Abstract
Background and Objectives:
Neural stem/precursor cells are found in relatively inaccessible neurogenic regions of the adult brain, making them difficult to harvest for therapeutic purposes.
Methods and Results:
In this study, nestin-expressing spheroids were induced from primary cultures of newborn and adult foreskin-derived dermal fibroblasts using a novel, two-step induction method. Approximately 80% of dermal fibroblasts became nestin-expressing cells within 12 days. Nestin expression and spheroid-forming capacity were both blocked by removal of bFGF from the induction medium. The bFGF-induced, nestin-expressing spheroids possessed most of the features distinctive of skin-derived precursor cells.
Conclusions:
Our findings support the possibility of inducing nestin-expressing spheroids from purified human dermal fibroblasts in a bFGF dependent manner. This is important because it may be impossible to induce spheroids from the small pool of residual neural crest-related precursor cells found in adults.
Neural stem cells are multipotent cells capable of differentiating into neurons, oligodendrocytes, or astrocytes (1). In adult mammals, neural stem cells have been found in the subventricular zone as well as the dentate gyrus of the hippocampus (2). Unfortunately, those regions of the brain are relatively inaccessible, making it difficult to obtain donor cells for use in therapeutic applications.
Several attempts have been made to identify accessible sources of neural stem cells. Hematopoietic stem cells (HSCs), mesenchymal stem cells (MSCs), and dermal cells can differentiate into neuronal and glial cells under certain conditions in vitro (3). Furthermore, multipotent skin-derived precursor cells (SKPs) can be derived from human scalp dermis as well as from murine skin. However, these cells require approximately 3 months of spheroid culture (4–10), which introduces the possibility of epigenetic reprogramming. Recent studies suggest SKPs originate from endogenous, embryonic neural crest related, precursor cells located near the dermal papillae of hair follicles which persist into adulthood as either rudimentary or residual stem cells (11, 12). However, it remains unclear whether SKPs can be directly induced from dermal fibroblasts under specific epigenetic culture conditions, or if they can be obtained via selective expansion of residual neural crest stem cells in the dermis.
To explore these possibilities, human dermal fibroblasts (HDFs) were isolated from newborn and adult foreskin lacking both hair follicles and nestin immunoreactivity. Dermal fibroblasts were obtained after first passage in cell culture and/or purified on anti-fibroblast MACS columns. The dermal fibroblasts were exposed to five different induction conditions using various combinations of serum, bFGF, laminin, and N2 as each of these is important for neuronal induction (13-1)]. The fibroblasts were then assessed for neural precursor-like characteristics on the basis of nestin expression (19–21), spheroid forming capacity, and cell-surface marker expression (22–24).
After obtaining informed consent, HDFs were isolated from newborn or adult foreskin immediately following circumcision performed at either the Cha Hospital or Goodman Clinic in Seoul, Korea. The epidermal sheet was mechanically removed after overnight incubation with dispase II (Roche, Indianapolis, IN), cut into 1 mm2 pieces, and then incubated in an enzyme cocktail consisting of 0.1% collagenase (Worthington, Lakewood, USA), 0.1% hyaluronidase, and 0.075% DNase (Sigma, St. Louis, USA). Isolated HDFs were then cultured in FGM2 medium (Clonetics, San Diego, CA) using 0.1% gelatin-coated culture dishes. When specified, HDFs were purified on MACS columns using anti-fibroblast beads (Miltenyl Biotech, Auburn, CA). Human keratinocytes (MCTT Inc, Seoul, Korea) and MSCs (Clonetics) were cultured according to the manufacturer’s specifications.
After one passage in FGM2 culture medium (Clonetics), HDFs were plated at a density of 10,000 cells/cm2 on poly-D-lysine/laminin(Sigma)-coated cover slips, or in culture dishes at 5×104 cells/ml, in identical medium. Cultures were incubated for one day to allow cell attachment, then transferred to one of five different media (9–14): 1) FGM (Clonetics), 2) DMEM/F12 with 10% FBS (M1 medium), 3) serum-free DMEM/F12 supplemented with N2 ((M2 medium), 4) M2 medium supplemented with 10 ng/ml bFGF (R&D Inc, Minneapolis, MN), 1μg/ ml laminin (Sigma) and 2μg/ml heparin (Sigma, M3 medium) for 12 days, and 5) two-step induction medium (i.e., cells were primed with M2 medium for six days, followed by M3 medium for six days). N2 supplements (Gibco, Grand Island, USA) consisted of 5μg/ml insulin, 10μM progesterone, 10μM putrascine, 10μM sodium selenite, and 100μg/ml transferrin. Long-term spheroid expansion was accomplished via cell culture in bacterial dishes containing M3 medium. Cell morphology and spheroid formation were examined under a phase contrast microscope (Olympus, Japan).
Cells cultured on cover slips were fixed in 3.7% form-aldehyde (Sigma), permeabilized with 0.2% Triton X-100 (USB, Cleveland, OH) for 10 minutes, blocked with 20% normal goat serum (Vector Lab, Burlingame, CA) for 1 hour, incubated with primary antibodies for overnight at 4°C followed by FITC- or Texas Red-conjugated IgG (Vector Lab, 1:100) for 1 hour at room temperature, and then stained with 4, 6-diamidino-2-phenylindole (DAPI) for 10 minutes. Sections were mounted with Vectashield and then examined under a Leica confocal microscope (Leica, Germany). Diaminobenzidine (DAB) staining of cells on culture dishes was performed by blocking endogenous peroxidase activity for 30 minutes in the presence of 0.3% H2O2 (Sigma) in methanol. The staining area was then cleaned with a cotton swab and demarcated using a paraffin pen and permeabilized with 0.2% Triton X-100 for 10 minutes, blocked with 20% normal goat serum for 1 hour, incubated with primary antibodies for overnight at 4°C. Subsequently, the cells were incubated with a biotinylated secondary antibody for 1 hour and an avidin-biotin peroxidase complex (Vector Lab) for 30 minutes. The final reaction for peroxidase was carried out with the DAB peroxidase substrate kit (Vector Lab). Cells were mounted under a coverslip with Aquatex (Merck, Whitehouse Station, NJ) and examined under a light microscope (Olympus). The spheroids staining were accomplished by impregnating the fixed spheroids with neutralized type I collagen (BD, Bedfold, USA) and embedding in a paraffin block. Paraffin sections (4μm) were deparaffinized, rehydrated, and then boiled in 0.01 M sodium citrate. The remainder of the staining procedure was performed as described above. Primary antibodies consisted of monoclonal antibodies against nestin (Chemicon, Temecula, CA, 1: 200), nestin (Biodesign, Saco, ME, 1:200) and β-III tubulin (Sigma, 1:400), vimentin (DAKO, Glostrup, Demark, 1:50), A2B5 (Chemicon, 1:200), O4 (Chemicon, 1:50), GFAP (DAKO, 1:50), NF-68 (Sigma, 1:400), NF-150 (Chemicon, 1:400), MAP2 (Chemicon, 1: 1,000), Tau (abCAM, Cambridge, MA 1:500) and polyclonal antibodies against p75 (Promega, Madison, WI, 1: 500).
SKPs were initially identified based upon nestin expression and sphere-forming capacity in scalp skin after long-term culture. These cells are reported to originate from residual embryonic neural crest-related precursor cells in the adult skin (4–8). Therefore, to determine whether nestin-expressing cells are endogenous to the newborn foreskin, or if they are induced by specific culture environments, formalin-fixed or frozen sections of newborn foreskin or adult scalp were stained with two different antibodies capable of recognizing the nestin protein in neuroblastoma SH-SY5Y cells (15–17). Sections were also stained using antibodies against vimentin as a positive control for HDFs. Nestin-expressing cells were not detected in the dermis of either newborn or adult foreskin (data not shown). However, vimentin-expressing HDFs were clearly observed (Fig. 1A).
To further explore the possibility of epigenetic trans-differentiation among vimentin-expressing HDFs, the cells were isolated, divided into five groups, and cultured as described in Materials and methods. DAPI-positive cells expressed vimentin under all five culture conditions, while nestin expression differed among the groups (Fig. 1B). Nestin-expressing cells were detected with weak intensity in FGM, M1, and M2 media, while their numbers increased in M3 medium. However, cells grown in the two-step induction medium strongly expressed nestin, as shown in Fig. 1C. Approximately 80% of the HDFs cultured in the two-step induction medium became nestin-expressing cells within 12 days. HDFs, which do not express neural precursor markers such as nestin in vivo, rapidly began to express nestin under specific culture conditions (e.g., growth in M3 medium). Absence of nestin-immunoreactivity in foreskin tissue sections, combined with the specific induction of nestin expression in two-step cultures, strongly suggest that nestin-expressing cells are induced by components of the M3 medium. It also suggests that this induction can be strongly stimulated by priming the cultures in serum-free M2 medium.
To further characterize the nestin-expressing cells generated using our two-step induction method, cells were stained via double-immunofluorescence labeling using antibodies against either nestin and fibronectin, or nestin and β-III tubulin (Fig. 1D). Following two-step induction, nestin-expressing cells strongly expressed FN and β-III tubulin, both of which are markers for immature neuronal cells. Therefore, two-step induction of HDFs stimulated the expression of markers specific for neural pre-cursor cells, as well as immature neurons such as nestin, fibronectin, and β-III tubulin.
To determine if nestin-expressing cells are capable of forming neurospheroid-like structures, cells primed in M2 medium were re-plated on poly-D-lysine/laminin-coated dishes in M3 medium (Fig. 2A). Spheroids did not form in M2 medium. However, cells exposed to M3 medium began to converge centrally after three days and formed spheroids after six days. Spheroids continued to grow for up to 40 days and either floated in suspension, or adhered to the bacterial culture dishes (Fig. 2B). Split spheroids reformed to generate spheroids (data not shown).
To characterize dermal cells that either attached to the surface of the culture dish or floated as spheroids, cells were fixed and stained using antibodies against nestin and vimentin (neuronal precursor cells), β-III tubulin (immature neuron cells), A2B5 (oligodendrocyte progenitor cells), p75 (neural crest stem cells), O4 (pro-oligodendrocytes), GFAP (astrocytes), and NF-68, NF-150, MAP2, and Tau (mature neurons) (Fig. 3A, B). Both attached cells and spheroids expressed p75, β-III tubulin, and A2B5, but did not express NF-68, GFAP, or O4. Therefore, spheroids induced via the two-step method satisfied most of the previously reported characteristics of SKPs (4–6).
To identify which factors in M3 medium are responsible for nestin and spheroid formation, cells were incubated in M3, both with and without bFGF or laminin, after priming the cultures in serum-free M2 medium. Following incubation, spheroid formation and nestin expression were examined (Fig. 4A, B). Spheroids were successfully formed in M3 medium supplemented with bFGF, regardless of the presence of laminin. However, spheroids were not formed in the absence of bFGF, even when laminin was supplied (Fig. 4A). Similarly, nestin expression also required bFGF (Fig. 4B). Therefore, these results suggest that bFGF induces nestin and spheroid formation in HDFs.
Purified HDFs can be induced to form spheroids that express markers for immature neurons and neuronal precursor cells by first priming in serum- and bFGF-free, followed by incubation in bFGF-containing medium. Previous reports suggest that MSCs have the potential to transdifferentiate into neuronal types capable of aiding in recovery after injury to the central nervous system (3). Therefore, we used our two-step induction method on both human MSCs and keratinocytes. This method did not induce nestin expression or spheroid formation in either of these two primary cultured cell types (Fig. 5). Thus, two-step induction of nestin-expressing spheroids seems possible only for HDFs in the presence of bFGF.
We have developed a novel and efficient method for inducing purified primary HDFs. Neural induction was evaluated by analyzing the expression of nestin, β-III tubulin, FN, and vimentin as markers for neural precursors, immature neurons, and spheroid-forming capacity, respectively. Our method of neural induction resulted in the conversion of approximately 80% of the HDFs into nestin-expressing cells within 12 days. Such high efficiency was obtained by first priming the fibroblasts (i.e., incubating the cells in an N2-supplemented medium in the absence of serum and growth factors), followed by incubation in a bFGF-containing M3 medium. Our two-step process used HDFs from either newborn or adult foreskin (data not shown) that had been purified after a single passage in cell culture medium. These data strongly suggest that neurospheroids can be generated from HDFs without the risk of epigenetic reprogramming, such as is encountered during long-term induction protocols.
Induction of neurospheroid formation requires the presence of bFGF in M3 medium and was specifically blocked by its removal. Furthermore, priming cells in M2 medium enhanced bFGF-stimulated nestin expression and increased induction by at least two-fold. The mechanisms underlying this phenomenon are not clearly understood, but our data suggest that bFGF is more effective on starved, rather than on actively proliferating cells. Future studies might test this hypothesis by adding cell proliferation-inhibiting agents to the two-step induction procedure.
Identification of SKPs following long-term culture of scalp-derived spheroids [4–6] has introduced the possibility of genetic reprogramming in the original dermis-derived cells. Recent reports (7, 8) suggest that SKPs originate from the selective expansion of residual neural crest stem cells and are present in low numbers in the dermis. However, our data suggest that selective expansion of residual neural crest cells does not occur during the two-step induction period. Conversely, 80% of fibroblast cells expressed nestin and generated spheroids following two-step induction. This supports the notion that dermal fibro-blast-derived neurospheroids are generated by the specific actions of bFGF, rather than by expansion of residual neural crest stem cells. While we were unable to induce MSCs and keratinocytes, we found that HDFs responded efficiently to bFGF-dependent induction. Therefore, HDFs purified via anti-fibroblast beads, which constitute the major population of cells isolated from the dermis, seem to retain an inherent capacity for transdifferentiation and are more plastic than previously expected. Overall, our findings reveal that neurospheroids can be induced by a short-term, two-step process and are an accessible source of stem cells for neuronal or glial cell therapies.
ACKNOWLEDGMENTS
This research was supported by grant SC3120 to Dr. Youngsook Son from the Stem Cell Research Center of the 21st Century Frontier Research Program, funded by the Ministry of Science and Technology Republic of Korea.
References
1. Alvarez-Buylla A, Temple S. Stem cells in the developing and adult nervous system. J Neurobiol. 1998. 36:105–110.


2. Song H, Stevens CF, Gage FH. Astroglia induce neurogenesis from adult neural stem cells. Nature. 2002. 417:39–44.


5. Toma JG, Akhavan M, Fernandes KJ, Barnabé-Heider F, Sadikot A, Kaplan DR, Miller FD. Isolation of multipotent adult stem cells from the dermis of mammalian skin. Nat Cell Biol. 2001. 3:778–784.


6. Toma JG, McKenzie IA, Bagli D, Miller FD. Isolation and characterization of multipotent skin-derived precursors from human skin. Stem Cells. 2005. 23:727–737.


7. Joannides A, Gaughwin P, Schwiening C, Majed H, Sterling J, Compston A, Chandran S. Efficient generation of neural precursors from adult human skin: astrocytes promote neurogenesis from skin-derived stem cells. Lancet. 2004. 364:172–178.


8. Mckenzie IA, Biernaskie J, Toma JG, Midha R, Miller FD. Skin-derived precursors generate myelinating schwann cells for the injured and dysmyelinated nervous system. J Neurosci. 2006. 26:6651–6660.


9. Shin DT, Lee DC, Chen SC, Tsai RY, Huang CT, Tsai CC, Shen EY, Chiu WT. Isolation and characterization of neurogenic MSCs in human scalp tissue. Stem cells. 2005. 23:1012–1020.


10. Fernandes KJ, Kobayashi NR, Gallagher CJ, Barnabé-Heider F, Aumont A, Kaplan DR, Miller FD. Analysis of the neurogenic potential of multipotent skin-derived precursors. Exp Neurol. 2006. 201:32–48.


11. Fernandes KJ, McKenzie IA, Mill P, Smith KM, Akhavan M, Barnabé-Haider F, Biernaskie J, Junek A, Kobayashi NR, Toma JG, Keplan DR, Labosky PA, Rafuse V, Hui CC, Miller FD. A dermal niche for multipotent adult skin-derived precursor cells. Nat Cell Biol. 2004. 6:1082–1093.


12. Wong CE, Paratore C, Dours-Zimmermann MT, Rochat A, Pietri T, Suter U, Zimmermann DR, Dufour S, Thiery JP, Meijer D, Beermann F, Barrandon Y, Sommer L. Neural crest-derived cells with stem cell features can be traced back to multiple lineages in the adult skin. J Cell Biol. 2006. 175:1005–1015.


13. Bottenstein JE, Harvey AL. Cell culture in the neuro-sciences. 3rd edition. New York and London: Plenum Press;1985.
14. Zhang SC, Wernig M, Duncan ID, Brüstle O, Thomson JA. In vitro differentiation of transplantable neural precursors from human embryonic stem cells. Nat Biotechnology. 2001. 19:1129–1133.


15. Tarasenko YI, Yu Y, Jordan PM, Bottenstein J, Wu P. Effect of growth factors on proliferation and phenotypic differentiation of human fetal neural stem cells. J Neurosci Res. 2004. 78:625–636.


16. Eriksson C, Björklund A, Wictorin K. Neuronal differentiation following transplantation of expanded mouse neurosphere cultures derived from different embryonic fore-brain regions. Exp Neurol. 2003. 184:615–635.


17. Lee SH, Lumelsky N, Studer L, Auerbach JM, McKay RD. Efficient generation of midbrain and hindbrain neurons from mouse embryonic stem cells. Nat Biotechnol. 2000. 18:675–679.


18. Kelly CM, Zietlow R, Dunnett SB, Rosser AE. The effects of various concentrations of FGF-2 on the proliferation and neuronal yield of murine embryonic neural precursor cells in vitro. Cell Transplant. 2003. 12:215–223.


19. Messam CA, Hou J, Major EO. Coexpression of nestin in neural and glial cells in the developing human CNS defined by a human-specific anti-nestin antibody. Exp Neurol. 2000. 161:585–596.


20. Tohyama T, Lee VM, Rorke LB, Marvin M, McKay RD, Trojanowski JQ. Nestin expression in embryonic human neuroepithelium and in human neuroepithelial tumor cells. Lab Invest. 1992. 66:303–313.
21. Dahlstrand J, Lardelli M, Lendahl U. Nestin mRNA expression correlates with the central nervous system progenitor cell state in many, but not all, regions of developing central nervous system. Dev Brain Res. 1995. 84:109–129.


22. Bronner-Fraser M. Origins developmental potential of the neural crest. Exp Cell Res. 1995. 218:405–417. (NCSC).
Fig. 1.
Induction of nestin, FN and β-III tubulin in HDFs by a novel two-step process. (A) Endogenous expression of nestin and vimentin in a formalin-fixed paraffin section of human newborn foreskin and a frozen section of human scalp tissue. Immunohistochemical staining for vimentin and nestin in paraffin sections of human foreskin developed by DAB and counterstained with Fast Red. Immunofluorescence staining against vimentin and nestin in a frozen section of human scalp was detected with FITC-conjugated secondary antibodies and counter-stained with DAPI. Two different nestin (nestin (1), Chemicon; nestin (2), Biodesign) antibodies were used. Brown-staining in the basal layer of the epidermis was due to melanin deposition, as shown in the control sample. (B) Expression of nestin and vimentin in HDFs derived from newborn foreskin. The cells were cultured under different conditions as described in materials and method. After acetone/ethanol fixation, double immunofluorescence staining with anti-nestin and anti-vimentin were performed, followed by DAPI counterstaining. Nestin-negative but vimentin-positive cells are noted by arrows. (C) Statistical comparison of total nestin-expressing versus DAPI-positive cells. Results are expressed as means±S.D, for three independent experiments. Cells cultured in M2/M3 showed significantly higher rates of nestin expression compared to other groups (P<0.05). (D) Double immunofluorescence staining of nestin-expressing cells obtained via two-step induction, showing expression of fibronectin and β-III tubulin. The cells were cultured under two-step induction condition and double immunofluorescence staining with anti-nestin and anti-fibronectin antibodies or anti-nestin and anti β-III tubulin were performed. Scale bar A 400 μm, B and D 100 μm.
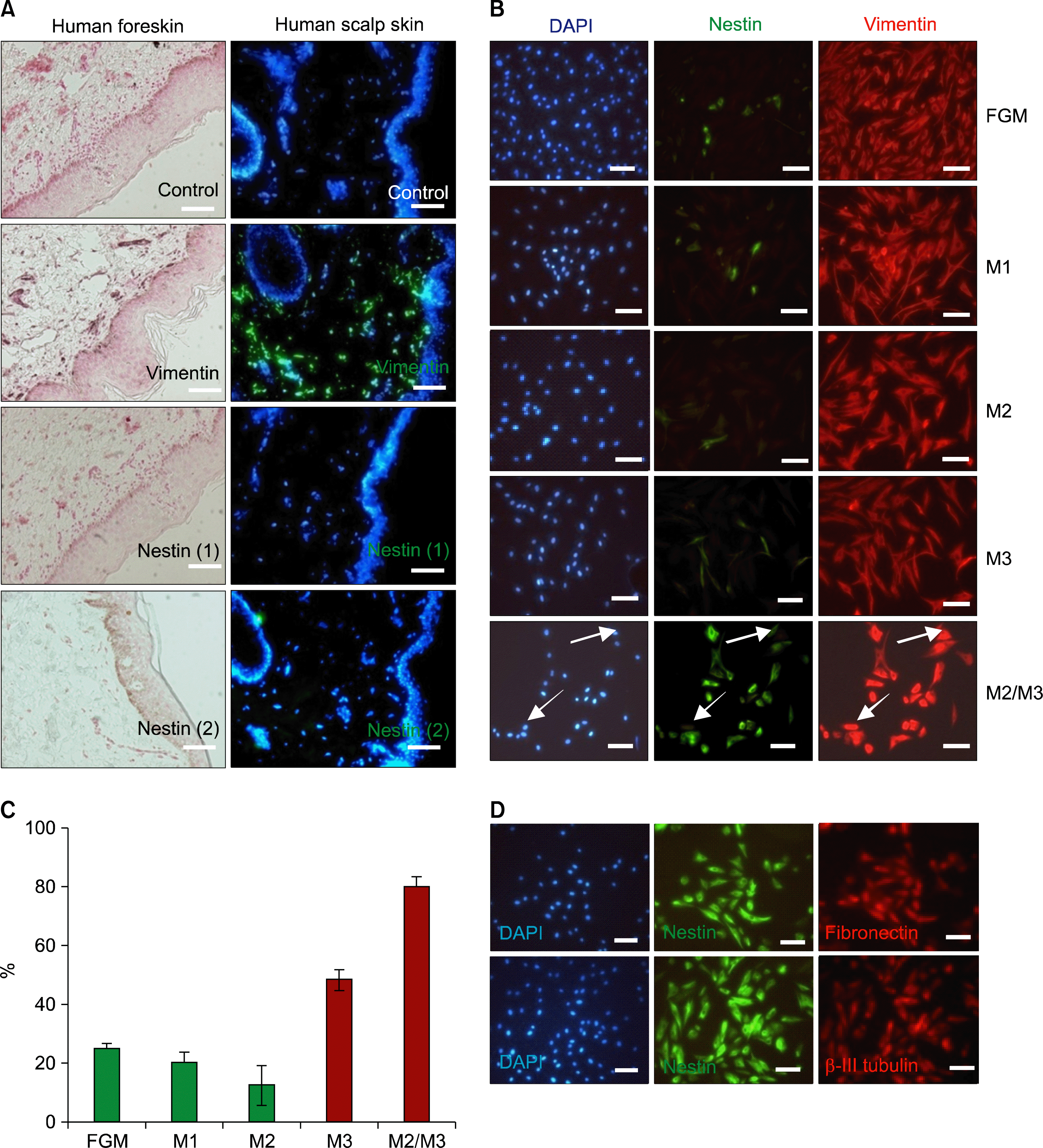
Fig. 2.
Specific induction of spheroids during incubation in M3 medium containing bFGF and laminin (LN), after priming in serum-free M2 medium. (A) Comparison of spheroid-forming capacities in M2 versus M3 media. HDFs did not form spheroids in M2 medium, but were induced to form spheroids within six days after transfer to M3 medium. Centripetal upward attraction of the cell sheet (arrow), which is an early sign of spheroid formation, is noted by an arrow. Obvious spheroid (arrows) formation was discernible at 6 days. (B) Long-term maintenance of spheroid-forming capacity. Spheroids were split into small clumps or single cells that reformed as spheroids(arrows) for up to eight weeks. Scale bar A 400 μm.
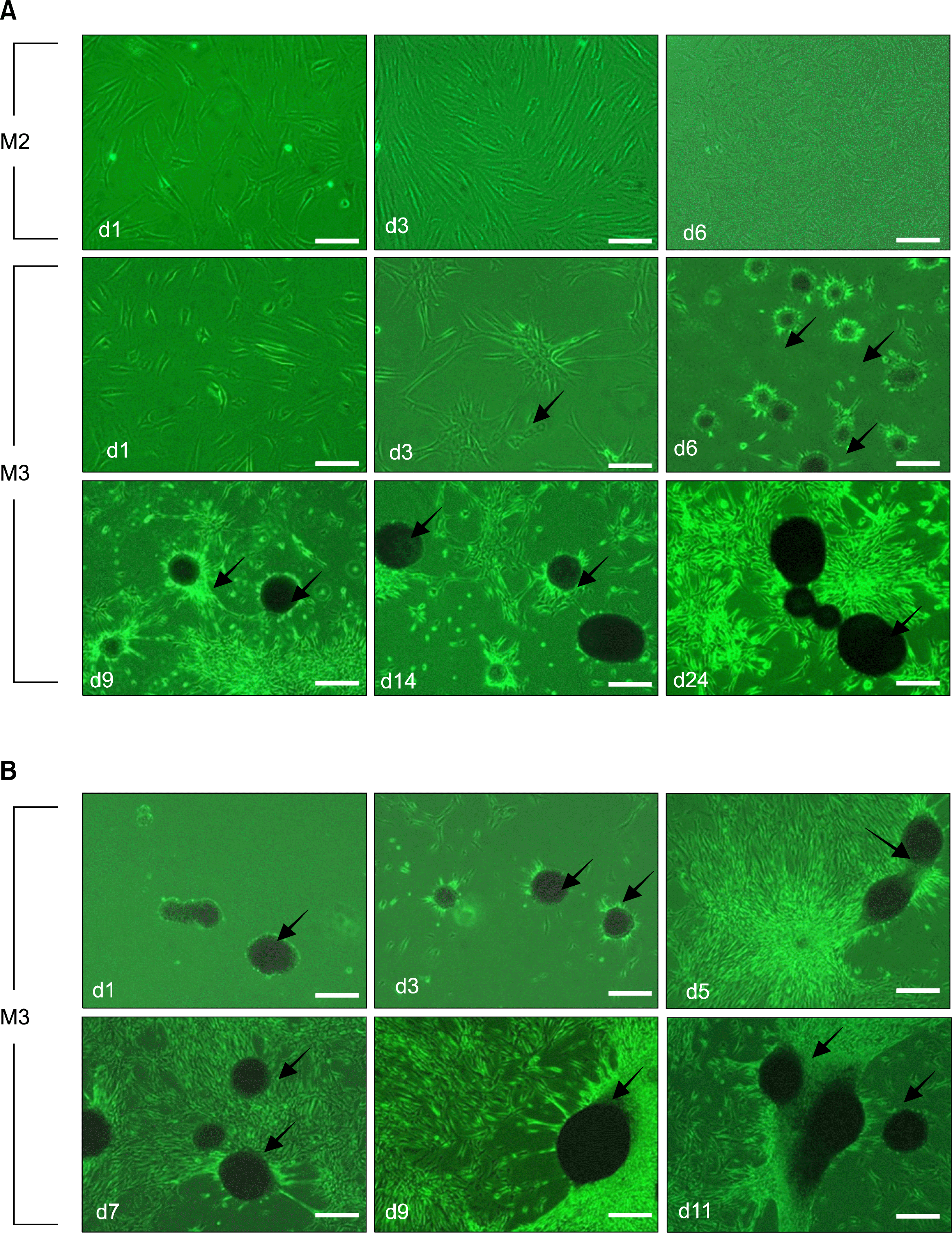
Fig. 3.
Characterization of nestin-expressing and spheroid-forming cells after long term culture. (A) Immunocytochemical staining of nestin-expressing spheroid-forming cells with anti-nestin, anti-vimentin, anti- β-III tubulin, anti-A2B5, anti-p75, anti-O4, anti-MAP2, anti-Tau, anti-NF-150, anti-NF-68, and anti-GFAP antibodies after 40 day spheroid culture. (B) Immunohistochemical staining of spheroids with anti-A2B5, anti-p75, anti-O4, anti-NF-68, and anti-GFAP antibodies. Scale bar A 100 μm, B and D 200 μm.
