Abstract
Neural stem cells (NSCs) transplantation has been studied as a promising tool for replacing damaged neurons in various neurological disorders. However, recent growing data showed new therapeutic benefits of NSCs, which is that transplanted NSCs can modulate cerebral inflammation and protect the brain from further degeneration. We review recent discoveries regarding to the anti-inflammatory effects of NSCs and their future perspectives.
Transplantation of neural stem cells (NSCs) is a promising tool for regeneration of damaged brain in various neurological disorders (1). Usually, NSC has been investigated as a means to replace the lost neurons and reconstitute the lost brain tissue. Thus, researchers have been eager to find methods to transplant NSCs efficiently into the brain and to differentiate them into functional neurons. In a number of studies, however, NSC transplantation rarely results in significant number of terminally differentiated neurons while the transplantation induces significant functional recovery of the animal models. This raises the suspicion that the beneficial effect of NSCs in disease models may be also attributable to alternative biologic properties, which is that transplanted NSCs may attenuate deleterious inflammation, protect the central nervous system from degeneration, and enhance endogenous recovery processes (Fig. 1) (2).
Several researchers have proposed that NSC transplantation may protect the central nervous system from inflammatory damage via a indirect mechanism rather than by direct cell replacement (3). NSCs rescue degenerating neurons by modulating the host environment by adopting a chaperone-like role (4, 5), exert immune-like functions to induce the apoptosis of encephalitogenic T cells (6, 7), and suppress peripheral adaptive immune responses (8).
As summarized in Table 1, the main story of an anti-inflammatory effect of neural progenitor cells (NPCs) came from experimental autoimmune encephalitis (EAE) models. In 2003, researchers from Milano have shown that intraventricular or intravenous transplantation of NPCs reduced brain inflammation and clinical disease severity of EAE model (6). Similar results were also reproduced by different research groups (9). Because clinical signs in this model result from disseminated brain inflammation, it was suggested that NPC transplantation have an anti-inflammatory effect. The amelioration of brain inflammation in NSCs-transplanted animals reduced demyelination and axonal loss. In 2005, the same group published more convincing data about an immunomodulatory effect by which NPCs promote apoptosis of type 1 T-helper cells, shifting the inflammatory process in the brain toward a more favorable climate of dominant type 2 T-helper cells (7). Similarly, a nonspecific immunosuppressive effect of NPCs on T-cell activation and proliferation has been also suggested (8). The intravenously-transplanted NPCs were transiently found in peripheral lymphoid organs, where they interacted with T cells to reduce their encephalitogenicity (8). These studies suggested that the beneficial effects of intravenous NPC injection in EAE models were mediated by a peripheral immunosuppressive mechanism, thus reducing immune cell infiltration into the CNS. These studies also confirmed the direct interactions of lymphocytes and NPCs in vivo and in vitro. NPCs inhibited the activation and proliferations of EAE-derived or naive T-cells. The interaction of NSCs and T cell involves specific receptors. Isolated NSCs from adult brain express CD80 and CD86 (B7-2), the co-stimulatory molecules for T cells (10). CD80 and CD86 expressed by NSCs are functional and can co-stimulate allogenic cells in a mixed lymphocyte reaction (10).
We can observe similar anti-inflammatory actions at mesenchymal stem cells (MSCs) in EAE models. MSC administration before disease onset also ameliorated EAE, decreased inflammatory infiltration and demyelination (11). MSCs inhibited T-cell response to myelin oligoglycoprotein (MOG) and mitogens (11). Similarly to the distribution of NSCs, those intravenously-transplanted MSCs were largely detected in the lymphoid organs of treated mice (11). These data suggest that the immunoregulatory properties of MSCs also occur within secondary lymphoid organs. In another study, MSCs reduced the encephalitogenic properties of T cells (12). MSCs reduced disease progression, cytotoxic cytokine production, and T-cell adoptive transfer-induced disease (12). However, unlike neurosphere cells, MSCs entered into CNS and migrated to the lesion areas. However, no MSCs differentiated into neural cells, suggesting that the reduced disease burden was not a consequence of trans-differentiation and direct repair by the injected MSCs (12).
Recently, human embryonic stem cell (ESC)-derived neural precursors also showed similar anti-inflammatory effects (13). In this study, the transplanted hESC-derived neural precursors reduced clinical signs of EAE, and migrated to the host white matter. However, their differentiation to mature oligodendrocytes and remyelination were negligible. They observed an attenuation of the inflammatory process in transplanted animals. The hESC-derived NPCs also inhibited activation and proliferation of lymph node-derived T cells. In this study, the therapeutic effect of transplantation was not related to graft itself or host remyelination but was mediated by an immunosuppressive neuroprotective mechanism, suggesting the importance of indirect role.
Thus, the anti-inflammatory mechanism of NSCs is supported by these major researches and is becoming important considerations in applications of transplantation therapy for the immune-mediated diseases, because it can protect the host CNS and grafts itself from additional immune attacks.
Inflammation is a major contributor to the post-stroke brain damage. For example, intracerebral hemorrhage (ICH) induces neurologic damage initially by local tissue deformation and followed by the subsequent developments of excitotoxicity, apoptosis, and inflammation (14). Of these, ICH-induced inflammation is a key factor of secondary brain damage, and thus, anti-inflammatory approaches can lessen the outcome of hemorrhagic stroke (14–19). During post-stroke inflammation, both innate and adaptive inflammatory mechanisms participate in the progression of disease (19). The innate responses are the activation of resident brain microglia and the inflammatory infiltration (neutrophils and macrophage) into brain (20). The adaptive inflammation after stroke is mediated by massive and rapid activation of the peripheral immune system, and dynamic and widespread activation of inflammatory cytokines and chemokines in immune organs, such as spleen (21). The spleen participates in the bursting of systemic inflammation by activating tumor necrosis factor-alpha (TNF-α) and Nuclear Factor-kappaB (NF-κ B) (22, 23). TNF-α is one of the major inflammatory mediators in stroke (24), and is largely produced by infiltrating macrophages (25).
In our study, we discovered that NSCs can modulate cerebral and peripheral inflammatory responses in acute stroke (26). We investigated the effect of systemic NSC transplantation on acute cerebral and peripheral inflammation after experimental intracerebral hemorrhage. NSCs from fetal human brain were injected intravenously (5 million cells) at 2 hours after ICH in a rat model. The transplanted NSCs reduced initial neurologic deteriorations, brain edema formation, microglial activation and cellular apoptosis. Human NSCs also attenuated cerebral activations of TNF-α, interleukin-6 (IL-6), and NF-κ B. However, we observed only a few stem cells in brain sections of transplanted animals. While the injected NSCs were mainly detected in marginal zone of spleens. In contrast, intracerebroventricular injection of NSCs was not effective to reduce the acute brain injury. Thus, the anti-inflammatory effect of NSCs needs systemic transplantation. Thus, to investigate whether NSCs interact with systemic immune or inflammatory organs to reduce cerebral inflammation, we screened inflammatory cytokine levels in spleen and lymph nodes, and found that NSCs attenuated the splenic inflammatory activations including TNF-α, IL-6, and NF-κ B. NSCs also decreased the number of TNF-α-expressing macrophages in spleen which were increased by ICH. Thus, we performed splenectomy prior to ICH induction, which eliminated the effect of systemic NSCs transplantation on cerebral inflammation to verify the importance of NSCs-spleen interaction. To confirm the anti-inflammatory properties of NSCs, we performed several in vitro studies, and found that NSCs inhibited macrophage activations after lipopolysaccharide stimulation. Our results suggest that intravenous NSC administration can attenuate systemic inflammatory response after hemorrhagic stroke and protect the brain via a indirect mechanism rather than via any direct cell replacement.
In the above mentioned study, we unexpectedly observed that spleen participates in cerebral inflammation as splenectomy itself reduced cerebral edema and inflammatory cell counts. Spleen is important both as an inducer of cerebral inflammation and as a target for anti-inflammatory intervention in stroke (21, 26), and the connection between brain and spleen inflammation has been referred to as “brain-spleen inflammatory coupling” (26). The communication between the brain and systemic immune responses is facilitated by humoral pathways, and cytokines such as TNF-α, IL-1β, and HMGB1 are known to link inflammatory responses between different cells and tissues (27). It is also known that changes in the peripheral immune system can occur following brain injury and can cause stroke-induced immune depression syndrome or increase the risk of infection (28). The splenic marginal zone is an important transit area for the transplanted cells that are floating in the bloodstream and entering the white pulp. Moreover, based on spleen structure, most of the blood flow goes through the marginal zone and directly along the white pulp, which enables efficient monitoring of the blood by the peripheral immune system (29). Macrophages represent a major component of the marginal zone and express pattern-recognition receptors (e.g., toll-like receptors) that are utilized for pathogen and antigen clearance (29). Thus, early splenic inflammatory activation after stroke can be a therapeutic target for the systemically injected NSCs (Fig. 2).
Similar neuroprotection of NSCs in stroke was suggested in an ischemic model (30). In this study, NSCs injected into the lateral ventricle at 4 hours after the ischemia migrated from the ventricle to the parenchyma, but progressively decreased in number. However, NSCs injection increased the mRNA expressions of trophic factors such as insulin growth factor-1, vascular endothelial growth factor, transforming growth factor-beta1, brain derived neurotrophic factor and stromal derived factor-1alpha (SDF-1α) at 24 h after NSC infusion. As the interaction between NSC and the ischemic environment is important for the protective actions of NSCs, the indirect control of the ischemic environment was the mechanism used by NSC to restore acutely injured brain function (30).
The parenchymal migration of NSCs to damaged site is dependent on SDF-1α-CXC chemokine receptor 4 signaling (31). However, the migration of NSCs to the inflamed tissues is mediated by integrins and chemokine receptors, and includes non-neuronal cell interaction (31). When NPCs from chemokine receptor CCR2 knock-out mice were transplanted, they exhibited little migration toward sites of inflammation (32). Similarly, wild-type NPCs exhibited little migration toward inflammatory sites when transplanted into tissue prepared from monocyte chemoattractant protein-1 (MCP-1/CCL2) knock-out mice (32). These data indicate that factors secreted by sites of neuroinflammation are attractive to neural progenitors and suggest that chemokines such as MCP-1 play an important role in this homing process (32).
The migration process starts from the endothelium-NSCs interaction (3). The rolling of extravasating cells on endothelium is the first essential step in the homing cascade and determines cell adhesion and trans-migration (33). The interactions between hNSCs and TNF-α-stimulated human endothelium (simulating an inflamed milieu) are mediated by a subclass of integrins, such as α2, α6, and β1, but not α4, αv, or the chemokine-mediated pathway CXCR4-SDF-1α. Thus, the mechanisms mediating hNSCs homing via the vascular endothelium differ from the mechanisms mediating homing through parenchymal migration.
The subventricular zone (SVZ) has great potential for neurogenesis, both in rodents and humans, and contains three major cell types (A=neuroblast that migrates to ol-factory bulb, B=neural stem cells that display an astrocyte-like phenotype, C=intermediate transit amplifying progenitors) (3). The cell lineage differentiation pathway proceeds from type B, through type C, to type A cells, with the type B cell (34). Although the relative contribution of the different cell types of the SVZ germinal niche to tissue repair upon CNS injury is still a matter of debate, it is clear that there is a boost of neurogenesis and induced migration of NSCs to the inflamed sites following acute inflammatory insults, such as ischemic stroke (35). However, there is contradictory evidence on the response of the endogenous brain stem cell compartment to chronic CNS inflammation.
Recent evidence by Pluchino et al., shows that persistent brain inflammation, induced by immune cells targeting myelin, extensively alters the proliferative and migratory properties of SVZ-resident NPCs in vivo leading to significant accumulation of non-migratory neuroblasts within the SVZ germinal niche (36). They demonstrated a quantitative reduction of the putative brain stem cells proliferation in the SVZ during persistent brain inflammation, which is completely reversed after in vitro culture of the isolated NPCs. The inflamed brain micro-environment seems to induce a non cell-autonomous dysfunction of the endogenous CNS stem cell compartment, which challenges the potential efficacy of proposed therapies aimed at mobilizing endogenous precursors in chronic inflammatory brain disorders.
As the issue of immune rejection after xenotransplantation is a long time issue among the stem cell researchers, the immunosuppressive effect of NSCs can be confused to be related with the immune rejection of transplanted NSCs. However, if the anti-inflammatory effect of NSCs in ICH models was from some kinds of rejection-mediated effects, splenectomy could not have ameliorated the anti-inflammatory effects because splenectomized rats are still immune competent (26). In addition, NSCs could not have inhibited the in vitro macrophage activation in the culture condition without T and B cells (26). Rodent neurosphere-forming cells express low levels of MHC class I, and co-stimulatory molecules CD80/86 interacting with T cells (10). Human NSCs express MHC class I molecules in a small proportion of them, but do not express MHC class II (37). Sometimes, human NSCs express small proportion of HLA-DQ, but no HLA-DR (38). Thus, the marginal expressions of MHC class I/II prevent NSCs from to be killed by T and NK cells (39). Without immunosuppression, human NSCs are not totally rejected from the rodent brain and thus can still survive (40). In contrast, allogenic MSC are completely rejected from the rat brain in 2 weeks (41). Similar examples are the results from astrocyte transplantations. When transplanted, MSCs and fibroblasts elicited inflammation and were rapidly rejected, whereas astrocytes demonstrated robust survival in the absence of inflammation (42). Accordingly, NSCs have the immunosuppressive effect and low immunogenicity, and are somewhat resistant to the rejection phenomenon, which are unique features of NSCs compared to the other stem cells.
The merits of NSC transplantation come from the hope that damaged or diseased tissues can be regenerated. In this point of view, someone can ask why we should focus the indirect effect of NSCs, such as anti-inflammatory activity, while there exists a number of anti-inflammatory drugs and neurotrophic factor injections. There exist two reasons for this; 1) we can have deep insights about the mechanism how NSCs attenuate the neurologic damages in CNS injuries. Thus, we can have unbiased view about the conventional regenerative potential of NSCs when we encounter enhanced neurologic recoveries. 2) We can design the optimal protocol for clinical NSC transplantations. To maximize the therapeutic potential of NSCs, considerations about the interaction between immune cells (inflammatory cells) and NSCs are crucial aspects not only in inflammatory CNS diseases such as multiple sclerosis, but also in stroke and spinal cord injury. In addition, the indirect role of NSCs may have therapeutic implications in ongoing neurodegenerative diseases. Before clinical applications, we should know how transplanted NSCs shall behave in our body as much as we can.
ACKNOWLEDGMENTS
This work was supported by a grant (SC4120) from the Stem Cell Research Center of the 21st Century Frontier Research Program funded by the Ministry of Science and Technology, South Korea.
References
2. Einstein O, Ben-Hur T. The changing face of neural stem cell therapy in neurologic diseases. Arch Neurol. 2008. 65:452–456.


3. Martino G, Pluchino S. The therapeutic potential of neural stem cells. Nat Rev Neurosci. 2006. 7:395–406.


4. Ourednik J, Ourednik V, Lynch WP, Schachner M, Snyder EY. Neural stem cells display an inherent mechanism for rescuing dysfunctional neurons. Nat Biotechnol. 2002. 20:1103–1110.


5. Hagan M, Wennersten A, Meijer X, Holmin S, Wahlberg L, Mathiesen T. Neuroprotection by human neural progenitor cells after experimental contusion in rats. Neurosci Lett. 2003. 351:149–152.


6. Pluchino S, Quattrini A, Brambilla E, Gritti A, Salani G, Dina G, Galli R, Del Carro U, Amadio S, Bergami A, Furlan R, Comi G, Vescovi AL, Martino G. Injection of adult neurospheres induces recovery in a chronic model of multiple sclerosis. Nature. 2003. 422:688–694.


7. Pluchino S, Zanotti L, Rossi B, Brambilla E, Ottoboni L, Salani G, Martinello M, Cattalini A, Bergami A, Furlan R, Comi G, Constantin G, Martino G. Neurosphere-derived multipotent precursors promote neuroprotection by an immunomodulatory mechanism. Nature. 2005. 436:266–271.


8. Einstein O, Fainstein N, Vaknin I, Mizrachi-Kol R, Reihartz E, Grigoriadis N, Lavon I, Baniyash M, Lassmann H, Ben-Hur T. Neural precursors attenuate autoimmune encephalomyelitis by peripheral immunosuppression. Ann Neurol. 2007. 61:209–218.


9. Einstein O, Karussis D, Grigoriadis N, Mizrachi-Kol R, Reinhartz E, Abramsky O, Ben-Hur T. Intraventricular transplantation of neural precursor cell spheres attenuates acute experimental allergic encephalomyelitis. Mol Cell Neurosci. 2003. 24:1074–1082.


10. Imitola J, Comabella M, Chandraker AK, Dangond F, Sayegh MH, Snyder EY, Khoury SJ. Neural stem/progenitor cells express costimulatory molecules that are differentially regulated by inflammatory and apoptotic stimuli. Am J Pathol. 2004a. 164:1615–1625.


11. Zappia E, Casazza S, Pedemonte E, Benvenuto F, Bonanni I, Gerdoni E, Giunti D, Ceravolo A, Cazzanti F, Frassoni F, Mancardi G, Uccelli A. Mesenchymal stem cells ameliorate experimental autoimmune encephalomyelitis inducing T-cell anergy. Blood. 2005. 106:1755–1761.


12. Gerdoni E, Gallo B, Casazza S, Musio S, Bonanni I, Pedemonte E, Mantegazza R, Frassoni F, Mancardi G, Pedotti R, Uccelli A. Mesenchymal stem cells effectively modulate pathogenic immune response in experimental autoimmune encephalomyelitis. Ann Neurol. 2007. 61:219–227.


13. Aharonowiz M, Einstein O, Fainstein N, Lassmann H, Reubinoff B, Ben-Hur T. Neuroprotective effect of transplanted human embryonic stem cell-derived neural pre-cursors in an animal model of multiple sclerosis. PLoS ONE. 2008. 3:e3145.


14. Aronowski J, Hall CE. New horizons for primary intra-cerebral hemorrhage treatment: experience from preclinical studies. Neurol Res. 2005. 27:268–279.


15. Chu K, Jeong SW, Jung KH, Han SY, Lee ST, Kim M, Roh JK. Celecoxib induces functional recovery after intra-cerebral hemorrhage with reduction of brain edema and perihematomal cell death. J Cereb Blood Flow Metab. 2004. 24:926–933.


16. Jung KH, Chu K, Jeong SW, Han SY, Lee ST, Kim JY, Kim M, Roh JK. HMG-CoA reductase inhibitor, atorvastatin, promotes sensorimotor recovery, suppressing acute inflammatory reaction after experimental intracerebral hemorrhage. Stroke. 2004. 35:1744–1749.


17. Wang J, Dore S. Inflammation after intracerebral hemorrhage. J Cereb Blood Flow Metab. 2007. 27:894–908.


18. Sinn DI, Kim SJ, Chu K, Jung KH, Lee ST, Song EC, Kim JM, Park DK, Kun Lee S, Kim M, Roh JK. Valproic acid-mediated neuroprotection in intracerebral hemorrhage via histone deacetylase inhibition and transcriptional activation. Neurobiol Dis. 2007. 26:464–472.


19. Hallenbeck JM, Hansson GK, Becker KJ. Immunology of ischemic vascular disease: plaque to attack. Trends Immunol. 2005. 26:550–556.


20. Del Bigio MR, Yan HJ, Buist R, Peeling J. Experimental intracerebral hemorrhage in rats. Magnetic resonance imaging and histopathological correlates. Stroke. 1996. 27:2312–2320.
21. Offner H, Subramanian S, Parker SM, Afentoulis ME, Vandenbark AA, Hurn PD. Experimental stroke induces massive, rapid activation of the peripheral immune system. J Cereb Blood Flow Metab. 2006. 26:654–665.


22. Huston JM, Ochani M, Rosas-Ballina M, Liao H, Ochani K, Pavlov VA, Gallowitsch-Puerta M, Ashok M, Czura CJ, Foxwell B, Tracey KJ, Ulloa L. Splenectomy inactivates the cholinergic antiinflammatory pathway during lethal endotoxemia and polymicrobial sepsis. J Exp Med. 2006. 203:1623–1628.


23. Tracey KJ. Physiology and immunology of the cholinergic antiinflammatory pathway. J Clin Invest. 2007. 117:289–296.


24. Zheng Z, Yenari MA. Post-ischemic inflammation: molecular mechanisms and therapeutic implications. Neurol Res. 2004. 26:884–892.


25. Gregersen R, Lambertsen K, Finsen B. Microglia and macrophages are the major source of tumor necrosis factor in permanent middle cerebral artery occlusion in mice. J Cereb Blood Flow Metab. 2000. 20:53–65.


26. Lee ST, Chu K, Jung KH, Kim SJ, Kim DH, Kang KM, et al. Antiinflammatory mechanism of intravascular neural stem cell transplantation in haemorrhagic stroke. Brain. 2008. 131:616–629.


27. Chamorro A, Urra X, Planas AM. Infection after acute ischemic stroke: a manifestation of brain-induced immunodepression. Stroke. 2007. 38:1097–1103.
28. Dirnagl U, Klehmet J, Braun JS, Harms H, Meisel C, Ziemssen T, Prass K, Meisel A. Stroke-induced immunodepression: experimental evidence and clinical relevance. Stroke. 2007. 38:770–773.
30. Capone C, Frigerio S, Fumagalli S, Gelati M, Principato MC, Storini C, Montinaro M, Kraftsik R, De Curtis M, Parati E, De Simoni MG. Neurosphere-derived cells exert a neuroprotective action by changing the ischemic micro-environment. PLoS ONE. 2007. 2:e373.


31. Imitola J, Raddassi K, Park KI, Mueller FJ, Nieto M, Teng YD, Frenkel D, Li J, Sidman RL, Walsh CA, Snyder EY, Khoury SJ. Directed migration of neural stem cells to sites of CNS injury by the stromal cell-derived factor 1alpha/ CXC chemokine receptor 4 pathway. Proc Natl Acad Sci U S A. 2004b. 101:18117–18122.


32. Belmadani A, Tran PB, Ren D, Miller RJ. Chemokines regulate the migration of neural progenitors to sites of neuroinflammation. J Neurosci. 2006. 26:3182–3191.


33. Mueller FJ, Serobyan N, Schraufstatter IU, DiScipio R, Wakeman D, Loring JF, Snyder EY, Khaldoyanidi SK. Adhesive interactions between human neural stem cells and inflamed human vascular endothelium are mediated by integrins. Stem Cells. 2006. 24:2367–2372.


34. Alvarez-Buylla A, Garcia-Verdugo JM. Neurogenesis in adult subventricular zone. J Neurosci. 2002. 22:629–634.


35. Jin K, Sun Y, Xie L, Mao XO, Childs J, Peel A, Logvinova A, Banwait S, Greenberg DA. Comparison of ischemia- directed migration of neural precursor cells after intrastriatal, intraventricular, or intravenous transplantation in the rat. Neurobiol Dis. 2005. 18:366–374.


36. Pluchino S, Muzio L, Imitola J, Deleidi M, Alfaro-Cervello C, Salani G, Porcheri C, Brambilla E, Cavasinni F, Bergamaschi A, Garcia-Verdugo JM, Comi G, Khoury SJ, Martino G. Persistent inflammation alters the function of the endogenous brain stem cell compartment. Brain. 2008. DOI: 10.1093/brain/awn198.


37. Allorecognition of human neural stem cells by peripheral blood lymphocytes despite low expression of MHC molecules: role of TGF-beta in modulating proliferation. Allorecognition of human neural stem cells by peripheral blood lymphocytes despite low expression of MHC molecules: role of TGFbeta in modulating proliferation. Int Immunol. 2007. 19:1063–1074.
38. Al Nimer F, Wennersten A, Holmin S, Meijer X, Wahlberg L, Mathiesen T. MHC expression after human neural stem cell transplantation to brain contused rats. Neuroreport. 2004. 15:1871–1875.


39. Mammolenti M, Gajavelli S, Tsoulfas P, Levy R. Absence of major histocompatibility complex class I on neural stem cells does not permit natural killer cell killing and prevents recognition by alloreactive cytotoxic T lymphocytes in vitro. Stem Cells. 2004. 22:1101–1110.


40. Wennersten A, Holmin S, Al Nimer F, Meijer X, Wahlberg LU, Mathiesen T. Sustained survival of xenografted human neural stem/progenitor cells in experimental brain trauma despite discontinuation of immunosuppression. Exp Neurol. 2006. 199:339–347.


Fig. 2.
Brain-spleen inflammatory coupling and anti-inflammatory mechanism of systemically-transplanted neural stem cells (NSCs). Brain inflammation initiated by stroke is further augmented by systemic activation of inflammatory cascades. Cerebral inflammation is followed by splenic inflammation, and cytokines and inflammatory cells activated in spleen accelerate cerebral inflammation. Systemically-transplanted NSCs can stop this vicious cycle by interrupting the splenic inflammation.
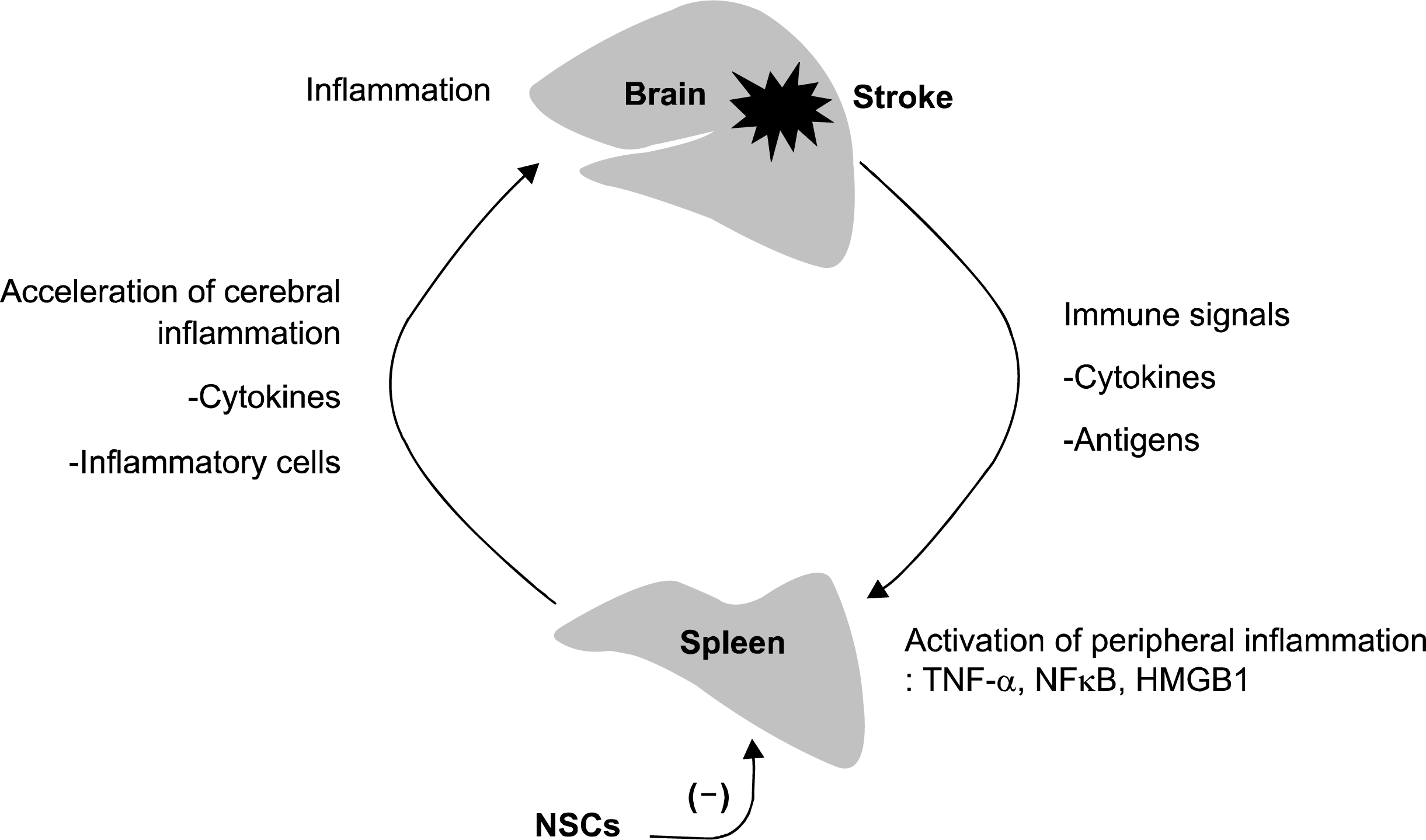
Table 1.
Researches showing anti-inflammatory properties of NSCs
Reference | Model | Cell type | Transplantation method | CNS homing | Systemic distribution | Anti-inflammation | Effects on inflammatory cells |
---|---|---|---|---|---|---|---|
Pluchino et al., 2003 | Mouse EAE | Murine neural progenitors | Intravenous, intracerebroventricular | Positive | Spleen, kidney, liver, lung, gut | Positive | Reduced inflammatory cytokines (MMP, TNF) |
Pluchino et al., 2005 | Mouse EAE | Murine neural progenitors | Intravenous | Positive | Liver, spleen, kidney | Positive | Apoptosis of Th1 cells |
Einstein et al., 2005 | Mouse EAE | Murine neural progenitors | Intracerebroventricular | Positive | Not tested | Positive | Decreasing Th1 cell and increasing Treg cells |
Einstein et al., 2007 | Mouse EAE | Murine neural progenitors | Intravenous | Negative | Spleen, lymph nodes | Positive | Inhibiting Th1 proliferation and activation |
Capone et al., 2007 | Mouse cerebral ischemia | Murine neural progenitors | Intracerebroventricular | Positive | Not tested | Positive | Reducing activated microglia |
Lee et al., 2008 | Rat intracerebral hemorrhage | Human fetal neural stem cells | Intravenous, at acute stage (2hr after ICH) | Rare | Spleen, lung, liver, brain | Positive | Inhibiting splenic macrophage activation |
Aharonowiz et al., 2008 | Mouse EAE | Human ESC-derived neural progenitors | Intracerebroventricular | Positive | Not tested | Positive | Inhibiting Th1 cell activation and reducing macrophage infitration |