Abstract
Background and Objectives
The application of adipose derived stem cells (ADSCs) in skin repair has attracted much attention nowadays. Epidermal growth factor (EGF) participates in the progress of skin proliferation, differentiation and so forth. We aimed to explore the role of EGF in the proliferation, invasion, migration and transdifferentiation into epidermal cell phenotypes of ADSCs.
Methods and Results
ADSCs were extracted from adipose tissues from patient. Immunophenotyping was determined by flow cytometry. Overexpressed EGF or siEGF was transfected by lentiviruses. EGF was determined by enzyme linked immunosorbent assay (ELISA) or western blot. ADSCs and HaCaT cells were co-cultured by Transwell chambers. Conditioned medium (CM) was obtained from cultured HaCaT cells and used for the culturing of ADSCs. Cell viability was tested by 3-(4,5-dimethylthiazol-2-yl)-2,5-diphenyltetrazolium bromide (MTT) assay. Invasion rate was measured by Transwell invasion assay and migration rate by wound healing test. mRNA and protein levels were measured by qPCR and western blot respectively. The extracted cells from adipose tissues were identified as ADSCs by morphology and immunophenotyping. The expression of EGF was up or down regulated constantly in HaCaT cell line after transfection. EGF overexpression upregulated the proliferation, migration and invasion rates of ADSCs, and EGF expression regulated the expression of cytokeratin-19 (CK19) and integrin-β as well.
Severe skin tissue loss can be caused by trauma, extensive burns, diabetes or other chronic diseases (1). Currently, autologous, allogeneic skin grafts and artificial substitutes are commonly used in clinical practice, however, these methods have limited effects (1, 2), as problems such as new traumas in the donor area, limited donor sources and immune rejection still exist (1, 2). Therefore, skin wound repair remains an urgent issue to be solved (1, 2).
Recently, with the continuous development of stem cell technology, the application of mesenchymal stem cells in skin repair has attracted much attention (3). Adipose-derived stem cells (ADSCs) can be obtained from the human body through minimally invasive methods or from the excess adipose tissue in the body (4, 5). The source of ADSCs is abundant, and the culturing of ADSCs is easy in vitro (4, 5). The amount of ADSCs in the same tissue is much larger than that in the bone marrow mesenchymal stem cells, and ADSCs, which have good self-proliferation and multi-lineage differentiation potentials, can differentiate into tissue cells such as adipocytes, osteoblasts, hepatocytes and endothelial cells (4, 5). Brzoska et al. (6) reported that all-trans retinoic acid (ATRA) induced the differentiation of adipose-derived stem cells into epidermal cells, indicating that adipose-derived stem cells have the ability to differentiate into epidermal cells across the germ layer. Yao et al. (7) revealed that the transplantation of ADSCs could promote the healing of skin deep partial-thickness scald wound of rabbit. Thus, the usage of ADSCs in the repair of wound skin shows a promising effect.
Epidermal growth factor (EGF) is a type of polypeptide, which is composed of 53 amino acids and promoting mitosis (8). After hydrolysis, it exerts its biological activity on and participates in the progress of skin proliferation, differentiation, apoptosis and carcinogenesis (9). Previous studies had shown that EGF could induce the early development of teeth and eyelids in mice inhibit the secretion of gastric acid, as well as promote the growth of epidermis and the keratinization process (8). In addition, researchers also reported that ADSCs treated with EGF by three-dimensional culturing method would differentiate to an epithelial phenotype (10, 11). Therefore, further study of EGF in the tissue engineering has a scientific significance.
In view of above evidence, we aimed to explore the role of EGF produced by HaCaT cells in the proliferation, invasion, migration and transdifferentiation of ADSCs into epidermal cell phenotypes. Our study provides supporting evidences for ADSCs to be used as ideal seed cells in tissue engineering.
The protocol of this study was approved by the ethics board of Sun Yat-sen Memorial Hospital, Sun Yat-sen University (approval number: SY2017010745). Adipose tissue was collected from a female patient who aged 29 years old. The patient signed the informed consents before the study has been conducted. Under sterile conditions, about 10 g of the remaining abdominal subcutaneous adipose tissue of the patient who took the plastic surgery was sent to the laboratory within 1 h. The tissue was first immersed in PBS (Solarbio Life Sciences, Beijing, China) containing penicillin (300 U/ml) and streptomycin (300 μg/ml) (V900929-100 ml, Sigma, Shanghai, China) in a super clean bench for 10 min and then rinsed twice with 2× PBS for 5 min. Blood vessels and connective tissues were carefully removed using ophthalmic scissors, and the adipose tissue was cut into 1 mm×1 mm×1 mm size. The tissues were gathered in a flask and added with 10 ml of 0.1% type I collagenase (SCR103, Sigma, Shanghai, China), and they were vigorously shaken and digested in a water bath at 37℃ for 1 h. 10% FBS (10 ml) (10099141, Gibco, Shanghai, China) was added to neutralize collagenase, and the samples were centrifuged at 1500 round/min for 10 min in a high-speed centrifuge at low temperature. The upper adipose tissue and supernatant were discarded, and the samples were resuspended with DMEM (10569044, Thermo Fisher, Waltham, USA) medium and sieved, and further centrifuged at 1500 round/min for 10 min. Next, the supernatant was discarded, and the samples were resuspended with DMEM medium and then placed in a 25 cm2 cell culture flask and incubated with 5% CO2 at 37℃. The medium was exchanged after 24 h to remove residual red blood cells and unattached cells. Meanwhile, HaCaT cell line was obtained from the Type Culture Collection of the Chinese Academy of Sciences, Shanghai, China and cultured in DMEM Medium supplied with 10% FBS and 5% CO2 at 37℃. Medium was renewed every 2∼3 days. The cells were subcultured at a ratio of 1:3 when they reached the logarithmic growth phase by being digested with 0.125% trypsin-EDTA (25300054, Gibco, Shanghai, China) at 37℃. The cells were observed under a microscope (Olympus IX71, Tokyo, Japan) when cell density reached 80%∼90% of culture flask.
100 μl of 5×107/ml single cell suspension with 20 μl of fluorescently labeled mouse anti-human CD13 (CD13-PE, 560998, BD, Shanghai, China, 1:500), CD14 (CD14-APC, 561708, BD, Shanghai, China, 1:500), CD34 (CD34-PerCP, 340430, BD, Shanghai, China, 1:500), CD44 (CD44-APC, 559942, BD, Shanghai, China, 1:500), CD90 (CD90-FITC, 561969, BD, Shanghai, China, 1:500), and CD105 (CD105-PE, 560839, BD, Shanghai, China, 1:500) antibodies were added to a clean plastic tube and incubated at 4℃. The cells were then added with 2 ml PBS, mixed and centrifuged at 1500 round/min for 5 min. Next, the supernatant was discarded and 200 μl PBS was added to each tube and well mixed. The tubes were loaded on flow cytometry and tested. Data was analyzed by Cell-Quest software.
cDNAs encoding EGF, siEGF and negative control sequence (nonsense) were cloned into the lentiviral expression vectors pLenti6/CMV/V5-DEST (synthesized by Genepharma, Shanghai, China). The sequences of the EGF siRNA were 5’-AAUCCUUAUGAGGAG UCGA-3’ and 5’-CCACCACUAUUCCGUAAGA-3’. HaCaT cells were centrifuged at 1000 round/min for 5 min, resuspended by trypsin and then collected. 5×105 cells were added into 1 ml of the culture medium, resuspended and counted, and then transferred to a 24-well plate. The cells were further added a volume of virus solution at multiplicity of infection (MOI) of 40 with 500 μl of culture medium. Ploybrene transfection enhancer (No.107689, Sigma, Shanghai, China) at a ratio of 1:200 was added, and the cells were then incubated in an incubator. After 24 h, the virus-containing medium was removed, and fresh culture medium was added to continue the culturing. HaCaT cells were grouped as control, Mock, EGF, NC and siEGF groups. Control group was a blank control, and Mock, EGF, NC, and siEGF were treated with lentiviral vector, lentiviral vector with EGF, negative control lentiviral vector of siEGF and lentiviral vector with siEGF respectively.
After the transfection and culturing of HaCaT cells for 24 h, 48 h and 72 h respectively, the co-culturing of ADSCs and HaCaT cells for 14 days and the culturing of ADSCs with conditioned medium (CM) from modified HaCaT cells, the determination of EGF was then conducted according to the instructions given (ab100504, Abcam, Shanghai, China). Briefly, the serum-free supernatants of the cells were collected and 100 μl of the samples were added to a 96-well reaction plate. The plate was thoroughly mixed with the samples and incubated at 37℃ for 120 min. Thereafter, the plate was washed with washing liquid for 4∼6 times and dried on a filter paper, and then 100 μl of working solution with primary antibody was added to each well, and the plate was incubated at 37℃ for 60 min. The plate was then washed, and 100 μl the working solution with enzyme-labeled antibody was added to each well, and the plate was incubated at 37℃ for 60 min. 100 μl working solution with substrate was added to each well after the plate was washed, and then the plate was placed in a dark drawer at 37℃ for 15 min. 100 μl stop solution was added to each well, and the OD value at 450 nm was read on a microplate reader (Multiskan, Thermo, Waltham, USA).
The Transwell plate (Corning, USA) was used to establish a cell co-culture system. There were different groups as follows: control group (The upper layer had no cells, while the lower layer was inoculated with 4×104 ADSCs); HaCaT-Mock group (The upper layer was inoculated with HaCaT cells containing lentiviral vector, while the lower layer was inoculated with 4×104 ADSCs); HaCaT-EGF group (The upper layer was inoculated with HaCaT cells containing lentiviral vector with EGF, while the lower layer was inoculated with 4×104 ADSCs); HaCaT-NC group (The upper layer was inoculated with HaCaT cells containing negative control lentiviral vector of siEGF, while the lower layer was inoculated with 4×104 ADSCs); HaCaT-siEGF group (The upper layer was inoculated with HaCaT cells containing siEGF, while the lower layer was inoculated with 4×104 ADSCs). The cells were cultured for 14 days.
The cells of HaCat-Mock,HaCat-EGF,HaCat-NC, HaCat-siEGF groups were collected when the cell confluence reached more than 85%. The cells were then incubated in a serum-free medium for the next 48 h, and the supernatant was gathered. After the supernatant was filtered with 0.22 μm filter membrane, the cells were marked as CM of each group and stored at −80℃. ADSCs were cultured and grouped as follows: control group (cells with DMEM medium); Mock-CM group (cells with 50% DMEM and 50% CM from HaCat-Mock group); EGF-CM group (cells with 50% DMEM and 50% CM from HaCat-EGF group); NC-CM group (cells with 50% DMEM and 50% CM from HaCat-NC group); siEGF-CM group (cells with 50% DMEM and 50% CM from HaCat-siEGF group).
Cell viability was measured after ADSCs have been co-cultured with HaCaT cells for 14 days and after the ADSCs have been cultured CM from modified HaCaT cells for 24 h, 48 h, and 72 h respectively. MTT Cell Proliferation Assay Kit (ab211091, Abcam, Shanghai, China) was used to measure the cell viability. The cells were gathered and loaded into a 96-well plate when cell density reached 5×106 cells/ml. Medium was discarded and 50 μl of serum-free media and MTT Reagent were added into each well. The plate was incubated at 37℃ for 3 h. After the incubation, 150 μl of MTT Solvent was added into each well. The plate was then wrapped in foil and shaken on an orbital shaker for 15 min. Finally, OD at 590 nm was read on a microplate reader (Multiskan, Thermo, Waltham, USA).
Invasion rate was assessed by Transwell chambers (8 μm, BD Biosciences, CA, USA). Briefly, the cells were collected and loaded into the top chamber when cell density reached 2.5×104 cells/cm2. 200 μl medium with 10% FBS (10099158, Gibco, Shanghai, China) was added into bottom chamber. The chambers were incubated at 37℃ for 48 h. Cells that did not invade through the membrane pores were removed by swab from the top chamber. The cells that did not invade into the bottom chamber were fixed and stained with 0.5% crystal violet (C6158, Sigma, Shanghai, China) for 30 min. The cells were then counted under an inverted microscope (Olympus IX71, Tokyo, Japan) and photographed.
Cells were incubated on 6-well plates until being merged into a monolayer. Thereafter, 0.5 cm gap was made by a 50 μl pipette tip and the cells were cultured for 24 h. The cells were washed with PBS for 2 times after medium has been removed and then supplied with fresh medium. The cells were cultured for the next 24 h. The distances between the edges of the gaps were then measured. The healing of the gaps of the monolayer of the cells was photographed under an inverted microscope (Olympus IX71, Tokyo, Japan) at the same observation site.
Total RNA was extracted by Trizol (Invitrogen, Shanghai, China) and cDNA was synthesized by iScriptTM cDNA Synthesis Kit (Bio-Rad, CA, USA) following the instructions of manufacturers. qPCR was conducted using Fast Start Universal SYBR Green Master kit (Roche, Shanghai, China). The total volume of reaction system was 20 μl and prepared as follows: 2× SYBER Green master mix 10 μl, cDNA template 2 μl, forward primer (10 μM) 0.5 μl, reverse primer (10 μM) 0.5 μl, ddH2O 7 μl. Procedures of PCR were as follows: at 95℃ for 30 sec, at 95℃ for 15 sec, at 60℃ for 30 sec, and at 72℃ for 2 min, 40 cycles. PCR was performed in CFX96 TouchTM (#6093, Bio-Rad, CA, USA). Primers were listed in Table 1.
The cells were lysed by RIPA (89900, Thermo Fisher, Shanghai, China) and centrifuged at 4℃, 16000 g for 30 min following the instructions of manufacturer. The supernatant of cells was gathered and stored at −80℃. The concentration of total protein was determined by the PierceTM BCA Protein Assay Kit (Thermo Fisher, Waltham, USA). 25 μl samples and standard proteins were diluted to 1, 0.5, 0.25, 0.125, 0.0625 g/ml, respectively, and then loaded into the left column of a 96-well plate. 200 μl Working Regent was added to each well and mixed with the samples or standard proteins thoroughly on a plate shaker for 30 sec. The plate was covered and incubated at 37℃ for 30 min. The absorbance at 562 nm was measured on a microplate reader (Multiskan, Thermo, Waltham, USA). The concentration of total protein was calculated by EXCEL software according to the standard curve.
Samples were boiled at 95℃ for 15 min. Thereafter, proteins were separated by 10% SDS-PAGE electrophoresis at 100 V for 2 h. The proteins were then transferred at 90 V for 1.5 h onto a PVDF membrane and blocked with 5% non-fat milk for 1 h at RT. Primary antibody anti-EGF antibody (ab9695, Abcam, San Francisco, USA, 1:1000), anti-beta actin antibody (ab8227, Abcam, San Francisco, USA, 1:2000), anti-cytokeratin 19 antibody (ab15463, Abcam, San Francisco, USA, 1:1000), and anti-integrin-β1 antibody (ab179471, Abcam, San Francisco, USA, 1:2000) were incubated with the membrane in sealing bags at 4℃ overnight. The membrane was washed 3 times with PBST (Solarbio Life Sciences, Beijing, China) for 5 min each time. The secondary antibody IgG H&L (HRP) (ab6721, Abcam, San Francisco, USA, 1:2000) was then incubated with the membrane for 1 h at RT. The membrane was washed 3 times in PBST for 5 min once more and detected by PierceTM ECL plus western blotting substrate (Thermo Fisher, Waltham, USA). Blots of proteins were photographed and analyzed.
In order to distinguish ADSCs from primary cultured cells, the third-generation cells with good growth status were observed under an inverted phase contrast microscope and their immunophenotypings were determined by flow cytometry. As shown in the images (Fig. 1A), the cells were in two forms, one was the fibroblast-like cells that had long protruding feet at both ends, another was in a flat irregular or polygonal shape with a large protrusion. In addition, the fused cells were arranged in such a directional pattern as “swirl”. The outcomes of cytometry showed that CD13, CD44, CD90 and CD105 were highly expressed, while the CD14 and CD34 were lowly expressed (Fig. 1B), indicating that these cultured cells extracted from tissues could be confirmed as ADSCs.
To confirm that whether EGF was successfully overexpressed or suppressed in HaCaT cells and whether the effectiveness could last for a period of time, we measured the expression of EGF by qPCR, western blot and ELISA. The results showed that the expression of EGF in EGF group was much higher than that in mock group, and meanwhile, the expression of EGF in siEGF group was lower than that in NC group after the transfection (Fig. 2A and 2B, **p<0.01, ^^p<0.01). This effectiveness lasted at least 3 days (Fig. 2C, **p<0.01, ^^p<0.01), suggesting that EGF could be overexpressed or suppressed constantly in HaCaT cell line.
To investigate whether the expression of EGF could regulate the proliferation of ADSCs and promote the transdifferentiation of ADSCs into epithelial stem cell types, we measured the cell viability of ADSCs by MTT, determined the protein levels of EGF in the bottom chamber and assessed the expressions of CK19 and integrin-β in ADSCs. On one hand, the results from the co-cultured ADSCs and HaCaT cells showed that the cell viability in HaCaT-EGF group was higher than that in HaCaT-Mock group, however, no significance between HaCaT-NC and HaCaT-siEGF groups (Fig. 3A, *p<0.05) was observed. Besides, the protein level of EGF in HaCaT-Mock and HaCaT-NC groups were both higher than that in control group (Fig. 3B, **p<0.01, ##p<0.01, ^^p<0.01), indicating that ADSCs and HaCaT cells transfected with lentiviral vector or negative control lentiviral vector alone could produce EGF spontaneously. Moreover, the protein level of EGF in HaCaT-EGF group was higher than that in HaCaT-Mock group, while it was lower in HaCaT-siEGF group than in HaCaT-NC group (Fig. 3B, **p<0.01, ##p<0.01, ^^p<0.01). In addition, the results of the expressions of CK19 and integrin-β were consistent with the results of the protein levels of EGF (Fig. 3C∼E, **p<0.01, ##p<0.01, ^^p<0.01). On the other hand, the results of the culturing of ADSCs with CM revealed that there were significance between cell viability in EGF-CM group and that in Mock-CM group after 72 hours of culturing, however, unfortunately, no significance between NC-CM and siEGF-CM groups was identified (Fig. 4A, *p<0.05). The outcomes of protein levels of EGF (endogenous and secreted) as well as the expressions of CK19 and integrin-β were consistent with the results of the co-culturing of ADSCs and HaCaT cells (Fig. 4B∼F, **p<0.01, ##p<0.01, ^^p<0.01, #p<0.05, ^p<0.05). Taken together, these results suggested that the proliferation of ADSCs could be elevated by the overexpression of EGF, and the expressions of CK19 and integrin-β might be regulated by the expression of EGF.
To observe whether the capacity of migration and invasion in ADSCs could be affected by the expression of EGF, we tested the migration and invasion rates of ADSCs by wound healing test and Transwell invasion assay. The results showed that the invasion rate in EGF-CM group was higher than that in Mock-CM group (Fig. 5A, *p<0.05). Similarly, the migration rate in EGF-CM group was higher than Mock-CM group (Fig. 5B, *p<0.05). However, no significant difference was observed between NC-CM and siEGF-CM groups on both invasion and migration rates (Fig. 5A and 5B). Thus, we assumed that the overexpression of EGF was able to raise the invasion and migration rates of ADSCs.
In this study, we extracted and identified ADSCs successfully in the first place. Further, after up- and down-regulation of the expression of EGF in HaCaT cells, we explored the alteration of cell viability, migration, invasion and the expressions of CK19 and integrin-β by co-culturing ADSCs with modified HaCaT cells or their CMs. Our study demonstrated the a promising application of EGF in the treatment of skin wound repair using ADSCs.
We first identified the morphology and immunophenotyping of the extracted and cultured cells from adipose tissues. According to the reports, the mature ADSCs would shape-like fibroblast cellsthat have long protruding feet at both ends or are in a flat irregular or polygonal shape with a large protrusion (12). With the extension of cell culture, the fused cells would be arranged as a “swirl” (12). In general, the cells we extracted were as previously described. This evidence, on one hand, confirmed that the cells were ADSCs. Nowadays, it is believed that the positive biomarkers of ADSCs were CD13, CD44, CD90, CD105, while the negative biomarker was CD14 (13). However, the expression of CD34 was still controversial (13, 14). Our results showed that the relative expression of CD13, CD44, CD90 and CD105 were high, while CD14 and CD34 were low. On the other hand, we verified the cells were ADSCs to some extent. Taken together, we affirmed that these cells extracted and cultured from adipose tissues were ADSCs, and further experiments could be continued.
HaCaT cells are a type of human immortalized epidermal cell line with the characteristics of epidermal basal cells, which are the same as primary keratinocytes, with stable genetic characteristics but without tumor characteristics (15). HaCaT cell line can replace human normal keratinocytes and is an ideal cell model in the research of skin diseases (15). The cell was selected under the consideration that HaCaT cells being able to continuously express the gene of interest was of great significance in the induction of skin formation in tissue engineering. As expected, we successfully overexpressed and suppressed EGF in HaCaT cells, and the outcome could last for at least 3 days. Tao et al. (16) demonstrated that HaCaT-EGF cells could be constructed as tissue engineering seed cells by pcDNA3.1-EGF eukaryotic expression vector. Hu et al. (17) suggested that HaCaT cells modified with human EGF gene could be promising seed cells for building skin substitute. Thus, in this sense, we build a model of EGF-HaCaT seed cells as the basics for further exploration.
Under physiological and pathological conditions, complex interactions between somatic cells as well as between cells and extracellular environment (18) exist. Compared with single-cell culture, the co-culture system can better simulate the environment in vivo and is closer to the somatic characteristics of cells, thus, it is convenient to observe the interactions between cells and cells as well as between cells and extracellular environment (18). Stem cells conditional medium contains a variety of cellular active substances secreted from them, and is easy to be freeze-dried, concentrated, preserved and transported, therefore, it became a research hotspot in the field of regenerative medicine and cell therapy (19-21). By co-culturing modified HaCaT and ADSCs as well as the culturing of ADSCs with CM from modified HaCaT, we revealed that EGF could be able to raise the proliferation of ADSCs, though there was no effect observed after the EGF has been suppressed. Mvula, B. et al. reported that EGF could elevate the proliferation of ADSCs (22). Jia et al. (23) revealed that EGF promoted the proliferation of fibroblasts differentiated from ADSCs. It suggested that EGF could elevate the proliferation of ADSCs. Integrins, a class of glycoprotein receptor family molecules located on the surface of cell membranes, are heterodimers, including α and β subunits (24). During the directional differentiation of basal cells, integrin-β loses its expression until the cells completely leave the basal layer (24). Thus, integrin-β can be used as a surface marker of epidermal like cells (24, 25). Keratin is a structural protein of epidermal cells that constitute microfilaments with a diameter of 10 nm and form a broad network in the cell (26). There are no less than 20 different types of keratin expression in the epithelial tissue of the human body (26). As it has different degrees of differentiation, epidermal cells express different keratin, keratin can also be used as a means of identification of stem cells, committed progenitor cells and differentiated cells (26). Epidermal like cell expresses CK19, hence it can be seen as markers for epidermal stem cells (26). Our results revealed that the expressions of CK19 and integrin-β were elevated using the method of co-culturing modified HaCaT and ADSCs and the culturing of ADSCs with CM from modified HaCaT. Early in 1999, a report showed that the function of integrin-β1 could be stimulated by EGF in metastatic breast carcinoma cell line MDA-MB-435 (27). Moreover, Yoneda et al. (28) reported that EGF induced CK19 expression with increased growth abilities in human hepatocellular carcinoma. Makarova et al. (29) demonstrated a dose-dependent increase of CK19 expression after EGF treatment in UT-SCC-26A cells. Therefore, we speculated that the expression of EGF could regulate the expressions of CK19 and integrin-β, showing the regulatory role in the induction of transdifferentiating ADSCs into epidermal like cells.
Our results showed that the invasion and migration rates of ADSCs could be elevated by the culturing of ADSCs with CM from modified HaCaT. However, the CM of HaCaT with suppressed expression of EGF did not show significant effect on the invasion or migration of ADSCs. However, there might be a limitation not inhibiting proliferation ability when studying migration ability, which would be further studied. Recently, Chen et al. (30) reported that EGF released from human platelets promoted the invasion, migrationand epithelial mesenchymal transition (EMT) of oral squamous cell carcinoma. Tumur et al. (31) showed that EGF stimulated the migration and invasion of head and neck squamous cell carcinoma. Thus, it suggested that the overexpression of EGF could elevate the migration and invasion rates of ADSCs, which would accelerate the healing of skin wound. However, further study is required to determine the role of suppressed EGF.
In conclusion, EGF overexpressed HaCaT cells could be served as a stimulus to promote the proliferation, migration, and invasion and the transdifferentiation into epidermal stem cell immunophenotyping of ADSCs. Our findings suggested that EGF has a promising effect on the repair of skin wound. It provided evidence for HaCaT cells becoming mother-cells in the tissue engineering. The deeper mechanism would be studied in the future, including the expression of EGFR and EGF related signals etc.
Acknowledgments
This work was supported by Project of Guangdong Sceience and Technology Department [Grant number: 2013B0218002].
Notes
Author Contributions
Substantial contributions to conception and design: YM, JM. Data acquisition, data analysis and interpretation: YX, XX. Drafting the article or critically revising it for important intellectual content: YM, JM, XX. Final approval of the version to be published: All authors. Agreement to be accountable for all aspects of the work in ensuring that questions related to the accuracy or integrity of the work are appropriately investigated and resolved: YX, XX.
References
1. Inzucchi SE, Bergenstal RM, Buse JB, Diamant M, Ferrannini E, Nauck M, Peters AL, Tsapas A, Wender R, Matthews DR. 2015; Management of hyperglycaemia in type 2 diabetes, 2015: a patient-centred approach. Update to a position statement of the American Diabetes Association and the European Association for the study of diabetes. Diabetologia. 58:429–442. DOI: 10.1007/s00125-014-3460-0. PMID: 25583541.


2. Takeo M, Lee W, Ito M. 2015; Wound healing and skin regeneration. Cold Spring Harb Perspect Med. 5:a023267. DOI: 10.1101/cshperspect.a023267. PMID: 25561722. PMCID: PMC4292081.


3. Sharma RR, Pollock K, Hubel A, McKenna D. 2014; Mesenchymal stem or stromal cells: a review of clinical applications and manufacturing practices. Transfusion. 54:1418–1437. DOI: 10.1111/trf.12421. PMID: 24898458. PMCID: PMC6364749.


4. Skubis A, Gola J, Sikora B, Hybiak J, Paul-Samojedny M, Mazurek U, Łos MJ. 2017; Impact of antibiotics on the proliferation and differentiation of human adipose-derived mesenchymal stem cells. Int J Mol Sci. 18:E2522. DOI: 10.3390/ijms18122522. PMID: 29186789. PMCID: PMC5751125.


5. Naderi N, Combellack EJ, Griffin M, Sedaghati T, Javed M, Findlay MW, Wallace CG, Mosahebi A, Butler PE, Seifalian AM, Whitaker IS. 2017; The regenerative role of adipose-derived stem cells (ADSC) in plastic and reconstructive surgery. Int Wound J. 14:112–124. DOI: 10.1111/iwj.12569. PMID: 26833722.


6. Brzoska M, Geiger H, Gauer S, Baer P. 2005; Epithelial differentiation of human adipose tissue-derived adult stem cells. Biochem Biophys Res Commun. 330:142–150. DOI: 10.1016/j.bbrc.2005.02.141. PMID: 15781243.


7. Yao YM, Yan H, Zhang ZM, Wu CF, Zhang L, Yang BB. 2016; [Effects of rabbit adipose-derived mesenchymal stem cells on the healing of skin deep partial-thickness scald wound of rabbit]. Zhonghua Shao Shang Za Zhi. 32:402–407. DOI: 10.3760/cma.j.issn.1009-2587.2016.07.004. PMID: 27464630. Chinese.
8. Swindle CS, Tran KT, Johnson TD, Banerjee P, Mayes AM, Griffith L, Wells A. 2001; Epidermal growth factor (EGF)-like repeats of human tenascin-C as ligands for EGF receptor. J Cell Biol. 154:459–468. DOI: 10.1083/jcb.200103103. PMID: 11470832. PMCID: PMC2150768.


9. Alexandrescu DT, Kauffman CL, Dasanu CA. 2009; The cutaneous epidermal growth factor network: can it be translated clinically to stimulate hair growth? Dermatol Online J. 15:1. PMID: 19379645.


10. Long JL, Zuk P, Berke GS, Chhetri DK. 2010; Epithelial differentiation of adipose-derived stem cells for laryngeal tissue engineering. Laryngoscope. 120:125–131. DOI: 10.1002/lary.20719. PMID: 19856398.


11. Li H, Xu Y, Fu Q, Li C. 2012; Effects of multiple agents on epithelial differentiation of rabbit adipose-derived stem cells in 3D culture. Tissue Eng Part A. 18:1760–1770. DOI: 10.1089/ten.tea.2011.0424. PMID: 22497213. PMCID: PMC3432900.


12. Fève B. 2005; Adipogenesis: cellular and molecular aspects. Best Pract Res Clin Endocrinol Metab. 19:483–499. DOI: 10.1016/j.beem.2005.07.007. PMID: 16311213.


13. Bourin P, Bunnell BA, Casteilla L, Dominici M, Katz AJ, March KL, Redl H, Rubin JP, Yoshimura K, Gimble JM. 2013; Stromal cells from the adipose tissue-derived stromal vascular fraction and culture expanded adipose tissue-derived stromal/stem cells: a joint statement of the International Federation for Adipose Therapeutics and Science (IFATS) and the International Society for Cellular Therapy (ISCT). Cytotherapy. 15:641–648. DOI: 10.1016/j.jcyt.2013.02.006. PMID: 23570660. PMCID: PMC3979435.


14. Jiang A, Li M, Duan W, Dong Y, Wang Y. 2015; Improvement of the survival of human autologous fat transplantation by adipose-derived stem-cells-assisted lipotransfer combined with bFGF. ScientificWorldJournal. 2015:968057. DOI: 10.1155/2015/968057. PMID: 25695105. PMCID: PMC4324956.


15. Schoop VM, Mirancea N, Fusenig NE. 1999; Epidermal organization and differentiation of HaCaT keratinocytes in organotypic coculture with human dermal fibroblasts. J Invest Dermatol. 112:343–353. DOI: 10.1046/j.1523-1747.1999.00524.x. PMID: 10084313.


16. Tao K, Bai XZ, Zhang ZF, Shi JH, Hu XL, Tang CW, Hu DH, Han JT. 2013; Construction of the tissue engineering seed cell (HaCaT-EGF) and analysis of its biological characteristics. Asian Pac J Trop Med. 6:893–896. DOI: 10.1016/S1995-7645(13)60159-5. PMID: 24083586.


17. Hu DH, Zhang ZF, Zhang YG, Zhang WF, Wang HT, Cai WX, Bai XZ, Zhu HY, Shi JH, Tang CW. 2012; A potential skin substitute constructed with hEGF gene modified HaCaT cells for treatment of burn wounds in a rat model. Burns. 38:702–712. DOI: 10.1016/j.burns.2011.12.014. PMID: 22360951.


18. Paschos NK, Brown WE, Eswaramoorthy R, Hu JC, Athanasiou KA. 2015; Advances in tissue engineering through stem cell-based co-culture. J Tissue Eng Regen Med. 9:488–503. DOI: 10.1002/term.1870. PMID: 24493315.


19. Pawitan JA. 2014; Prospect of stem cell conditioned medium in regenerative medicine. Biomed Res Int. 2014:965849. DOI: 10.1155/2014/965849. PMID: 25530971. PMCID: PMC4229962.


20. Sugitani S, Tsuruma K, Ohno Y, Kuse Y, Yamauchi M, Egashira Y, Yoshimura S, Shimazawa M, Iwama T, Hara H. 2013; The potential neuroprotective effect of human adipose stem cells conditioned medium against light-induced retinal damage. Exp Eye Res. 116:254–264. DOI: 10.1016/j.exer.2013.09.013. PMID: 24076412.


21. Satarian L, Javan M, Kiani S, Hajikaram M, Mirnajafi-Zadeh J, Baharvand H. 2013; Engrafted human induced pluripotent stem cell-derived anterior specified neural progenitors protect the rat crushed optic nerve. PLoS One. 8:e71855. DOI: 10.1371/journal.pone.0071855. PMID: 23977164. PMCID: PMC3747054.


22. Mvula B, Moore TJ, Abrahamse H. 2010; Effect of low-level laser irradiation and epidermal growth factor on adult human adipose-derived stem cells. Lasers Med Sci. 25:33–39. DOI: 10.1007/s10103-008-0636-1. PMID: 19172344.


23. Jia YY, Zhou JY, Chang Y, An F, Li XW, Xu XY, Sun XL, Xiong CY, Wang JL. 2018; Effect of optimized concentrations of basic fibroblast growth factor and epidermal growth factor on proliferation of fibroblasts and expression of collagen: related to pelvic floor tissue regeneration. Chin Med J (Engl). 131:2089–2096. DOI: 10.4103/0366-6999.239301. PMID: 30127219. PMCID: PMC6111681.


24. Suzuki Y, Yanagisawa M, Yagi H, Nakatani Y, Yu RK. 2010; Involvement of beta1-integrin up-regulation in basic fibroblast growth factor- and epidermal growth factor-induced proliferation of mouse neuroepithelial cells. J Biol Chem. 285:18443–18451. DOI: 10.1074/jbc.M110.114645. PMID: 20371608. PMCID: PMC2881770.


25. Watt FM. 1998; Epidermal stem cells: markers, patterning and the control of stem cell fate. Philos Trans R Soc Lond B Biol Sci. 353:831–837. DOI: 10.1098/rstb.1998.0247. PMID: 9684280. PMCID: PMC1692275.


26. Michel M, Török N, Godbout MJ, Lussier M, Gaudreau P, Royal A, Germain L. 1996; Keratin 19 as a biochemical marker of skin stem cells in vivo and in vitro: keratin 19 expressing cells are differentially localized in function of anatomic sites, and their number varies with donor age and culture stage. J Cell Sci. 109(Pt 5):1017–1028. PMID: 8743949.


27. Adelsman MA, McCarthy JB, Shimizu Y. 1999; Stimulation of beta1-integrin function by epidermal growth factor and heregulin-beta has distinct requirements for erbB2 but a similar dependence on phosphoinositide 3-OH kinase. Mol Biol Cell. 10:2861–2878. DOI: 10.1091/mbc.10.9.2861. PMID: 10473632. PMCID: PMC25524.


28. Yoneda N, Sato Y, Kitao A, Ikeda H, Sawada-Kitamura S, Miyakoshi M, Harada K, Sasaki M, Matsui O, Nakanuma Y. 2011; Epidermal growth factor induces cytokeratin 19 expression accompanied by increased growth abilities in human hepatocellular carcinoma. Lab Invest. 91:262–272. DOI: 10.1038/labinvest.2010.161. PMID: 20856226.


29. Makarova G, Bette M, Schmidt A, Jacob R, Cai C, Rodepeter F, Betz T, Sitterberg J, Bakowsky U, Moll R, Neff A, Sesterhenn A, Teymoortash A, Ocker M, Werner JA, Mandic R. 2013; Epidermal growth factor-induced modulation of cytokeratin expression levels influences the morphological phenotype of head and neck squamous cell carcinoma cells. Cell Tissue Res. 351:59–72. DOI: 10.1007/s00441-012-1500-y. PMID: 23111772.


30. Chen R, Jin G, Li W, McIntyre TM. 2018; Epidermal Growth Factor (EGF) autocrine activation of human platelets promotes EGF receptor-dependent oral squamous cell carcinoma invasion, migration, and epithelial mesenchymal transition. J Immunol. 201:2154–2164. DOI: 10.4049/jimmunol.1800124. PMID: 30150285. PMCID: PMC6143412.


31. Tumur Z, Katebzadeh S, Guerra C, Bhushan L, Alkam T, Henson BS. 2015; RhoC mediates epidermal growth factor-stimulated migration and invasion in head and neck squamous cell carcinoma. Neoplasia. 17:141–151. DOI: 10.1016/j.neo.2014.12.002. PMID: 25622907. PMCID: PMC4309735.


Fig. 1
ADSCs were identified by morphology and immunophenotyping. (A) The representative images of ADSCs (×100, 200). (B) ADSCs were identified by immunophenotyping, with the detection of CD13, CD14, CD34, CD44, CD90, and CD105.
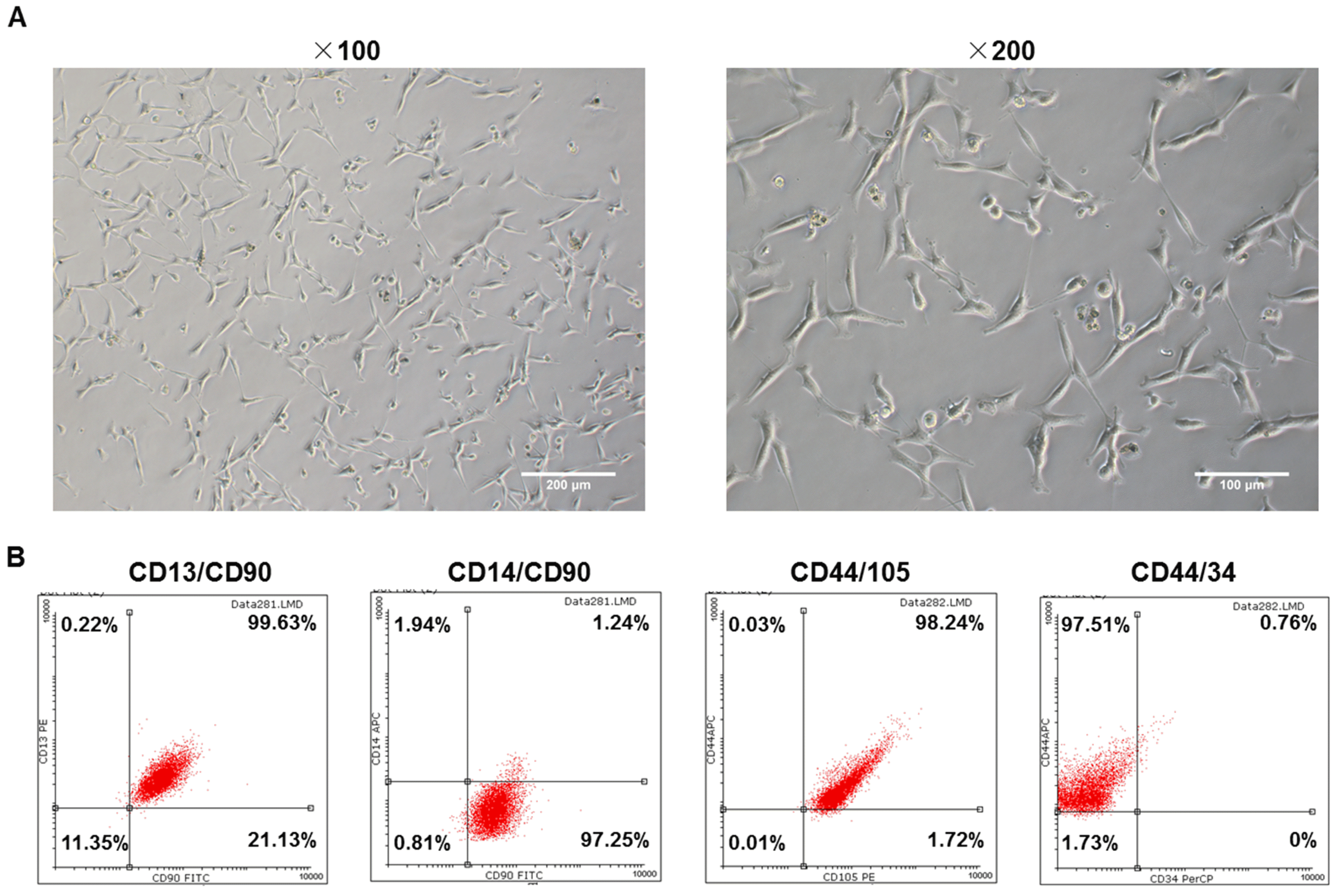
Fig. 2
The expression of EGF after EGF or siEGF transfection into HaCaT cells was detected. (A) Relative mRNA levels of EGF (of control) in each group were detected using RT-qPCR. (B) Relative protein levels of EGF (of β-actin) in each group were detected using Western blot. (C) Protein levels of excretive EGF (pg/ml) within 3 days in each group were detected using ELISA. Bars indicated means±SD. **p<0.01 vs. Mock group; ^^p<0.01 vs. NC group.
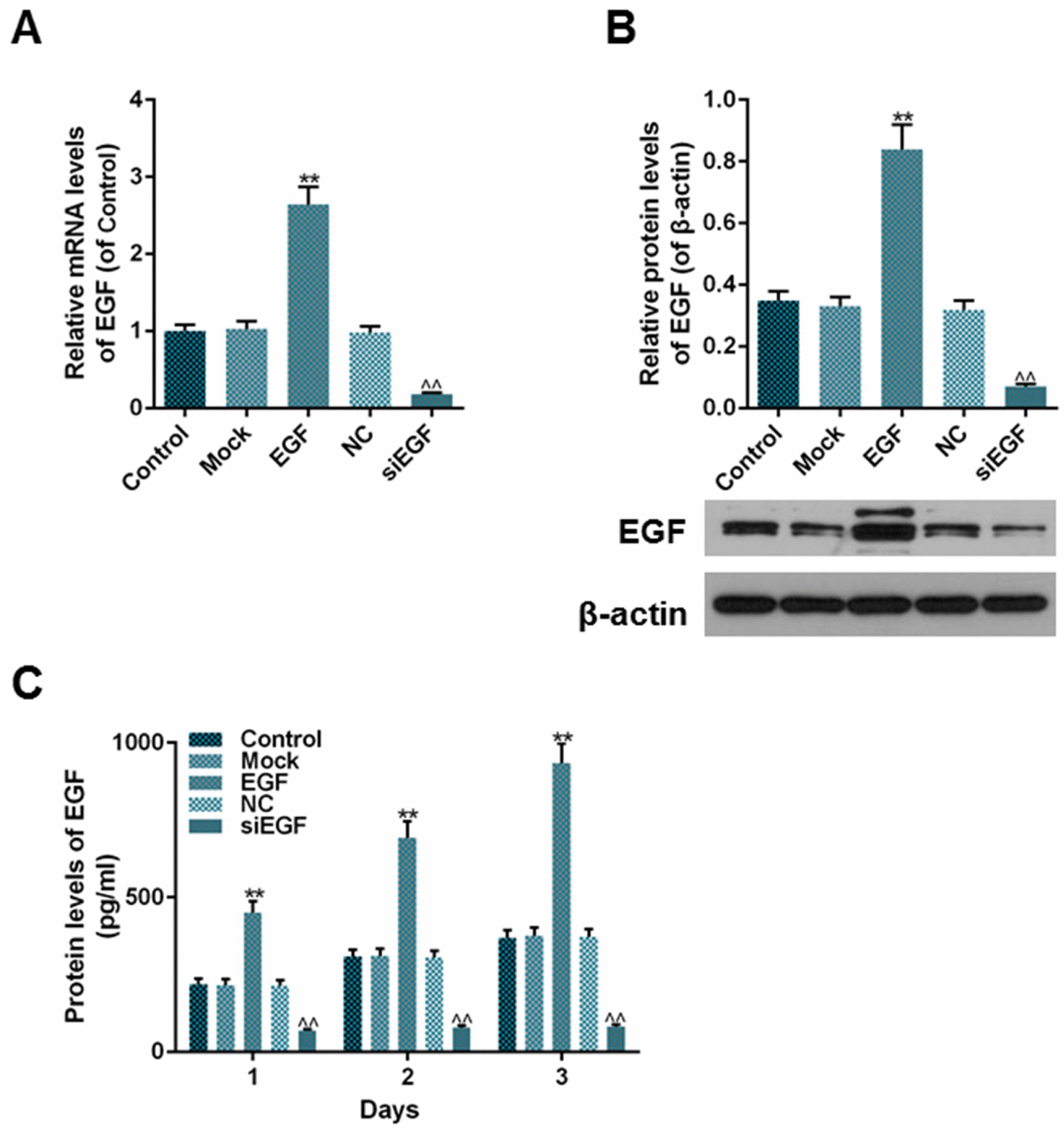
Fig. 3
Effect of EGF expression in HaCat on the viability of ADSCs. (A) Cell viability of ADSCs was detected using MTT assay. (B) Protein levels of excretive EGF (pg/ml) were detected using ELISA. (C) Relative mRNA levels (of control) of CK19 and integrin-β were detected using RT-qPCR. (D, E) Relative protein levels (of β-actin) of CK-19 and integrin-β were detected using Western blot. Bars indicated means±SD. **p<0.01 and *p<0.05 vs. HaCaT-Mock group; ##p<0.01 and #p<0.05 vs. control group; ^^p<0.01 and ^p<0.05 vs. HaCat-siEGF group.
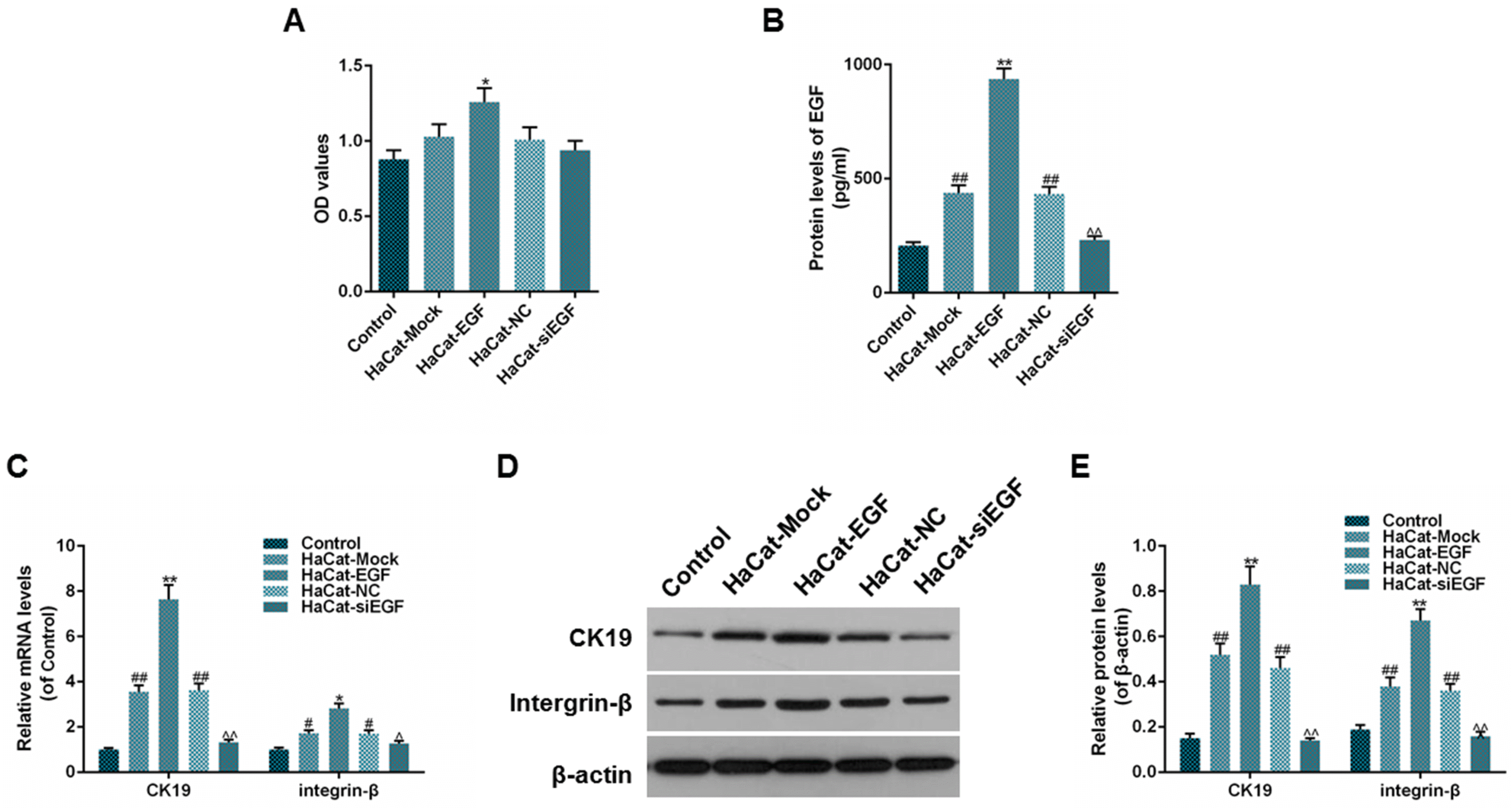
Fig. 4
Effect of EGF-containing medium of EGF-expressed HaCat cells on the viability of ADSCs. (A) Cell viabilities of ADSCs after 24, 48, 72 h of culturing were detected using MTT assay. (B) Protein levels of excretive EGF (pg/ml) were detected using ELISA. (C) Relative protein levels (of β-actin) of EGF were detected using Western blot. (D) Relative mRNA levels (of control) of CK19 and integrin-β were detected using RT-qPCR. (E, F) Relative protein levels (of β-actin) of CK-19 and integrin-β were detected using Western blot. Bars indicated means±SD. **p<0.01 and *p<0.05 vs. Mock-CM group; ##p<0.01 vs. control group; ^^p<0.01 and ^p<0.05 vs. siEGF-CM group.
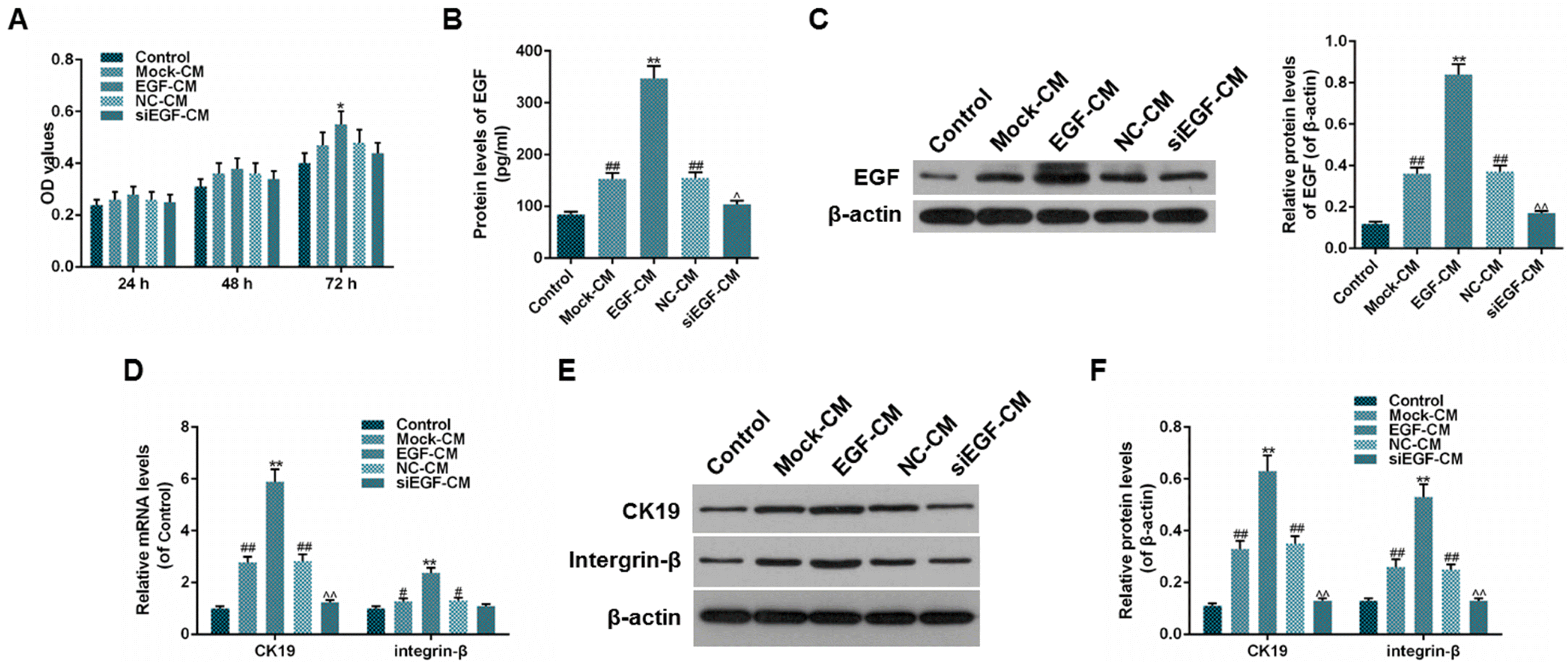
Fig. 5
Effect of EGF-containing medium of EGF-expressed HaCat cells on invasion and migration rates of ADSCs. (A) Cell invasion rates were detected using transwell assay. (B) Cell migration rates were were detected using wound healing assay. Bars indicated means±SD. *p<0.05 vs. Mock-CM group.
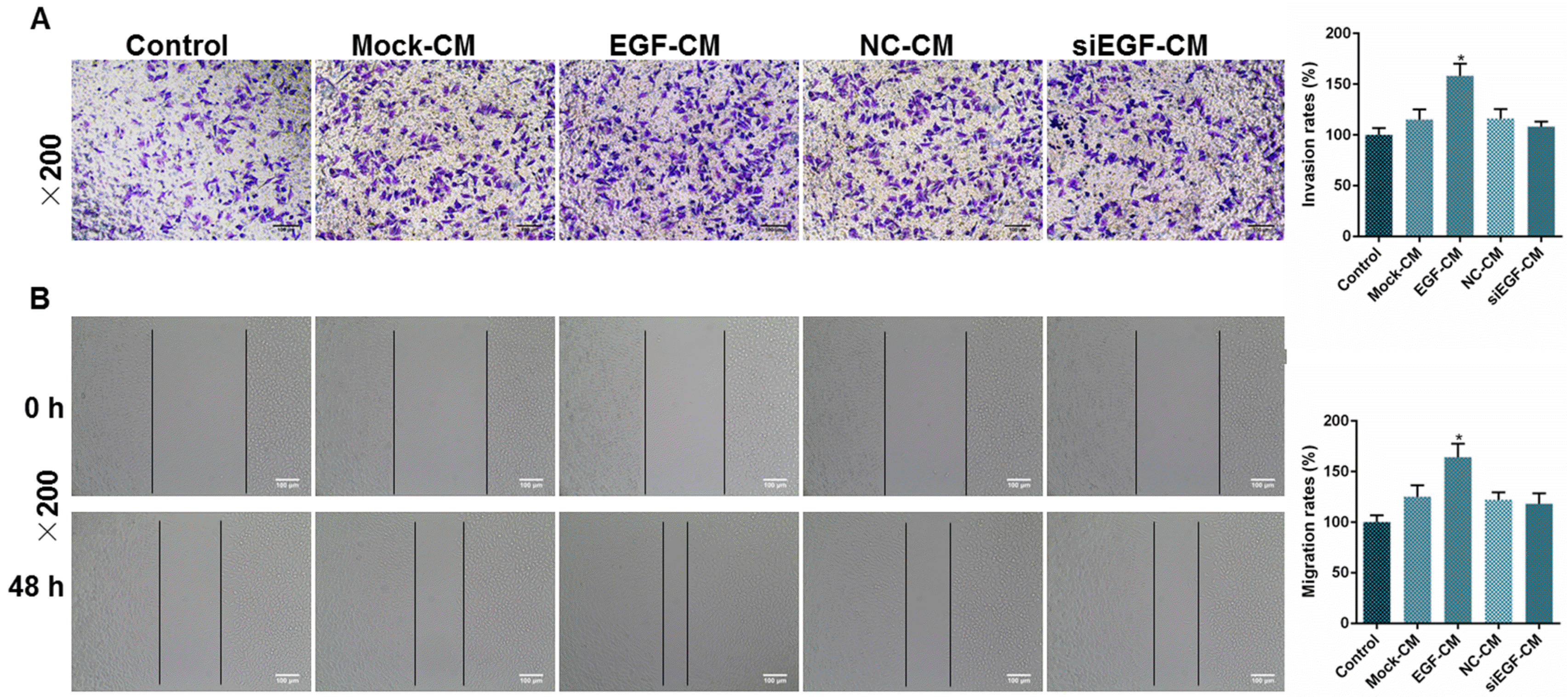
Table 1
Primers used in quantitative PCR