Abstract
Background and Objectives
Adipose tissue-derived mesenchymal stem cells (ASCs) are recognized as an advantaged source for the prevention and treatment of diverse diseases including type 2 diabetes mellitus (T2DM). However, alterations in characteristics of ASCs from the aforementioned T2DM patients are still obscure, which also hinder the rigorous and systematic illumination of progression and pathogenesis.
Methods and Results
In this study, we originally isolated peripancreatic adipose tissue-derived mesenchymal stem cells from both human type 2 diabetic and non-diabetic donors (T2DM-ASCs, ND-ASCs) with the parental consent, respectively. We noticed that T2DM-ASCs exhibited indistinguishable immunophenotype, cell vitality, chondrogenic differentiation and stemness as ND-ASCs. Simultaneously, there’s merely alterations in migration and immunoregulatory capacities in T2DM-ASCs. However, differing from ND-ASCs, T2DM-ASCs exhibited deficiency in adipogenic and osteogenic differentiation, and in particular, the delayed cell cycle and different cytokine expression spectrum.
Diabetes mellitus (DM), predominantly consisted of the type 1 (T1DM) and type 2 (T2DM) pathogenetic categories together with other classifications such as gestational DM and drug-induced diabetes, is acknowledged as a metabolic disease and public health concern characterized by hyperglycaemia and defects in insulin secretion and action worldwide (1-3). Of them, patients with T2DM are more pervasive (85∼95% of all cases) due to the combination of inadequate compensatory insulin secretory responses and insulin resistance at cellular level (4-6). Even though the routine therapeutic strategies, including oral antidiabetic agents along with weight management and moderate exercises, together with transplantation of insulin-producing cells (IPCs), could help partially ameliorate hyperglycaemia, yet these therapeutic regiments are largely insufficient for T2DM patients with extensive β cell destruction and subsequent insulin deficiency (1, 3, 7, 8).
Mesenchymal stem cells (MSCs) are acknowledged as an advanced source with unique immunosuppressive and hematopoietic-supporting characteristics for regenerative medicine (8-12). In the 1960s, Friedenstein and his colleagues firstly reported the isolation and identification of the spindle-like MSCs from bone marrow (BM-MSCs) (13). Thereafter, MSCs have been successfully identified from various clinical specimens including adipose tissue (ASCs), placenta (P-MSCs), dental pulp (DPSCs) and umbilical cord (UC-MSCs), and even derived from human pluripotent stem cells (hPSC-MSCs) (9,14-16). Of the above-mentioned MSCs, BM-MSCs with the most extensive applications in clinical trials whereas UC-MSCs possess the most advantageous long-term proliferation feature (10, 17). According to the ClinicalTrials.gov website of National Institutes of Health (NIH), a total number of 983 registered clinical trials with MSCs have been conducted worldwide on multisystem disordering such as osteoarthritis, Crohn’s disease, aplastic anaemia, cerebral stroke, diabetes mellitus, critical limb ischemia (CLI), graft-versus-host disease (GVHD) and acute-on-chronic liver failure (ACLF) (5, 7, 10, 11, 14, 18).
State-of-the-art updates on basic research and clinical medicine have indicated the dysfunction and correlation of MSCs in progression and pathogenesis of numerous diseases (3, 12, 19, 20). For instance, He et al. (21) verified that immune thrombocytopenia (ITP)-MSCs exhibited multifarious defects in signatures such as impaired cell vitality, increased proinflammatory factor secretion, which would accelerate disease progression of ITP patients by altering the hematopoietic microenvironment. Similar phenomenon was also observed in ASCs derived from subcutaneous adipose tissue compared with ND-ASCs (3, 22). With regard to DM, MSCs have been recognized as a pivotal microenvironmental ingredient for the secretion of multiple cytokines and inflammatory factors (e.g., VEGF, IL-6, TNF-α) and the transdifferentiation into insulin-secreting β cells in the pancreas as well (7, 17, 23). Studies on experimental type 1 diabetic mouse model showed that T1DM mice-derived BM-MSCs exhibited indistinguishable characteristics including cytomorphology, immunosuppressive and migration capabilities as the control mice but with abnormally elevated expression of mediators such as MIP-1α/β, MCP-1/5, CCL-2, CCL-3 and IL-6, which facilitated recruitment and accumulation of inflammatory M1 macrophages and aggravated the occurrence of diabetic complications (2, 6, 17, 22). Yet, a considerable variety of defects were observed in T2DM-MSCs including alterations in cell proliferation, cell cycle and apoptosis, migration and multipotential differentiation, based on T2DM-derived BM-MSCs or subcutaneous adipose tissue-derived ASCs (subcutaneous ASCs) (3,24-26). However, the current studies on T2DM-associated MSCs are still piecemeal and even controversial to some extent, and in particular, the systematically exploration of the characteristics and alterations is woefully inadequate as well (3, 20). Hence, there’s a necessity and urgency of performing multidimensional comparation of MSCs from T2DM and non-diabetic donors (ND).
In this study, we reported the isolation and identification of type 2 diabetic adipose tissue -derived ASCs (T2DM-ASCs) and non-diabetic adipose tissue-derived ASCs (ND-ASCs). Differing from the ND-ASCs, T2DM-ASCs exhibited numerous defects in characteristics including the delayed cell cycle and multi-lineage differentiation. Dramatically, the T2DM-ASCs exhibited indiscri minate signatures in cytomorphology, immunophenotype, migration, chondrogenic differentiation potential together with comparable immunosuppressive property and stemness as well. Taken together, we originally isolated and systematically evaluated the phenotype of T2DM-ASCs, which also held the potential as an alternative source of MSCs for autologous application in regenerative medicine.
Human peripancreatic adipose tissues were obtained from T2DM (n=3) and non-diabetic organ donors (n=5) with informed consents, between May 2017 and October 2019. ND-ASCs and T2DM-ASCs were extracted from the indicated adipose tissues according to the guideline of Declaration of Helsinki. All the experimental procedures were approved by the Ethics Committee of Tianjin First Central Hospital of Nankai University (ethics number: 2017N080KY). The information of the ND and T2DM donors is available in Table 1.
As mentioned above, ND-ASCs and T2DM-ASCs were isolated from peripancreatic adipose tissues by enzymatic digestion. Briefly, 20 ml adipose tissues were harvested from the candidates and washed with 1× PBS in a 50 ml Falcon tube (Sigma-Aldrich), and digested with an equal volume of 1 mg/ml collagenase type I at 37℃ for 30 min. Then, the stromal vascular fraction cells were isolated by centrifugation at 300 ×g at room temperature and cultured in 25 cm2 flasks in Serum-Free Human MSC Medium (Yocon) supplemented with 100 U/ml penicillin and streptomycin (Gibco). The ND-ASCs and T2DM-ASCs were enriched and purified by continuous passages in the abovementioned culture medium at 37℃, 5% CO2. T2DM-ASCs and ND-ASCs at passage 2∼6 were used for phenotypic analysis.
Flow cytometry assay were conducted as we recently described (27, 28). Briefly, ND-ASCs and T2DM-ASCs were dissociated into single cells by 0.25% Trypsin-EDTA (Gibco) and labelled with the indicated antibodies against CD34-APC, CD44-APC, CD45-FITC, CD73-FITC, CD90-FITC, CD105-APC, together with isotype matched IgG1-FITC and IgG2b-APC (BD Pharmigen), in 0.2% BSA for 30 min in dark and washing with 1× PBS for twice. Finally, the ND-ASCs and T2DM-ASCs were analysed by Accuri C6 Plus (BD, San Jose, CA, USA). The flow cytometry assay results were analysed with FlowJo 7.0 software (BD, San Jose, CA, USA).
Cell proliferation of ND-ASCs and T2DM-ASCs were conducted by utilizing the CCK-8 kit (Sigma-Aldrich, St. Louis, USA) according to the manufacturer’s instructions. In brief, 5×103 ND-ASCs and T2DM-ASCs were seeded in 96-well plates (100 μl/well) at a density of per well with 5 replications for each time point (4 h, 24 h, 48 h, 72 h) at 37℃, 5% CO2. After that, ND-ASCs and T2DM-ASCs were incubated with 10 μl CCK-8 solution at 37℃ for an additional 2 h. The absorbance of the solutions was measured at 450 nm using a microplate absorbance reader (Bio-Rad, California, USA).
ND-ASCs and T2DM-ASCs were collected and incubated with Annexin V and 7-AAD (BD) for 15 min at RT according to the BD manufacture’s instruction. The proportion of apoptotic ND-ASCs and T2DM-ASCs were quantified by flow cytometry analysis using an Accuri C6 plus instrument (BD, San Jose, CA, USA). All flow cytometry assay data were analysed with FlowJo 7.0 software (BD, San Jose, CA, USA).
ND-ASCs and T2DM-ASCs were collected and fixed in 70% ethanol for at least 2 h at 4℃. After washing with 1× PBS for twice, ND-ASCs and T2DM-ASCs were incubated for 30 min at room temperature with 10 μg/ml propidium iodide (PI, Beyotime) staining solution. The distribution of multiple cell cycle phases was quantified by flow cytometry using an Accuri C6 plus instrument (BD, San Jose, CA, USA).
Adipogenic differentiation was conducted as we recently reported with several modification (10). In details, ND-ASCs and T2DM-ASCs were seeded at a density of 2×104 cells/cm2 at 37℃, 5% CO2. When cells reached 80% confluent, the supernatant was discarded and changed into adipogenic differentiation medium containing 1 μM dexamethasone, 0.1 mM indomethacin, 0.5 mM 3-isobutyl-1-methylxanthine, and 10 mg/L insulin in Serum-free human MSC medium (Yocon). The medium was subsequently changed twice a week. After 21 days, adipogenic differentiation was assessed by Oil Red O staining of the fat droplets. The phase contrast image was photographed with Nikon ElipseTi-U microscope (Nikon, Tokyo, Japan).
Osteogenic differentiation was conducted as we previously described with several modification (10). In details, ND-ASCs and T2DM-ASCs were seeded at a density of 2×104 cells/cm2 at 37℃, 5% CO2. When cells reached 80% confluent, the supernatant was discarded and changed into osteogenic differentiation medium containing 100 nM dexamethasone, 50 μM L-ascorbic acid 2-phosphate, and 10 mM β-glycerophosphate in Serum-free human MSC medium (Yocon). The medium was subsequently changed twice a week. After 28 days, osteogenic differentiation was assessed by von Kossa staining (Abcam). The phase contrast image was photographed with Nikon ElipseTi-U microscope (Nikon, Tokyo, Japan).
Chondrogenic differentiation was performed as we recently reported (10). Briefly, ND-ASCs and T2DM-ASCs were seeded at a density of 2×104 cells/cm2 at 37℃, 5% CO2. When cells reached 80% confluent, the supernatant was discarded and changed into chondrogenic differentiation medium (StemCells). The medium was subsequently changed twice a week. After 28 days, chondrogenic differentiation was assessed by Alcian Blue staining (Abcam). The phase contrast image was photographed with Nikon ElipseTi-U microscope (Nikon, Tokyo, Japan).
Total RNA extraction and cDNA synthesis were performed by utilizing the RNeasy Mini Kit (QIAGEN) and PrimeScript RT reagent Kit with gDNA Eraser (Takara) as we described (23). qRT-PCR was performed with SYBR Premix ExTaq II (Takara) and LightCycler96 System (Roche). Relative mRNA expression of the indicated genes was calculated by the 2−DDCT method. GAPDH was used as an internal control. The Primer sequences are listed in Table 2.
Inhibition of lymphocyte proliferation to human peripheral blood mononuclear cells (PBMCs) by the indicated ASCs. In brief, PBMCs were isolated from peripheral blood of healthy donors by standard Ficoll density gradient centrifugation. Then, the prepared PBMCs were cocultured with or without 5×104 ND-ASCs or T2DM-ASCs at a ratio of 5:1 in 96-well plates at 37℃, 5% CO2 in the presence of 5 ng/μl PHA. 3 days later, PBMCs in the supernatant were collected and labelled with antibodies against CD3 and analysed by Accuri C6 Plus (BD, San Jose, CA, USA) and Flowjo software (BD, San Jose, CA, USA).
ND-ASCs or T2DM-ASCs were seeded in 6-well plate, when the ND-ASCs or T2DM-ASCs reached 80% confluent, the indicated cell layers were scratched with 200 μl tips and captured with a Nikon ElipseTi-U microscope (Nikon, Tokyo, Japan) at the indicated time points (0 h, 12 h, 24 h). Images of the scratch area were captured and analyzed by the ImageJ software.
Statistical analysis in the study was conducted by utilizing Prism 5 software (GraphPad, San Diego, USA). The data were presented as means±SEM. Student’s t-test was used to analyse the data of the two indicated groups. p< 0.05 was considered statistically significant. *, p<0.05; **, p<0.01; ***, p<0.001; NS, not significant.
To clarify the underlying type 2 diabetes-associated pathogenesis and uncover the potential defects of MSCs during disease progression, we originally isolated and cultured the peripancreatic adipose tissue-derived MSCs from 3 T2DM (T2DM-ASCs) and 5 non-diabetic donors (ND-ASCs), according to the Declaration of Helsinki and the Ethics Committee of Tianjin First Central Hospital (Table 1). Compared with ND-ASCs, T2DM-ASCs exhibited indistinguishable spindle-like cytomorphology after in vitro passages for 3 times (Fig. 1A). Flow cytometry analysis showed that both T2DM-ASCs and ND-ASCs expressed high level of MSC-associated biomarkers (CD44, CD73, CD90, CD105) (>95%), whereas with minimal expression of hematopoietic or endothelial-associated biomarkers (CD34, CD45) (Fig. 1B and 1C). Taken together, we successfully obtained T2DM-ASCs and ND-ASCs from peripancreatic adipose tissue, whereas the indicated ASCs showed indistinguishable cytomorphology and immunophenotype.
We then speculated whether the cell viability of the two ASCs was distinguished from each other. For the purpose, we originally conducted cell count analysis and found that T2DM-ASCs and ND-ASCs showed comparable cell proliferation capacity, which was further verified by the growth curve (Fig. 2A and 2B). With the aid of flow cytometry analysis, we found T2DM-ASCs showed a cell cycle arrest at G0/G1 phases and a reduction in G2/M phases when compared with ND-ASCs, which indicated the impaired cell cycle in T2DM-ASCs (Fig. 2C and 2D). Unexpectedly, the proportion of population with early apoptotic (Annexin Ⅴ+ 7-AAD−) or apoptotic (Annexin Ⅴ+) property in T2DM-ASCs was consistent with that in the ND-ASCs group, which was identified by 7-AAD and Annexin Ⅴ staining (Fig. 2E and 2F). In summary, the isolated T2DM-ASCs held similarities with ND-ASCs in most of cell viability except for the distribution of multiple cell cycle phases.
To further illuminate the potential distinctions in biological signatures, we subsequently detected the adipogenic, osteogenic and chondrogenic differentiation capacity of T2DM-ASCs and ND-ASCs. As shown by Oil Red S staining, fewer typical adipose droplets were generated from T2DM-ASCs after 21 days of adipogenic differentiation compared with the ND-ASCs group (Fig. 3A, Supplementary Fig. S1A). Quantitative analysis of adipogenic markers (e.g., PPAR-γ) further confirmed the decline of adipocyte generation in the T2DM-ASCs group (Fig. 3B). Interestingly, ND-ASCs-derived cells also showed preferable osteogenic differentiation capacity than that in the T2DM-ASCs group as indicated by von Kossa staining (Fig. 3C, Supplementary Fig. S1B) and expression of osteogenic marker, BGLAP (Fig. 3D). Differing from the aforementioned adipogenic and osteogenic differentiation, T2DM-ASCs revealed indistinguishable chondrogenic differentiation potential as confirmed by Alcian Blue staining (Fig. 3E, Supplementary Fig. S1C) and ACAN mRNA expression (Fig. 3F). Hence, T2DM-ASCs possessed impaired adipogenic and osteogenic differentiation potential to the ND-ASCs group.
To date, we and other investigators have manifested the alterations of ASCs during multiple pathological processes including cell migration, immunosuppressive capacity, together with numerous cytokine secretion. Thus, we primitively took advantage of wound healing analysis and found that T2DM-ASCs with attenuated migration capacity compared with that in ND-ASCs (Fig. 4A and 4B). Simultaneously, by conducting the coculture of ASCs with peripheral blood mononuclear cells (PBMCs), we noticed that the inhibitory capacity of T2DM-ASCs on proliferation of CD3+ T cells was comparable to the PBMCs+PHA+ND-ASCs group (Fig. 4C and 4D). Collectively, T2DM-ASCs exhibited indiscriminate signatures with ND-ASCs in migration and immunoregulation.
Having primitively verified the indiscriminate immunosuppression upon PBMC proliferation, we next are wondering about the expression levels of cytokines as well as inflammatory factors between the two indicated ASCs. For the purpose, we conducted quantitative analysis of a variety of cytokines in ND-ASCs and T2DM-ASCs, and found there were no discernible differences in IDO1, SDF-1 or CXCR4 expression (Fig. 5A). Meanwhile, besides those with no statistically significant differences in expression, we also noticed the alterations such as HGF, TGF-β, IGF-1, IL-1Ra and TSG-6, which were involved in regulation of islet cell function (Fig. 5B). However, the expression levels of stemness-associated biomarkers (POU5F1, NANOG) in ND-ASCs and T2DM-ASCs at various passages (P2, P6) didn’t show statistically significant difference in vitro, which confirmed the retained property of T2DM-ASCs in the stemness potential (Fig. 5C).
Longitudinal studies have demonstrated adipose tissue as an easily accessible source of high-quality mesenchymal stem cells (MSCs) with extensive prospects for clinical applications, which are also acknowledged as a pivotal microenvironmental component involved in numerous disease progression (5, 11, 19, 21). However, there’s merely reports on stem cell identification from T2DM- or ND-derived peripancreatic adipose tissues (2). Above all, the systematic comparation of T2DM-ASCs with ND-ASCs is largely unprocurable as well. Therefore, we originally isolated the indicated peripancreatic ASCs and comprehensively investigated the signatures to dissect the alterations occurred in T2DM-ASCs. For instance, compared with ND-ASCs, these T2DM-ASCs exhibited impaired adipogenic and osteogenic differentiation properties, together with delayed cell cycle. Hence, our findings have provided new evidence for the defects of T2DM-ASCs, together with the explanation to pathogenesis of type 2 diabetes mellitus as well.
For long stretches, to our knowledge, no reports have been documented on isolating T2DM-ASCs from peripancreatic adipose tissue (4, 7). For decades, we and other investigators have identified ASCs from subcutaneous adipose tissue for applications in regenerative medicine (3, 5). However, in this study, the identified T2DM-ASCs were isolated from the peripancreatic adipose tissue of type 2 diabetesmellitus, which was distinguished from the reported ASCs. For instance, the subcutaneous adipose tissue-derived ASCs from T2DM patients possessed enhanced differentiation capacity into adipocytes whereas declined potential into osteoblasts (24). The contrarious conclusions might be largely ascribed to the sophisticated and polytropic microenvironment induced by inflammatory factors or metabolites during the disease progression (11, 20, 25). For example, diabetes and obesity could contribute to local hypoxia in adipose tissue and increase the secretion of hormones, growth factors, and adipokines to initiate the proliferation, adipogenic differentiation or microvessel formation of ASCs, together with the immune properties (10,29-31). Although it has been reported that a short term of high glucose stimulation reduced proliferation of gestational-MSCs but enhanced adipogenic differentiation in vitro (32), our results demonstrated that the peripanceatic diabetic milieus, including hyperglycemia, cause cell cycle delay as well as compromised adipogenic and osteogenic differentiation of T2DM-ASCs in the long run. Overall, there’s no surprises that ASCs with different origins might exhibit dissimilar biological potentials, which has been demonstrated among subtypes of MSCs such as the above-mentioned UC-MSCs, P-MSCs and BM-MSCs (8, 10, 17). In addition, the defects in cell vitality including the delayed cell cycle between T2DM-ASCs and ND-ASCs in this study were consist with current reports that hypoxia and hyperglycaemia didn’t affect proliferation of ASCs but “stemness” (12, 20, 26, 33).
MSCs have been acknowledged as a splendid candidate source for disease managements such as hematologic malignancies, graft-versus-host disease (GVHD), acute myocardial infarction, liver cirrhosis and diabetes mellitus (4, 5, 11, 18, 27). To date, ASCs together with other tissue derived MSCs, including the non-invasive umbilical cord-derive MSCs (UC-MSCs), placental-derived MSCs (P-MSCs) and dental pulp stem cells (DPSCs) together with even pluripotent stem cell-derived MSCs (PSC-MSCs), have been demonstrated preferable alternatives of the invasive bone marrow-derived MSCs (BM-MSCs) for future clinical applications (5, 9, 11). However, systematic and detailed dissection of T2DM-ASCs both at the cellular and molecular levels is fundamental and prerequisite to discover the proofs on pathogenesis of diabetes mellitus (7, 19). For instance, Wang et al. (23) and Krishnan et al. (34) reported that intravenous injection of ASCs was sufficient to improve insulin sensitivity and alleviate β cell death and even ameliorate hippocampal dysfunction of T2DM percipient mice, respectively. Congruously, we currently verified that MSCs participated in the restorative process of dysfunctional type 2 diabetic islets by reversing β cell dedifferentiation, yet the underling pathogenesis of T2DM-ASCs involved in the progression and efficacy still need further systematic and in-depth research (23). Here, the present study further dissected the potential of T2DM-ASCs in the treatment of T2DM. MSCs ameliorate islet dysfunction mainly through its paracrine function, secreting factors modulating inflammation and immunity, as reviewed in previous studies (3, 35-37). Some of these MSCs-secreted factors have been reported improving β cell function, proliferation or viability, such as IGF (38), HGF (39) and IL-1Ra (23). Thus we evaluated the gene expression of these factors and results showed that IGF-1, HGF, and IL-1Ra were significantly downregulated in T2DM-ASCs, while the factors that modulating immunity have no significant change, suggesting a possibility of compromised ability of T2DM-ASCs in improving the islet pathology of T2DM patients.
In the present study, we have isolated and identified human T2DM-ASCs and ND-ASCs, respectively. Compared with ND-ASCs, T2DM-ASCs show limited multifaceted alterations in biological signatures including multi-differentiation potentials, cell vitality, PBMC inhibition and stemness together with indistinguishable characteristics as ND-ASCs as well. Above all, the minimal and conservative alterations of T2DM-ASCs in multifaceted characteristics also indicated the possibility of autologous application of ASCs for cell-based T2DM treatment in the future, yet there is still a need to develop strategies to further improve T2DM-ASCs functions.
Supplementary data including one figure can be found with this article online at http://pdf.medrang.co.kr/paper/pdf/IJSC/IJSC-13-s20028.pdf.
Acknowledgments
The work was supported by the National Natural Science Foundation of China (81870535), Key projects of Tianjin Natural Science Foundation (18JCZDJC33100), Chun Foundation of Tianjin First Central Hospital (2019CM01 and 2019CF28), Annual scientific and technological activities for overseas graduates in Tianjin (2019), China Postdoctoral Science Foundation (2019M661033), Natural Science Foundation of Tianjin (19JCQNJC12500).
References
1. Yang W, Lu J, Weng J, Jia W, Ji L, Xiao J, Shan Z, Liu J, Tian H, Ji Q, Zhu D, Ge J, Lin L, Chen L, Guo X, Zhao Z, Li Q, Zhou Z, Shan G, He J. China National Diabetes and Metabolic Disorders Study Group. 2010; Prevalence of diabetes among men and women in China. N Engl J Med. 362:1090–1101. DOI: 10.1056/NEJMoa0908292. PMID: 20335585.


2. He X, Yang Y, Yao MW, Ren TT, Guo W, Li L, Xu X. 2019; Full title: High glucose protects mesenchymal stem cells from metformin-induced apoptosis through the AMPK-mediated mTOR pathway. Sci Rep. 9:17764. DOI: 10.1038/s41598-019-54291-y. PMID: 31780804. PMCID: PMC6882892.


3. Zang L, Hao H, Liu J, Li Y, Han W, Mu Y. 2017; Mesenchymal stem cell therapy in type 2 diabetes mellitus. Diabetol Metab Syndr. 9:36. DOI: 10.1186/s13098-017-0233-1. PMID: 28515792. PMCID: PMC5433043.


4. Cho J, D'Antuono M, Glicksman M, Wang J, Jonklaas J. 2018; A review of clinical trials: mesenchymal stem cell transplant therapy in type 1 and type 2 diabetes mellitus. Am J Stem Cells. 7:82–93. PMID: 30510843. PMCID: PMC6261870.
5. Qi Y, Ma J, Li S, Liu W. 2019; Applicability of adipose-derived mesenchymal stem cells in treatment of patients with type 2 diabetes. Stem Cell Res Ther. 10:274. DOI: 10.1186/s13287-019-1362-2. PMID: 31455405. PMCID: PMC6712852.


6. Ying W, Fu W, Lee YS, Olefsky JM. 2020; The role of macrophages in obesity-associated islet inflammation and β-cell abnormalities. Nat Rev Endocrinol. 16:81–90. DOI: 10.1038/s41574-019-0286-3. PMID: 31836875.


7. Holmes D. 2014; Diabetes: MSC transplant prevents β-cell dysfunction. Nat Rev Endocrinol. 10:701. DOI: 10.1038/nrendo.2014.172. PMID: 25265979.
8. Samsonraj RM, Raghunath M, Nurcombe V, Hui JH, van Wijnen AJ, Cool SM. 2017; Concise review: multifaceted characterization of human mesenchymal stem cells for use in regenerative medicine. Stem Cells Transl Med. 6:2173–2185. DOI: 10.1002/sctm.17-0129. PMID: 29076267. PMCID: PMC5702523.


9. Zhang L, Wang H, Liu C, Wu Q, Su P, Wu D, Guo J, Zhou W, Xu Y, Shi L, Zhou J. 2018; MSX2 Initiates and accelerates mesenchymal stem/stromal cell specification of hPSCs by regulating TWIST1 and PRAME. Stem Cell Reports. 11:497–513. DOI: 10.1016/j.stemcr.2018.06.019. PMID: 30033084. PMCID: PMC6092836.


10. Zhao Q, Zhang L, Wei Y, Yu H, Zou L, Huo J, Yang H, Song B, Wei T, Wu D, Zhang W, Zhang L, Liu D, Li Z, Chi Y, Han Z, Han Z. 2019; Systematic comparison of hUC-MSCs at various passages reveals the variations of signatures and therapeutic effect on acute graft-versus-host disease. Stem Cell Res Ther. 10:354. DOI: 10.1186/s13287-019-1478-4. PMID: 31779707. PMCID: PMC6883552.


11. Kabat M, Bobkov I, Kumar S, Grumet M. 2020; Trends in mesenchymal stem cell clinical trials 2004-2018: is efficacy optimal in a narrow dose range? Stem Cells Transl Med. 9:17–27. DOI: 10.1002/sctm.19-0202. PMID: 31804767. PMCID: PMC6954709.


12. Shi Y, Wang Y, Li Q, Liu K, Hou J, Shao C, Wang Y. 2018; Immunoregulatory mechanisms of mesenchymal stem and stromal cells in inflammatory diseases. Nat Rev Nephrol. 14:493–507. DOI: 10.1038/s41581-018-0023-5. PMID: 29895977.


13. Friedenstein AJ, Petrakova KV, Kurolesova AI, Frolova GP. 1968; Heterotopic of bone marrow. Analysis of precursor cells for osteogenic and hematopoietic tissues. Transplantation. 6:230–247. DOI: 10.1097/00007890-196803000-00009. PMID: 5654088.
14. Wei Y, Hou H, Zhang L, Zhao N, Li C, Huo J, Liu Y, Zhang W, Li Z, Liu D, Han Z, Zhang L, Song B, Chi Y, Han Z. 2019; JNKi- and DAC-programmed mesenchymal stem/stromal cells from hESCs facilitate hematopoiesis and alleviate hind limb ischemia. Stem Cell Res Ther. 10:186. DOI: 10.1186/s13287-019-1302-1. PMID: 31234947. PMCID: PMC6591900.


15. Zhang X, Yang Y, Zhang L, Lu Y, Zhang Q, Fan D, Zhang Y, Zhang Y, Ye Z, Xiong D. 2017; Mesenchymal stromal cells as vehicles of tetravalent bispecific Tandab (CD3/CD19) for the treatment of B cell lymphoma combined with IDO pathway inhibitor D-1-methyl-tryptophan. J Hematol Oncol. 10:56. DOI: 10.1186/s13045-017-0397-z. PMID: 28228105. PMCID: PMC5322661.


16. Yao J, Chen N, Wang X, Zhang L, Huo J, Chi Y, Li Z, Han Z. 2020; Human supernumerary teeth-derived apical papillary stem cells possess preferable characteristics and efficacy on hepatic fibrosis in mice. Stem Cells Int. 2020:6489396. DOI: 10.1155/2020/6489396. PMID: 32399047. PMCID: PMC7204141.


17. Pourgholaminejad A, Aghdami N, Baharvand H, Moazzeni SM. 2016; The effect of pro-inflammatory cytokines on immunophenotype, differentiation capacity and immunomodulatory functions of human mesenchymal stem cells. Cytokine. 85:51–60. DOI: 10.1016/j.cyto.2016.06.003. PMID: 27288632.


18. McGonagle D, Baboolal TG, Jones E. 2017; Native joint-resident mesenchymal stem cells for cartilage repair in osteoarthritis. Nat Rev Rheumatol. 13:719–730. DOI: 10.1038/nrrheum.2017.182. PMID: 29118440.


19. Kfoury Y, Scadden DT. 2015; Mesenchymal cell contributions to the stem cell niche. Cell Stem Cell. 16:239–253. DOI: 10.1016/j.stem.2015.02.019. PMID: 25748931.


20. Nombela-Arrieta C, Ritz J, Silberstein LE. 2011; The elusive nature and function of mesenchymal stem cells. Nat Rev Mol Cell Biol. 12:126–131. DOI: 10.1038/nrm3049. PMID: 21253000. PMCID: PMC3346289.


21. He Y, Xu LL, Feng FE, Wang QM, Zhu XL, Wang CC, Zhang JM, Fu HX, Xu LP, Liu KY, Huang XJ, Zhang XH. 2018; Mesenchymal stem cell deficiency influences megakaryocytopoiesis through the TNFAIP3/NF-κB/SMAD pathway in patients with immune thrombocytopenia. Br J Haematol. 180:395–411. DOI: 10.1111/bjh.15034. PMID: 29327472.


22. Weisberg SP, McCann D, Desai M, Rosenbaum M, Leibel RL, Ferrante AW Jr. 2003; Obesity is associated with macrophage accumulation in adipose tissue. J Clin Invest. 112:1796–1808. DOI: 10.1172/JCI200319246. PMID: 14679176. PMCID: PMC296995.


23. Wang L, Liu T, Liang R, Wang G, Liu Y, Zou J, Liu N, Zhang B, Liu Y, Ding X, Cai X, Wang Z, Xu X, Ricordi C, Wang S, Shen Z. 2020; Mesenchymal stem cells ameliorate β cell dysfunction of human type 2 diabetic islets by reversing β cell dedifferentiation. EBioMedicine. 51:102615. DOI: 10.1016/j.ebiom.2019.102615. PMID: 31918404. PMCID: PMC7000334.
24. Kočí Z, Turnovcová K, Dubský M, Baranovičová L, Holáň V, Chudíčková M, Syková E, Kubinová S. 2014; Characterization of human adipose tissue-derived stromal cells isolated from diabetic patient's distal limbs with critical ischemia. Cell Biochem Funct. 32:597–604. DOI: 10.1002/cbf.3056. PMID: 25251698.


25. Barbagallo I, Li Volti G, Galvano F, Tettamanti G, Pluchinotta FR, Bergante S, Vanella L. 2017; Diabetic human adipose tissue-derived mesenchymal stem cells fail to differentiate in functional adipocytes. Exp Biol Med (Maywood). 242:1079–1085. DOI: 10.1177/1535370216681552. PMID: 27909015. PMCID: PMC5444636.


26. Alicka M, Major P, Wysocki M, Marycz K. 2019; Adipose-derived mesenchymal stem cells isolated from patients with type 2 diabetes show reduced "stemness" through an altered secretome profile, impaired anti-oxidative protection, and mitochondrial dynamics deterioration. J Clin Med. 8:765. DOI: 10.3390/jcm8060765. PMID: 31151180. PMCID: PMC6617220.


27. Lim M, Wang W, Liang L, Han ZB, Li Z, Geng J, Zhao M, Jia H, Feng J, Wei Z, Song B, Zhang J, Li J, Liu T, Wang F, Li T, Li J, Fang Y, Gao J, Han Z. 2018; Intravenous injection of allogeneic umbilical cord-derived multipotent mesenchymal stromal cells reduces the infarct area and ameliorates cardiac function in a porcine model of acute myocardial infarction. Stem Cell Res Ther. 9:129. DOI: 10.1186/s13287-018-0888-z. PMID: 29751831. PMCID: PMC5948807.


28. Zhang L, Liu C, Wang H, Wu D, Su P, Wang M, Guo J, Zhao S, Dong S, Zhou W, Arakaki C, Zhang X, Zhou J. 2018; Thrombopoietin knock-in augments platelet generation from human embryonic stem cells. Stem Cell Res Ther. 9:194. DOI: 10.1186/s13287-018-0926-x. PMID: 30016991. PMCID: PMC6050740.


29. Pachón-Peña G, Serena C, Ejarque M, Petriz J, Duran X, Oliva-Olivera W, Simó R, Tinahones FJ, Fernández-Veledo S, Vendrell J. 2016; Obesity determines the immunophenotypic profile and functional characteristics of human mesenchymal stem cells from adipose tissue. Stem Cells Transl Med. 5:464–475. DOI: 10.5966/sctm.2015-0161. PMID: 26956208. PMCID: PMC4798735.


30. Serena C, Keiran N, Ceperuelo-Mallafre V, Ejarque M, Fradera R, Roche K, Nuñez-Roa C, Vendrell J, Fernández-Veledo S. 2016; Obesity and type 2 diabetes alters the immune properties of human adipose derived stem cells. Stem Cells. 34:2559–2573. DOI: 10.1002/stem.2429. PMID: 27352919.


31. Kornicka K, Houston J, Marycz K. 2018; Dysfunction of mesenchymal stem cells isolated from metabolic syndrome and type 2 diabetic patients as result of oxidative stress and autophagy may limit their potential therapeutic use. Stem Cell Rev Rep. 14:337–345. DOI: 10.1007/s12015-018-9809-x. PMID: 29611042. PMCID: PMC5960487.


32. Hankamolsiri W, Manochantr S, Tantrawatpan C, Tantikanlayaporn D, Tapanadechopone P, Kheolamai P. 2016; The effects of high glucose on adipogenic and osteogenic differentiation of gestational tissue-derived MSCs. Stem Cells Int. 2016:9674614. DOI: 10.1155/2016/9674614. PMID: 27057179. PMCID: PMC4707328.


33. Lafosse A, Dufeys C, Beauloye C, Horman S, Dufrane D. 2016; Impact of hyperglycemia and low oxygen tension on adipose-derived stem cells compared with dermal fibroblasts and keratinocytes: importance for wound healing in type 2 diabetes. PLoS One. 11:e0168058. DOI: 10.1371/journal.pone.0168058. PMID: 27992567. PMCID: PMC5167273.


34. Krishnan B, Sallam HS, Tumurbataar B, Saieva S, Baymon D, Tuvdendorj D, Micci MA, Abate N, Taglialatela G. 2020; Amelioration of hippocampal dysfunction by adipose tissue-targeted stem cell transplantation in a mouse model of type 2 diabetes. J Neurochem. 153:51–62. DOI: 10.1111/jnc.14915. PMID: 31730234.


35. Qi K, Li N, Zhang Z, Melino G. 2018; Tissue regeneration: the crosstalk between mesenchymal stem cells and immune response. Cell Immunol. 326:86–93. DOI: 10.1016/j.cellimm.2017.11.010. PMID: 29221689.


36. Caplan AI. 2017; Mesenchymal stem cells: time to change the name! Stem Cells Transl Med. 6:1445–1451. DOI: 10.1002/sctm.17-0051. PMID: 28452204. PMCID: PMC5689741.


37. Bernardo ME, Fibbe WE. 2013; Mesenchymal stromal cells: sensors and switchers of inflammation. Cell Stem Cell. 13:392–402. DOI: 10.1016/j.stem.2013.09.006. PMID: 24094322.


38. Cornu M, Thorens B. 2009; GLP-1 protects β-cells against apoptosis by enhancing the activity of an IGF-2/IGF1-receptor autocrine loop. Islets. 1:280–282. DOI: 10.4161/isl.1.3.9932. PMID: 21099285.


39. Gaddy DF, Riedel MJ, Pejawar-Gaddy S, Kieffer TJ, Robbins PD. 2010; In vivo expression of HGF/NK1 and GLP-1 from dsAAV vectors enhances pancreatic ß-cell proliferation and improves pathology in the db/db mouse model of diabetes. Diabetes. 59:3108–3116. DOI: 10.2337/db09-1886. PMID: 20841608. PMCID: PMC2992772.


Fig. 1
T2DM-ASCs show similar cytomorphology and immunophenotype. (A) Morphology of ND-ASCs and T2DM-ASCs under same conditions (left, 40× magnification; right, 100× magnification,). (B, C) Immunophenotype of ND-ASCs (n=5) and T2DM-ASCs (n=3) at P6, analysed by flow cytometry. The markers including CD44, CD73, CD90, CD105, CD34 and CD45. The data were shown as the mean±SEM, each experiment was repeated at least twice, one representative example shown. NS: not significant.
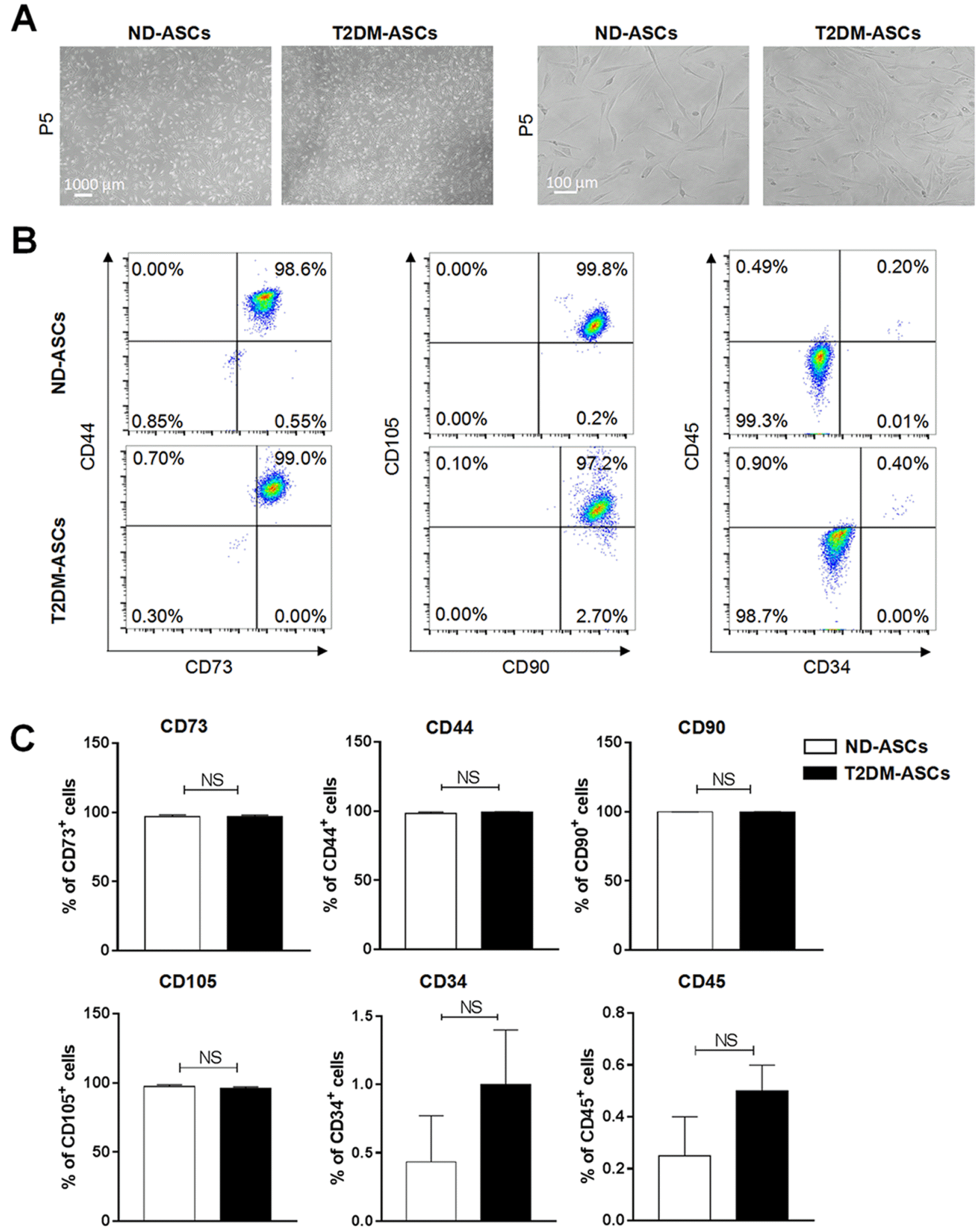
Fig. 2
T2DM-ASCs exhibit indiscriminate signatures in cell viability. (A) Cell count of ND-ASCs (n=4) and T2DM-ASCs (n=3) at P5. (B) CCK-8 assay of ND-ASCs (n=3) and T2DM-ASCs for 4, 24, 48 and 72 h. (C) PI staining profiles of ND-ASCs (n=4) and T2DM-ASCs (n=3) by flow cytometry. (D) Histograms statistics of cell cycle status populations. (E) 7-AAD and Annexin V staining profiles of ND-ASCs (n=4) and T2DM-ASCs (n=3) by flow cytometry. (F) Histograms statistics of cell apoptosis, including early apoptosis (Annexin Ⅴ+7-AAD−) and total apoptosis (Annexin Ⅴ+) populations. The data were shown as the mean± SEM, each experiment was repeated at least twice, one representative example shown. NS: not significant. ***p<0.001.
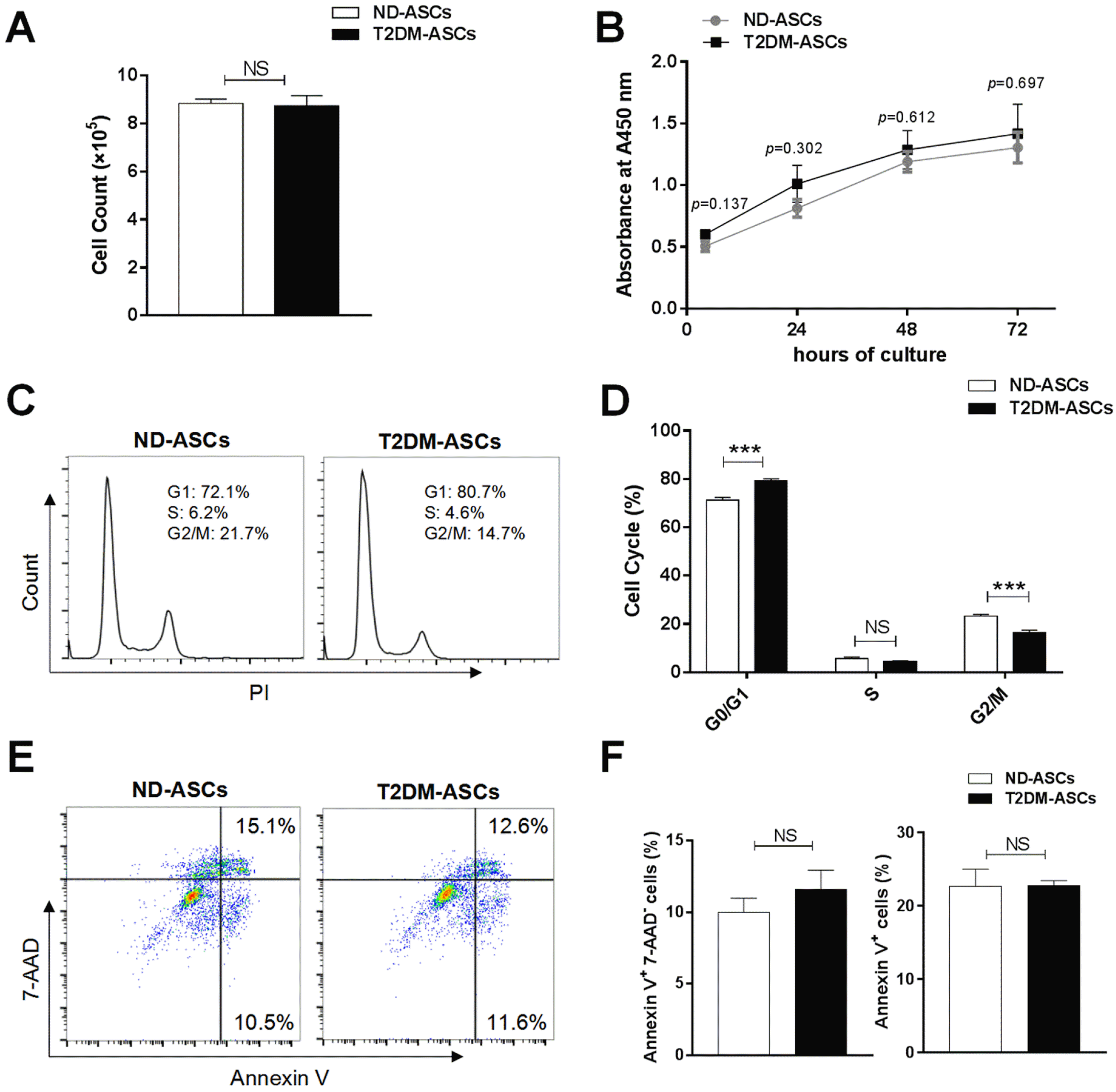
Fig. 3
T2DM-ASCs possess impaired adipogenic and osteogenic differentiation potential. (A) The morphology of adipogenic differentiation of ND-ASCs (n=3) and T2DM-ASCs (n=3) by Oil Red O staining (200× magnification). (B) qRT-PCR assessment of adipogenic marker PPAR-γ. (C) The morphology of osteogenic differentiation of ND-ASCs (n=3) and T2DM-ASCs (n=3) by von Kossa staining (200× magnification). (D) qRT-PCR assessment of osteogenic marker BGLAP. (E) The morphology of chondrogenic differentiation of ND-ASCs (n=3) and T2DM-ASCs (n=3) by Alcian Blue staining (200× magnification). (F) qRT-PCR assessment of chondrogenic marker ACAN. All values are normalized to the negative control (NC) group. The data were shown as the mean±SEM, each experiment was repeated at least twice, one representative example shown. *p<0.05, **p<0.01.
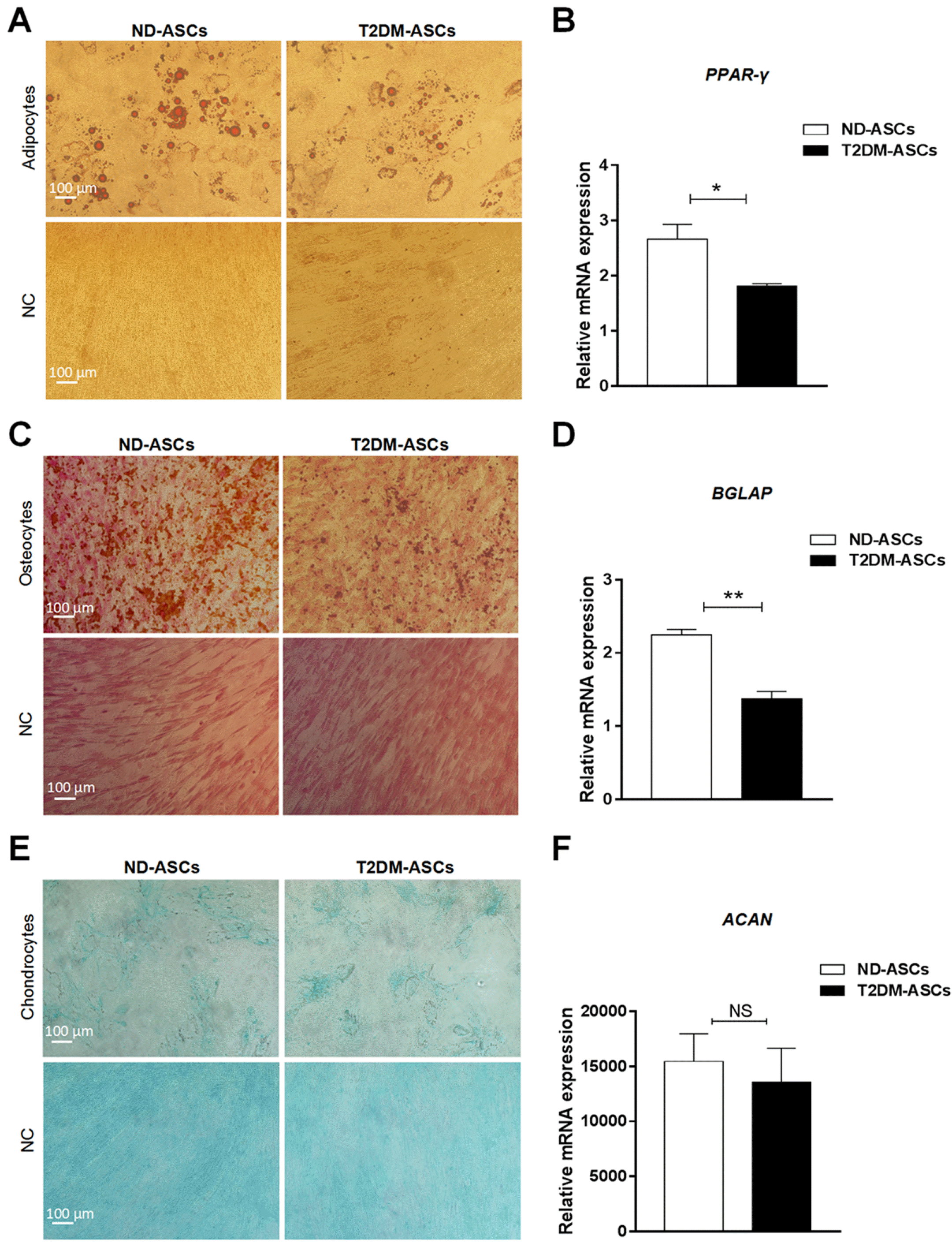
Fig. 4
T2DM-ASCs show comparable migration and immunosuppressive capacity. (A) Cell migration ability of ND-ASCs (n=3) and T2DM-ASCs (n=3) at 0, 12 and 24 hours (40×magnification). (B) Statistical analysis of cell migration area by the ImageJ. (C) ND-ASCs (n=3) and T2DM-ASCs (n=3) cocultured with PBMCs for 3 days, flow cytometry analysis of PBMCs with CD3 staining. (D) Percentages of CD3+ lymphocyte proliferation. The data were shown as the mean±SEM, each experiment was repeated at least twice, one representative example shown. NS: not significant. **p<0.01, ***p<0.001.
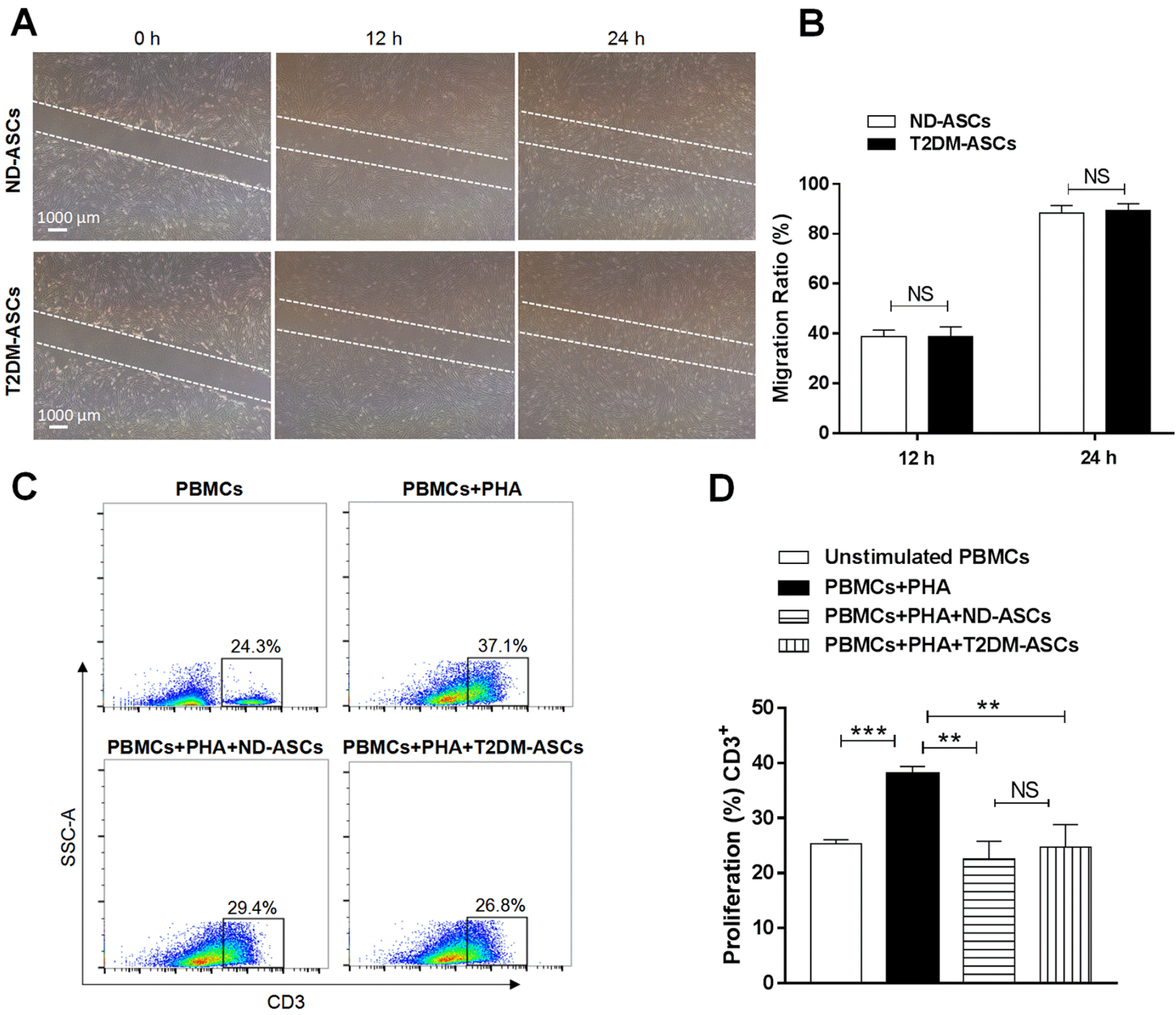
Fig. 5
The characteristics of cytokine expression and stemness of T2DM-ASCs and ND-ASCs. (A, B) The comparation of immunomodulation-associated factors (A) and islet function-related factors (B) between T2DM-ASCs (n=3) and ND-ASCs (n=3). (C) The comparation of stemness-associated gene expression (NANOG, POU5F1) between T2DM-ASCs (n=3) and ND-ASCs (n=3). The data were shown as the mean± SEM, each experiment was repeated at least twice. NS: not significant. **p<0.01, ***p<0.001.
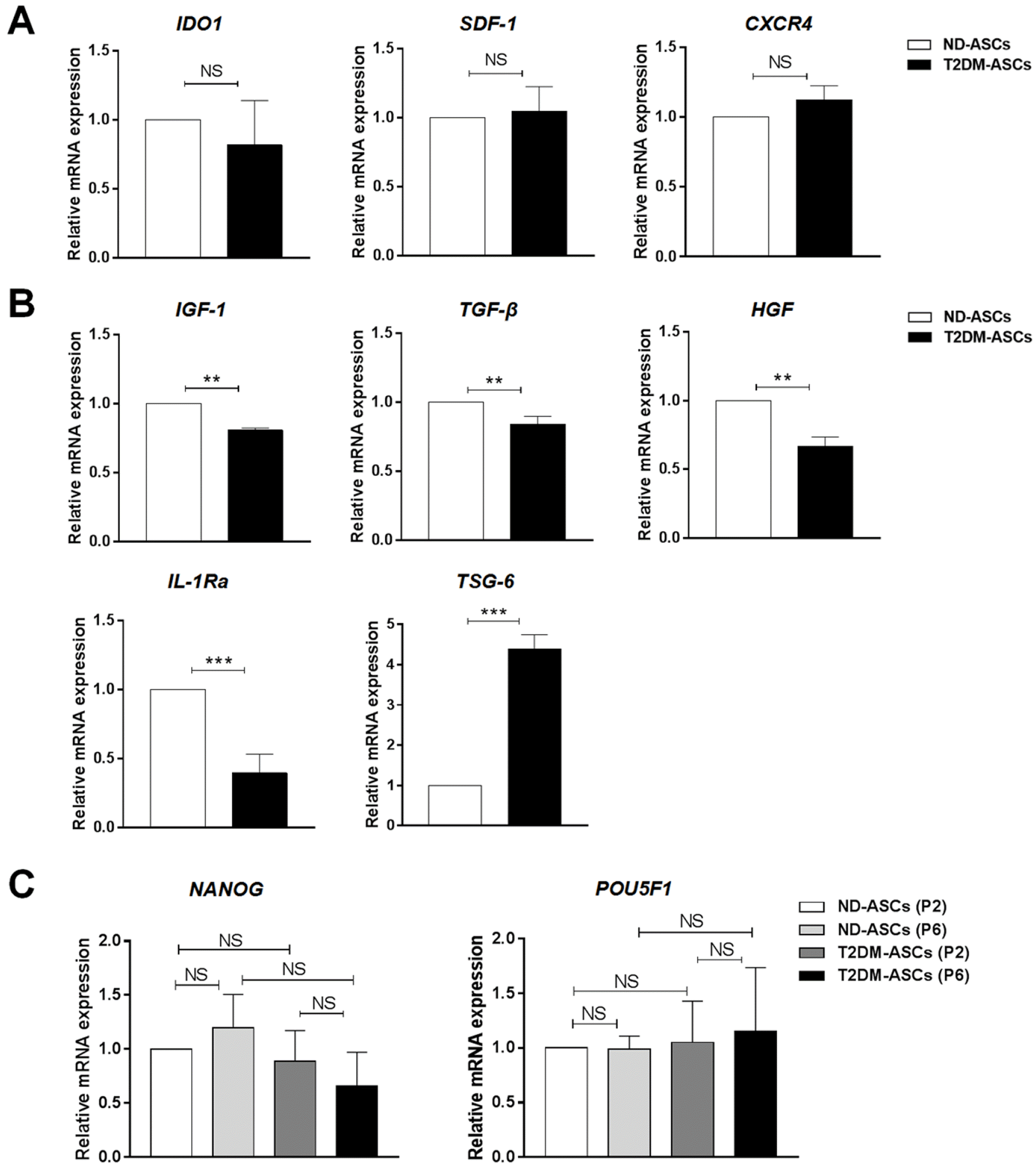
Table 1
Donor information
Age (y) | BMI (kg/m2) | HbA1c (%) | N (Male/Female) | |
---|---|---|---|---|
ND | 43.60±4.15 | 24.9±1.29 | 5.10±0.15 | 5 (3/2) |
T2DM | 50.33±1.33 | 27.17±0.48 | 7.03±0.66 | 3 (2/1) |
p | 0.276 | 0.244 | 0.047 |
Table 2
Human genes primer sequences for qRT-PCR