at the crossroads of key cellular functions. Prog Lipid Res. 2006. 45:120–159.


67. Wang XY, Yin Y, Yuan H, Sakamaki T, Okano H, Glazer RI. Musashi1 modulates mammary progenitor cell expansion through proliferin-mediated activation of the Wnt and Notch pathways. Mol Cell Biol. 2008. 28:3589–3599.


68. Varnat F, Heggeler B, Grisel P, Boucard N, Corthésy-Theulaz I, Wahli W, Desvergne B. PPARβ/δ regulates paneth cell differentiation via controlling the hedgehog signaling pathway. Gastroenterology. 2006. 131:538–553.


69. Pakrasi PL, Jain AK. Cyclooxygenase-2 derived PGE2 and PGI2 play an important role via EP2 and PPARδ receptors in early steps of oil induced decidualization in mice. Placenta. 2008. 29:523–530.


70. Jawerbaum A, Gonzalez ET, Sinner D, Pustovrh D, White V, Gimeno MA. Diminished PGE2 content, enhanced PGE2 release and defects in 3H-PGE2 transport in embryos from overtly diabetic rats. Reprod Fertil Dev. 2000. 12:141–147.


71. Kennedy TG. Evidence for the involvement of prostaglandins throughout decidual cell reaction in the rat. Biol Reprod. 1985. 33:140–146.


72. Tawfik OW, Sagritlo C, Johnson DC, Dey SK. Decidualization in the rat: role of leukotrienes and prostaglandins. Prostaglandins Leukot Med. 1987. 29:221–227.
73. Papay KD, Kennedy TG. Characterizatio of temporal and cell specific changes in transcripts for prostaglandin E(2) receptor in pseudopregnant rat endometrium. Biol Rprod. 2000. 62:1515–1525.


74. Burdon T, Smith A, Savatier P. Signaling, cell cycle and pluripotency in embryonic stem cells. Trends Cell Biol. 2002. 12:432–438.
75. Crackower MA, Oudit GY, Kozieradzki I, Sarao R, Sun H, Sasaki T, Hirsch E, Suzuki A, Shioi T, Irie-Sasaki J, Sah R, Cheng HY, Rybin VO, Lembo G, Fratta L, Oliveirados-Santos AJ, Benovic JL, Kahn CR, Izumo S, Steinberg SF, Wymann MP, Backx PH, Penninger JM. Regulation of myocardial contractility and cell size by distinct PI3KPTEN signaling pathways. Cell. 2002. 110:737–749.


Fig. 1.
The hypothesized model for the signal pathways involved in high glucose-induced mouse ES cell proliferation. High glucose increased PGE2 synthesis, which is controlled by the coupled activation of cPLA2/AA and COX2 via ROS. After PGE2 is released into the extracellular space, it binds to the membrane coupled EP receptors. This effect is mediated, at least in part, by the activation of PI3K/Akt. Finally, these molecules may induce PPARδ, which increases cell cycle regulatory protein expression levels. Abbreviations: AA, arachidonic acid; CDK, cyclin-dependent kinase; COX2, cyclooxygenase-2; cPLA2, cytosolic phospholipase A2; NAC, N-acetylcysteine; PGE2, prostaglandin E2; PI3K, phosphoinositide 3-kinase; PPAR, peroxisome proliferator activated receptor; ROS, reactive oxygen species (34).
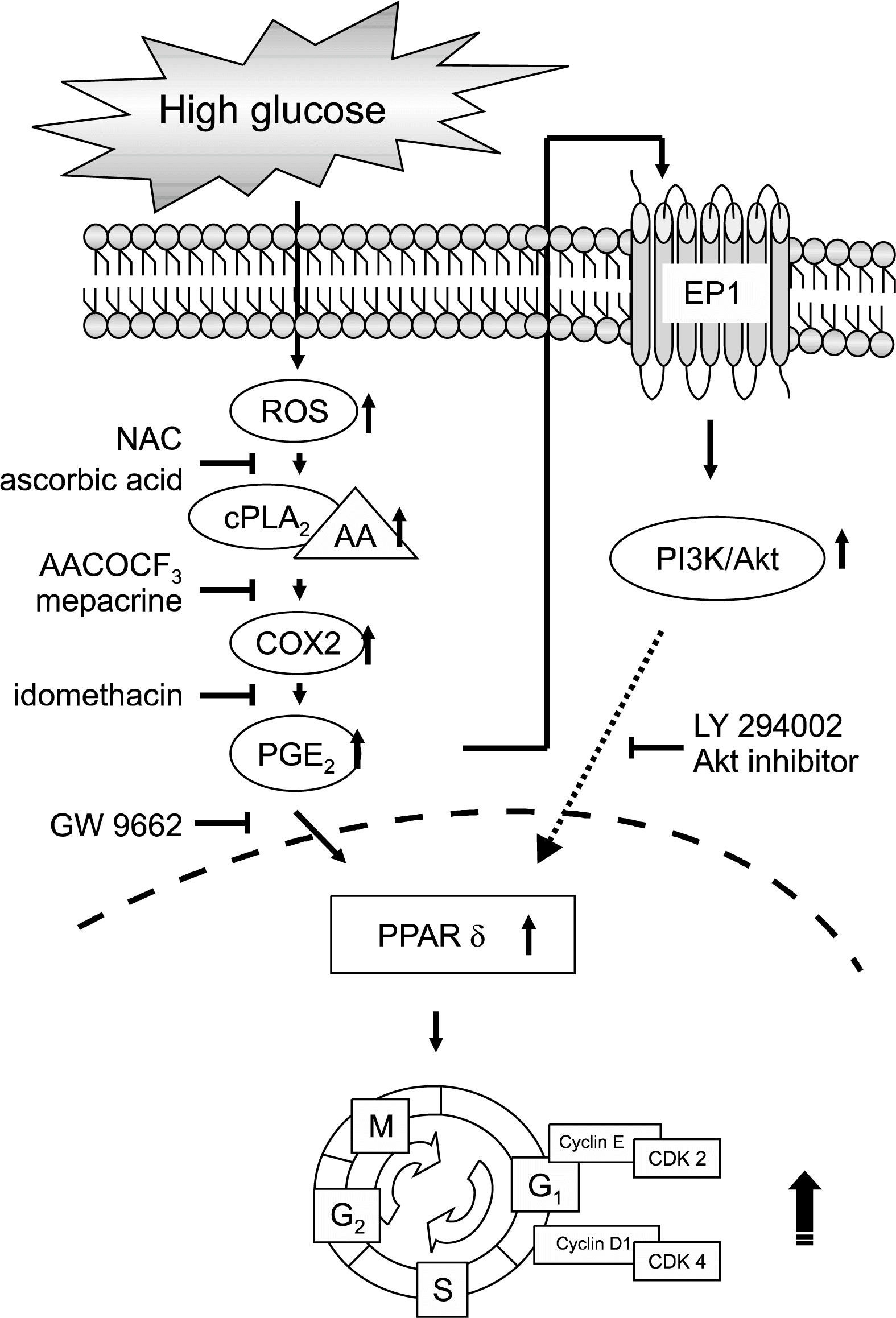