Abstract
Hematopoietic stem cells (HSCs) are regarded as one of essential cell sources for treating regenerative diseases. Among many stem cells, the feasibility of using adult-derived hematopoietic stem cells in therapeutic approaches is very diverse, and is unarguably regarded as an important cell source in stem cell biology. So far, many investigators are exploring HSCs and modified HSCs for use in clinical and basic science. In the present review, we briefly summarized HSCs and their application in pathophysiologic conditions, including non-hematopoietic tissue regeneration as well as blood disorders. HSCs and HSCs-derived progenitors are promising cell sources in regenerative medicine and their contributions can be properly applied to treat pathophysiologic conditions. Among many adult stem cells, HSCs are a powerful tool to treat patients with diseases such as hematologic malignancies and liver disease. Since HSCs can be differentiated into diverse progenitors including endothelial progenitors, they may be useful for constructing strategies for effective therapy.
Hematopoietic stem cells (HSCs) are multipotent primitive cells that can develop into all types of blood cells, including myeloid-lineage and lymphoid-lineage cells (1). HSCs can be found in several organs, such as peripheral blood (PB), bone marrow (BM), and umbilical cord blood (UCB). All blood cell lineages are produced via functional maturation of a rare population of multipotent HSCs that can proliferate by self-renewal and differentiation. Thus, understanding the molecular mechanisms regulating the self-renewal and cell fate determination of HSCs/progenitor cells is important for the development of clinical applications based on disease type and severity. Only a small population of HSCs is required to initiate the entire hematopoietic process. Since Cheng et al. (2) first reported that HSC quiescence is maintained by p21cip1/waf1 using p21−/− mice, numerous papers have found that long term-hematopoietic stem cells (LT-HSC) quiescence and activation are regulated by the genetic and epigenetic regulation of key molecules as well as by microenvironmental factors (3, 4). In addition to the attribute of self-renewal, a characteristic that distinguishes HSCs from other mature cells is the ability to undergo specific and large-scale differentiation into cells of various lineages. These findings from a colony-forming unit (CFU) assay also revealed HSC activity, and further measured the capacity of HSCs to produce mature blood cells in humans following myeloablation. Similar with LT-HSC, numerous papers has been studied the properties of short term HSC (ST-HSC) and progenitors and addressed that HSC/progenitors can directly or indirectly contributes in regenerating tissues and blood disorders. Based on these literatures, we described the available sources of HSCs, aging HSCs, and plasticity of HSCs in scientific information and clinical application of HSCs in blood disorders and liver deficiency.
All lineage blood cells are produced by a rare population of multipotent HSC, which can proliferate by self-renewal and differentiate to accomplish the functional maturation. From HSC to mature cells, there are several intermediate progenitor cells (5). These cells can display both functional multipotent and lineage-committed properties simultaneously or separately prior to complete maturation. Blood is a highly regenerative tissue due to its short life span, and BM supports the dynamic movement of diverse cells to maintain homeostasis of blood cells. Approximately, one trillion cells are generated daily to compensate apoptotic cells in human BM, suggesting rapid circulation of blood cells. HSC can be divided into LT-HSC, ST-HSC, and multipotent progenitor (MPP) in terms of duration of repopulation (6). In normal physiological conditions, rare HSC populations such as LT-HSC can develop into all lineage blood cells in the BM. However, HSC populations in the PB tend to be higher in myelosuppressive conditions caused by drug and granulocyte colony-stimulating factor (G-CSF), and rapidly migrate from the BM, suggesting different properties. HSC/progenitor cells in the PB are ST-HSC, which might directly contribute to recovering damaged tissues, and these are regarded as optimal curative cell sources in regenerative medicine. Blood cells from HSCs are divided into two lineages: lymphoid cells and myeloid cells (7). The lymphoid branch consists of T, B, and natural killer (NK) cells, which are relevant to innate and adaptive immune cells. This process is known lymphopoiesis. Myeloid lineage cells include all blood cells except lymphoid cells. There are several types of cells including monocytes from monoblasts, erythrocytes from erythroblasts, platelets from megakaryocytes, and granulocytes, which consist of neutrophils, eosinophils, and basophils, and are from myleoblasts. Lineage committed progenitor cells are specified for cell fate and develop into mature cells through myelopoiesis. In myeloid lineage cells, committed myeloid progenitors can be converted into mature types of myeloid cells. Lymphoid cells also have a similar process as the myeloid cells for generating progenitor cells and lymphoblast are from committed lymphoid progenitor cells. These blood cells are affected by dynamic niche composition including hormone and pericytes in BM (8). Similar to stem cells, progenitor cells in each step can also function as a strong cell source in regenerative medicine (Fig. 1). Thus, understanding HSC/progenitor cells is important to apply these cells in regenerative medicine depending on the disease type and severity. Thomas et al. (9, 10) reported that human BM cells infused into irradiation and chemotherapy receiving patients, resulted in the recovery of white blood cells and increased hemoglobin level. Two decades ago, existence HSC/progenitor cells in vivo is proved by advanced technology such as retroviral skill and clinic application using HSC has been continuously developed with exclusive demand. Remarkably, Till and Mcculloch (1) showed a colony forming unit (CFU) assay that can quantify HSC/progenitor cells; because HSC/primitive progenitor cells can repopulate through self-renewal, regenerative HSCs can be assayed with clonal expansion to generate multipotent HSCs. Moreover, this method further motivated scientist to develop ex vivo expansion for HSCs. Acquiring high number of HSCs/progenitors is a critical issue in regenerative medicine in order to overcome the limitations of cell sources. Besides attributes of self-renewal, a distinguishing feature of HSC aggregation from other mature cells is its specific differentiation with huge numbers into various lineage cells. These findings from the CFU assay also revealed LT-HSC activity, which further quantified HSC in producing mature blood cells in patients with myeloablation. Ultimately, we know that self-renewal proliferation of HSCs and differentiation into committed cell lineages through intermediate stages including progenitors are pivotal properties of HSCs. Because rare populations of HSC can lead the entire hematopoiesis, knowing the properties of HSC is very important to enhance therapeutic effects in clinical application. Recently, aging is emerging as an issue in HSC biology. Since Morrison et al. (11) mentioned homing and engraftment of HSCs based on aging, many papers have demonstrated the relevance of aging by DNA damage in HSC and molecules such as ATR gene and Cdc42 are pivotal to maintain phenotypes without HSC loss and back to rejuvenation (12). The immune system is orchestrated in terms of coordination of adaptive immune cells by lymphoid lineage cells including T and B cells and innate immune cells by myeloid cells. During aging, there is notable reduction in lymphocytes, especially naïve T cells, which are apparent, as well as the accumulation and clonal expansion of memory T cells (13). Meanwhile, myeloid lineage cells in BM seem to be increased or persist at the same level in an age-dependent manner, which leads to a proinflammatory environment (14). Thus, identification and isolation of bona fide HSCs with specific indicators should be demanded prior to experiments to address HSCs by aging. Until now, identification of HSCs using CD markers has advanced and the technique for isolation with high purity has been improved.
Research reports have consistently showed that understanding the properties of HSCs is very important for enhancing their therapeutic effects in clinical applications. Aging is relevant to the functional decline of normal HSCs as well as increased risk of hematologic malignancies (15). Abnormal clonal hematopoiesis which frequently occurs in the HSCs of the elderly is caused by DNA mutation in specific loci and shortening telomerase (16). Besides, there are several emerging intrinsic factors such as increased polarity, reactive oxygen species, and injured autophagy and mitochondria metabolism as ample evidence of aging of HSCs (17), which show the main features of aged HSCs compared to that of young HSCs. Especially, Mohrin et al. (17) showed the reversible contributing factor, deregulation of a UPR(mt)-mediated metabolic checkpoint, in HSC aging, which suggested that mitochondria metabolism may play a role to govern juvenescence of HSCs (12). Although these evidence remain unclear, pharmacological and metabolic interventions in these pathways may be continuously exploited to restore function in aged HSCs. Epigenetic reprogramming as a part of intrinsic regulation is one of the main factors in aging of HSCs. Although epigenetic abnormality is easily detected, correlation between aged HSC and epigenetic aberrancy is unreadable due to the slow loss of normal stem cell potential. Epigenetic regulation by TET2, DNMT3, and EZH2 led to hematologic malignant transformation, implying the faithful role of epigenetic control in the aging of HSCs (18). Epigenetic fidelity in a normal stem cell niche is required to maintain normal HSCs, because aging of HSCs is driven by a strong contribution of aged niche (19). Aged BM has two- to ten-fold HSCs when compared to that of the young BM (11). Multiple myeloma (MM) is a clonal plasma cell malignancy from BM failure by epigenetic defects (20). Thus, an epigenetic modulating agent such as decitabine is used to enhance therapeutic efficacy in MM. Regardless of aberrant epigenetic defects in the niche as well as autonomous HSCs, gene mutations may ultimately alter the epigenetic memory of HSCs, and then clonally expand with mutant epigenetic memory leading to abnormal hematopoiesis. It shows that modulation of epigenetic agents synergistically enhances clinical drug responses and normalization of abnormal mutation from aging status. The aging of HSCs depends on cellular changes, such as epigenetic factors, telomere and genomic damage, and molecular damage, including DNA damage, and finally resulting in dysfunctional HSCs (15). Notably, the immune system is regulated via the coordination of adaptive immune cells by lymphoid lineage cells, including T cells and B cells, and of innate immune cells by myeloid cells. Aging leads to a reduction in lymphocytes, especially naïve T cells, and the accumulation and clonal expansion of memory T cells (13). Meanwhile, myeloid lineage cells in BM appear to increase or persist at the same level depending on age, leading to a proinflammatory environment (14, 21). There are two models of HSC aging; one is a clonal alteration model, in which all lymphoid cells can temporarily be converted into myeloid cells due to aging via a differentiation stage. The other is a population shift model, in which myeloid-biased stem cells exclusively expand in old BM but not young BM, thus lymphoid-biased stem cell populations are overwhelmingly decreased, resulting in an imbalance (17, 21). As previously mentioned, there is some debate regarding the properties of aged HSCs in humans, and analysis of HSC characteristics during aging remains inconclusive. Pang et al. (22) insisted that aged HSCs do not engraft with increased homing abilities or differentiate into blood lineage cells with decreased efficiency compared to young HSCs. In contrast, increased proliferative activity has been reported by Kuranda et al. (23) in aged HSCs compared to young HSCs. Because both studies used different HSC markers to investigate the characteristics of aged and young HSCs, there have been inconsistencies after transplantation. Thus, the identification and isolation of bona fide HSCs are required prior to investigating aging HSCs, which can be achieved by applying recently developed advanced isolation techniques that ensure high purity, and using improved HSC-specific markers.
HSCT involves the intravenous infusion of autologous or allogeneic stem cells collected from BM, PB, and USB to reconstruct a functional hematopoietic system in patients with blood disorders. This process is used to allow a person to receive high-dose chemotherapy to treat a disease. Globally, 53% and 47% of HSCT is autologous and allogeneic, respectively, per year, and almost 68.8% of total 854 patients (Acute myeloid leukemia (AML) 158, acute lymphoblastic leukemia (ALL) 59, non-Hodgkin lymphoma (NHL) 392, Hodgkin disease (HD) 245) are alive 10 years after autologous HSCT (24). Cell therapy using HSCs was first performed more than 60 years ago (25). In humans, HSCs were identified and cultured in the 1980s (26). HSCT is one representative of many revolutionary solutions using HSCs, including cell and gene therapy. Among many other diseases, leukemia especially requires HSC therapy. AML is an aggressive malignancy with high mortality; it has been considered the worst blood cancer. For all AML types except M3, which is known as acute promyelocytic leukemia, HSCT is commonly conducted post-chemotherapy; the ultimate goal is the restoration of functional immune cells. HSCT prolongs life and can be divided into two main methods: autologous and allogeneic transplantation. Both methods of HSCT are regarded as supportive care and are an established therapy in many cases; in fact, HSCT has been integrated into the therapeutic strategy of most large, multicenter, cooperative study group trials. Recently, studies on the effectiveness of autologous HSCT in older adults have included patients harboring multiple types of hematologic malignancies; for example, autologous HSCT has been used as a therapeutic option among patients with both multiple myeloma (MM) and lymphoma (27). Among hematologic malignancies, MM is the most common indication for autologous HSCT, and overall survival is prolonged among patients treated with autologous HSCT compared to those treated with only conventional chemotherapy. Of note, autologous HSCT during the first complete remission is not superior to the continuation of chemotherapy in any of the reviewed trials. Although the debate is ongoing for AML, autologous transplantation in AML patients is still challenging due to the severe heterogeneity of AML and graft versus host disease (GVHD). Meanwhile, allogeneic HSCT is more popular for many types of diseases, including malignant and non-malignant disorders. For allogeneic HSCT, it is important to achieve a high degree of human leukocyte antigen (HLA) matching between the donor and recipient. Graft immune rejection and GVHD are remarkably decreased when well-matched HSCs are transplanted. To achieve this, the HSC donor should be investigated in diverse clinical aspects, especially hematologic condition and HLA typing. Allogenic HSCT is used to treat the following disorders: leukemia, including AML, ALL, chronic myeloid leukemia (CML), and chronic lymphocytic leukemia (CLL); myeloproliferative disorders; MM, NHL; and anemia. Young CLL patients are able to undergo both autologous and allogeneic HSCT. Both NHL and HD patients, who failed to achieve complete remission by conventional therapy, are used to high dose chemotherapy with HSCT. This treatment can successfully induce complete remission in relapsed patients (28). Anemia is a group of diseases involving red blood cells. Among them, thalassemia major patients need blood transfusions; however, blood transfusion is an insufficient treatment due to the presence of the genetic problems associated with the disease (29). HSCT treatment has undergone advancements over the last two decades, and 80% disease-free survival has been reported with allogeneic HSCT (30). Representative therapeutic approaches, such as HSCT, have been improved; furthermore, improved techniques, such as gene transfer with HSCT, could be used to treat a variety of diseases (31).
HSCT is feasible in myeloablated and non-myeloablated hosts, leading to a chimeric condition when allogeneic HSCT is carried out. In addition to using HSCT to induce hematopoietic cell reconstruction, cell therapy using BM-derived stem/progenitor cells has been continuously applied in regenerative medicine. Additionally, tissue-specific stem/progenitor cells in hematopoietic and non-hematopoietic tissues are also regarded as important therapeutic cell sources. BM-derived stem/progenitor cells are able to differentiate into cells of other lineages in a process called transdifferentiation, as well as differentiation (32). Many reports have shown that HSCs can transdifferentiate into cells of other lineages, such as endothelial cells, cardiomyocytes, neural cells, and hepatocytes (33, 34). These results seem to contradict the common assumptions of cell origins from the three germ layers and question whether HSCs are multipotent cells that can transdifferentiate or whether they are more primitive functional cells. However, since the mid-2000s, refutations of the plasticity of HSCs are emerging in regenerative medicine fields, suggesting that there is only a limited population of HSCs under physiological conditions (35). Stem/progenitor cells are needed to regenerate organs, and interest in stem/progenitor cells has been fueled by a serious need for lineage-specific progenitor cells for the treatment of diseases. Additionally, despite the rarity of stem/progenitor cells in regenerated organs, it is now believed that diverse effects from stem/progenitor cells other than direct cell contribution, such as paracrine effects, cell recruitment, and microenvironmental modulation, can repair injured organs. In particular, liver cells, which are from an endodermal lineage, rapidly acquire noticeable cellular functions and regenerative capacity. Because all changes in the liver are elicited by highly conserved intrinsic signals and genetic factors, the activity of both HSCs and oval progenitor cells in the liver is extremely relevant to developmental biology. Studies using animal disease models are leading to the development of methods to derive hepatocyte and hepatic oval cells from diverse stem/progenitor cell types (36). Among progenitor cells, endothelial progenitor cells (EPCs) represent progenitor cells that are derived from blood lineage cells; EPCs were first reported by Asahara et al. (32). Later, EPCs were studied by many investigators in regenerative medicine (37). Similar to HSCs, the ability to transdifferentiate EPCs into cells of other lineages has also been continuously debated (32). To enhance the use of stem/progenitor cells in therapeutic approaches, understanding how intrinsic regenerative capacities can be stimulated will help facilitate the control of cellular plasticity in stem/progenitor cells. Although the direct transdifferentiation of HSCs or BM-derived progenitor cells to regenerative organs has been continuously debated and does not seem to happen in vivo, HSCs/progenitor cells are still considered important elements in injury repair in terms of disease severity and organ types (38-49). We have briefly summarized the contribution by the plasticity of HSCs/progenitors to non-hematopoietic tissue regeneration in Table 1.
Liver regeneration is a fundamental response of the liver to injury. Similar to other organs, it is well known that local preexisting hepatic progenitor cells and circulating BM-derived progenitor cells are major contributors during liver regeneration (50). All elements are orchestrated, and then complete liver regeneration is accomplished. It has been shown that hepatocyte proliferation mainly contributes to liver regeneration and is regulated by various factors and cytokines, such as hepatocyte growth factor, epidermal growth factor, and interleukin-6 (51). When hepatocytes cannot function due to factors such as toxins and carcinogens, hepatic progenitor cells can be found in the injured liver. Liver progenitors/oval cells are very small and contain little cytoplasm and oval nuclei. Although preexisting mature hepatocytes are the quickest and most efficient cell sources for liver repair, their renewal capacity is exhausted and impaired under some pathological conditions. Thus, hepatic stem/progenitor cells, rather than mature hepatocytes, actively proliferate and differentiate into hepatocytes. This phenomenon occurs in both mice and humans (52). Clinically, liver transplantation is the only available therapy for terminal liver failure. However, the greatest impediment is finding a suitable HLA-matched donor. Therefore, there has been a major effort to develop alternative methods or sources to improve liver regeneration. Increasing evidence suggests that BM-derived stem/progenitor cells can differentiate into hepatocytes, thus resulting in the accumulation of hepatocytes (53). Starzl (54) performed the first liver transplantation and attempted five more transplantations in 1963. After that, the mixed chimeric status of blood cells in the liver after transplantation was reported by Taniguchi et al. in 1996 (53). Hepatocytes can be found in the transplanted livers of animals and humans, and transplantation of reduced-size liver grafts accelerates the recruitment and regeneration of these cells (50). This paper showed that BM-derived CD34+ cells can be directly incorporated into newly regenerated liver following liver transplantation in humans, suggesting the transdifferentiation of BM-derived stem/progenitor cells into hepatocytes. Although liver cells are derived from the endoderm and blood cells are derived from the mesoderm, the concept of transdifferentiation is emerging in liver regeneration fields. In mice, the adult liver consists of c-kit+Sca-1+Linlow/− cells, which are defined as HSCs, and these cells can form hematopoietic colonies in vivo and in vitro (53), which shows the intimate relationship between hematopoietic cells and hepatocytes. Although hepatic lineage cells are totally different from hematopoietic lineage cells, Petersen et al. also showed that hepatic oval cells can express the Thy-1 HSC marker when hepatic injury is induced by 2-acetylaminofluorene. This finding indicates that the Thy-1+ oval cell population could be used to study hepatic oval cells. Theise et al. (55) reported that BM cells can transdifferentiate into hepatocytes in irradiated mice and humans. Based on this concept, Lagasse et al. (34) tried to isolate purified HSCs and differentiate them into hepatocytes, resulting in a high proportion of bone marrow stem cell (BMSC)-derived hepatocytes following strong positive HSC selection. Several studies have suggested that hepatic stem/progenitor cells possess the phenotype of a side population of blood cells that has the ability to efflux Hoechst 33342. This side population of cells among liver cells is similar to hematopoietic lineage cells and expresses the CD34 marker. When hepatic stem cells are activated, BCRP1 (ABCG2) mRNA usually increases in liver tissue. Because BCRP1 is one of the determinants of the side population, its detection indicates an increase in hepatic stem cells with the side population phenotype (56). Most experiments that address the contribution of HSC-derived cells in liver regeneration use a mouse injury model and have shown that liver regeneration is caused by the clonal expansion of multipotent HSCs (33, 34, 50). Krause et al. (33) reported that non-hepatic adult stem cells can differentiate into hepatocytes in humans. In addition, the differentiation capacity of human HSCs into cells of other lineages, including skin, gut, and liver cells, has been reported (57). Human biopsy liver samples have shown that circulating blood stem cells differentiate into mature hepatocytes and epithelial cells via sex-mismatched transplantation. As mentioned previously, all extrahepatic stem/progenitor cells contribute to liver regeneration. However, the low efficiency (less than 0.01%) of extrahepatic stem/progenitor cell contribution post HSCT has been a matter of debate. Most scientists think that extrahepatic cells, such as BM-derived HSCs/progenitor cells, fuse with host hepatocytes rather than transdifferentiate, which requires confirmation by cytogenetic analysis (58). However, while it is relatively easy to obtain samples from mouse models, acquiring a transplanted liver sample from humans is very difficult. Nevertheless, all evidence suggests that cell fusion occurs rather than BM-derived HSCs/progenitor cell transdifferentiation. However, Lee et al. (50) clearly showed transdifferentiation of BM-derived CD34+ stem/progenitor cells in human liver transplants. Despite the different levels of contribution, BM-derived HSCs/progenitor cells might be a suitable tool for addressing some liver diseases, such as metabolic defects, via fusion-mediated additive gene transfer. Additionally, because de novo regenerated liver is very important for transplant survival in humans, the contribution of BM-derived HSCs is also considered important in clinical practice. Many clinical studies have suggested the implementation of HSC and hepatocyte transplantation (59). Clinical approaches using BM-derived cells, including mononuclear cells (MNCs), CD34+ stem cells, and mesenchymal stem/stromal cells (MSCs), have been attempted for the treatment of liver diseases (60-83). We expect that multipotent BM-stem/progenitor cells have beneficial effects and can rescue liver cells via cell transdifferentiation, paracrine effects, anti-fibrotic effects, and proangiogenic events. So far, there have been no unexpected severe side effects. However, the potentially increased risk of hepatic disease associated with HSC therapy requires long-term monitoring in clinical trials. In Table 2, we have summarized the reports on the use of HSC therapy.
Several assumptions regarding HSCs underlie the translation of stem cell biology to regenerative medicine. As mentioned, HSC/progenitor cells isolated using CD markers can potentially replace hematopoietic cells. Among all adult derived stem cells, HSCs are the most powerful cell sources to treat diverse diseases and regenerative medicine. Since HSCs can be differentiated into progenitors including endothelial progenitors and mesenchymal stem/progenitor cells, it may be useful to construct strategies for effective therapy in pathologic issues. Especially, the treatment of liver diseases using stem cell therapy has been improved, and there has been evidence of a positive effect from stem cells. Although the mechanism by which stem cells ameliorate diseases such as liver and blood disorders remain unclear, positive results are continuously reported for their use in regenerative medicine. These results strongly suggest that further research using stem cells will be required to fully elucidate potential adverse effects, such as immune rejection, and understanding HSCs will be helpful for further expansion with feasibility of using stem cells in regenerative medicine.
Acknowledgments
This study was supported by grants from the National Research Foundation (NRF) funded by the Korean government (MSIT) (2019R1A2C2005453), Basic Science Research Program through the NRF funded by the Ministry of Education (2017R1D1A1B03031406) and 2017 Research Grant from Kangwon National University (No. 520170448).
References
1. Till JE, Mcculloch EA. 1961; A direct measurement of the radiation sensitivity of normal mouse bone marrow cells. Radiat Res. 14:213–222. DOI: 10.2307/3570892. PMID: 13776896.


2. Cheng T, Rodrigues N, Shen H, Yang Y, Dombkowski D, Sykes M, Scadden DT. 2000; Hematopoietic stem cell quiescence maintained by p21cip1/waf1. Science. 287:1804–1808. DOI: 10.1126/science.287.5459.1804. PMID: 10710306.


3. Zhao M, Perry JM, Marshall H, Venkatraman A, Qian P, He XC, Ahamed J, Li L. 2014; Megakaryocytes maintain homeostatic quiescence and promote post-injury regeneration of hematopoietic stem cells. Nat Med. 20:1321–1326. DOI: 10.1038/nm.3706. PMID: 25326798.


4. Ye M, Zhang H, Amabile G, Yang H, Staber PB, Zhang P, Levantini E, Alberich-Jordà M, Zhang J, Kawasaki A, Tenen DG. 2013; C/EBPa controls acquisition and maintenance of adult haematopoietic stem cell quiescence. Nat Cell Biol. 15:385–394. DOI: 10.1038/ncb2698. PMID: 23502316. PMCID: PMC3781213.


5. Seita J, Weissman IL. 2010; Hematopoietic stem cell: self-renewal versus differentiation. Wiley Interdiscip Rev Syst Biol Med. 2:640–653. DOI: 10.1002/wsbm.86. PMID: 20890962. PMCID: PMC2950323.


6. Morrison SJ, Weissman IL. 1994; The long-term repopulating subset of hematopoietic stem cells is deterministic and isolatable by phenotype. Immunity. 1:661–673. DOI: 10.1016/1074-7613(94)90037-X. PMID: 7541305.


7. Mebius RE, Miyamoto T, Christensen J, Domen J, Cupedo T, Weissman IL, Akashi K. 2001; The fetal liver counterpart of adult common lymphoid progenitors gives rise to all lymphoid lineages, CD45+CD4+CD3-cells, as well as macrophages. J Immunol. 166:6593–6601. DOI: 10.4049/jimmunol.166.11.6593. PMID: 11359812.


8. Kim JY, Lee JY, Ha KS, Han ET, Park WS, Min CK, Hong SH. 2018; Perivascular cells and NADPH oxidase inhibition partially restore hyperglycemia-induced alterations in hematopoietic stem cell and myeloid-derived suppressor cell populations in the bone marrow. Int J Stem Cells. 12:63–72. DOI: 10.15283/ijsc18097. PMID: 30595009. PMCID: PMC6457702.


9. Thomas ED, Lochte HL Jr, Lu WC, Ferrebee JW. 1957; Intravenous infusion of bone marrow in patients receiving radiation and chemotherapy. N Engl J Med. 257:491–496. DOI: 10.1056/NEJM195709122571102. PMID: 13464965.


10. Thomas ED, Lochte HL Jr, Cannon JH, Sahler OD, Ferrebee JW. 1959; Supralethal whole body irradiation and isologous marrow transplantation in man. J Clin Invest. 38:1709–1716. DOI: 10.1172/JCI103949. PMID: 13837954. PMCID: PMC444138.


11. Morrison SJ, Wandycz AM, Akashi K, Globerson A, Weissman IL. 1996; The aging of hematopoietic stem cells. Nat Med. 2:1011–1016. DOI: 10.1038/nm0996-1011. PMID: 8782459.


12. Florian MC, Dörr K, Niebel A, Daria D, Schrezenmeier H, Rojewski M, Filippi MD, Hasenberg A, Gunzer M, Scharffetter-Kochanek K, Zheng Y, Geiger H. 2012; Cdc42 activity regulates hematopoietic stem cell aging and rejuvenation. Cell Stem Cell. 10:520–530. DOI: 10.1016/j.stem.2012.04.007. PMID: 22560076. PMCID: PMC3348626.


13. Dorshkind K, Montecino-Rodriguez E, Signer RA. 2009; The ageing immune system: is it ever too old to become young again? Nat Rev Immunol. 9:57–62. DOI: 10.1038/nri2471. PMID: 19104499.


14. Linton PJ, Dorshkind K. 2004; Age-related changes in lymphocyte development and function. Nat Immunol. 5:133–139. DOI: 10.1038/ni1033. PMID: 14749784.


15. Sun D, Luo M, Jeong M, Rodriguez B, Xia Z, Hannah R, Wang H, Le T, Faull KF, Chen R, Gu H, Bock C, Meissner A, Göttgens B, Darlington GJ, Li W, Goodell MA. 2014; Epigenomic profiling of young and aged HSCs reveals concerted changes during aging that reinforce self-renewal. Cell Stem Cell. 14:673–688. DOI: 10.1016/j.stem.2014.03.002. PMID: 24792119. PMCID: PMC4070311.


16. Allsopp RC, Morin GB, Horner JW, DePinho R, Harley CB, Weissman IL. 2003; Effect of TERT over-expression on the long-term transplantation capacity of hematopoietic stem cells. Nat Med. 9:369–371. DOI: 10.1038/nm0403-369. PMID: 12669037.


17. Mohrin M, Shin J, Liu Y, Brown K, Luo H, Xi Y, Haynes CM, Chen D. 2015; Stem cell aging. A mitochondrial UPR-mediated metabolic checkpoint regulates hematopoietic stem cell aging. Science. 347:1374–1377. DOI: 10.1126/science.aaa2361. PMID: 25792330. PMCID: PMC4447312.


18. Ley TJ, Miller C, Ding L, Raphael BJ, Mungall AJ, Robertson A, Hoadley K, Triche TJ Jr, Laird PW, Baty JD, Fulton LL, Fulton R, Heath SE, Kalicki-Veizer J, Kandoth C, Klco JM, Koboldt DC, Kanchi KL, Kulkarni S, Lamprecht TL, Larson DE, Lin L, Lu C, McLellan MD, McMichael JF, Payton J, Schmidt H, Spencer DH, Tomasson MH, Wallis JW, Wartman LD, Watson MA, Welch J, Wendl MC, Ally A, Balasundaram M, Birol I, Butterfield Y, Chiu R, Chu A, Chuah E, Chun HJ, Corbett R, Dhalla N, Guin R, He A, Hirst C, Hirst M, Holt RA, Jones S, Karsan A, Lee D, Li HI, Marra MA, Mayo M, Moore RA, Mungall K, Parker J, Pleasance E, Plettner P, Schein J, Stoll D, Swanson L, Tam A, Thiessen N, Varhol R, Wye N, Zhao Y, Gabriel S, Getz G, Sougnez C, Zou L, Leiserson MD, Vandin F, Wu HT, Applebaum F, Baylin SB, Akbani R, Broom BM, Chen K, Motter TC, Nguyen K, Weinstein JN, Zhang N, Ferguson ML, Adams C, Black A, Bowen J, Gastier-Foster J, Grossman T, Lichtenberg T, Wise L, Davidsen T, Demchok JA, Shaw KR, Sheth M, Sofia HJ, Yang L, Downing JR, Eley G. Cancer Genome Atlas Research Network. 2013; Genomic and epigenomic landscapes of adult de novo acute myeloid leukemia. N Engl J Med. 368:2059–2074. DOI: 10.1056/NEJMoa1301689. PMID: 23634996. PMCID: PMC3767041.
19. Sharma S, Gurudutta G. 2016; Epigenetic regulation of hematopoietic stem cells. Int J Stem Cells. 9:36–43. DOI: 10.15283/ijsc.2016.9.1.36. PMID: 27426084. PMCID: PMC4961102.


20. Dimopoulos K, Gimsing P, Grønbæk K. 2014; The role of epigenetics in the biology of multiple myeloma. Blood Cancer J. 4:e207. DOI: 10.1038/bcj.2014.29. PMID: 24786391. PMCID: PMC4042299.


21. Kim MJ, Kim MH, Kim SA, Chang JS. 2008; Age-related deterioration of hematopoietic stem cells. Int J Stem Cells. 1:55–63. DOI: 10.15283/ijsc.2008.1.1.55. PMID: 24855509. PMCID: PMC4021776.


22. Pang WW, Price EA, Sahoo D, Beerman I, Maloney WJ, Rossi DJ, Schrier SL, Weissman IL. 2011; Human bone marrow hematopoietic stem cells are increased in frequency and myeloid-biased with age. Proc Natl Acad Sci U S A. 108:20012–20017. DOI: 10.1073/pnas.1116110108. PMID: 22123971. PMCID: PMC3250139.


23. Kuranda K, Vargaftig J, de la Rochere P, Dosquet C, Charron D, Bardin F, Tonnelle C, Bonnet D, Goodhardt M. 2011; Age-related changes in human hematopoietic stem/progenitor cells. Aging Cell. 10:542–546. DOI: 10.1111/j.1474-9726.2011.00675.x. PMID: 21418508.


24. Bhatia S, Robison LL, Francisco L, Carter A, Liu Y, Grant M, Baker KS, Fung H, Gurney JG, McGlave PB, Nademanee A, Ramsay NK, Stein A, Weisdorf DJ, Forman SJ. 2005; Late mortality in survivors of autologous hematopoietic-cell transplantation: report from the Bone Marrow Transplant Survivor Study. Blood. 105:4215–4222. DOI: 10.1182/blood-2005-01-0035. PMID: 15701723. PMCID: PMC1895040.


25. Ford CE, Hamerton JL, Barnes DW, Loutit JF. 1956; Cytological identification of radiation-chimaeras. Nature. 177:452–454. DOI: 10.1038/177452a0. PMID: 13309336.
26. Morstyn G, Nicola NA, Metcalf D. 1980; Purification of hemopoietic progenitor cells from human marrow using a fucose-binding lectin and cell sorting. Blood. 56:798–805. DOI: 10.1182/blood.V56.5.798.798. PMID: 7000205.


27. Attal M, Harousseau JL, Stoppa AM, Sotto JJ, Fuzibet JG, Rossi JF, Casassus P, Maisonneuve H, Facon T, Ifrah N, Payen C, Bataille R. 1996; A prospective, randomized trial of autologous bone marrow transplantation and chemotherapy in multiple myeloma. Intergroupe Français du Myélome. N Engl J Med. 335:91–97. DOI: 10.1056/NEJM199607113350204. PMID: 8649495.


28. Kanate AS, Kharfan-Dabaja MA, Hamadani M. 2012; Controversies and recent advances in hematopoietic cell transplantation for follicular non-hodgkin lymphoma. Bone Marrow Res. 2012:897215. DOI: 10.1155/2012/897215. PMID: 23097707. PMCID: PMC3477524.


29. Boulad F, Wang X, Qu J, Taylor C, Ferro L, Karponi G, Bartido S, Giardina P, Heller G, Prockop SE, Maggio A, Sadelain M, Rivière I. 2014; Safe mobilization of CD34+ cells in adults with β-thalassemia and validation of effective globin gene transfer for clinical investigation. Blood. 123:1483–1486. DOI: 10.1182/blood-2013-06-507178. PMID: 24429337. PMCID: PMC3945860.


30. Lucarelli G, Isgrò A, Sodani P, Gaziev J. 2012; Hematopoietic stem cell transplantation in thalassemia and sickle cell anemia. Cold Spring Harb Perspect Med. 2:a011825. DOI: 10.1101/cshperspect.a011825. PMID: 22553502. PMCID: PMC3331690.


31. Biffi A, Montini E, Lorioli L, Cesani M, Fumagalli F, Plati T, Baldoli C, Martino S, Calabria A, Canale S, Benedicenti F, Vallanti G, Biasco L, Leo S, Kabbara N, Zanetti G, Rizzo WB, Mehta NA, Cicalese MP, Casiraghi M, Boelens JJ, Del Carro U, Dow DJ, Schmidt M, Assanelli A, Neduva V, Di Serio C, Stupka E, Gardner J, von Kalle C, Bordignon C, Ciceri F, Rovelli A, Roncarolo MG, Aiuti A, Sessa M, Naldini L. 2013; Lentiviral hematopoietic stem cell gene therapy benefits metachromatic leukodystrophy. Science. 341:1233158. DOI: 10.1126/science.1233158. PMID: 23845948.


32. Asahara T, Murohara T, Sullivan A, Silver M, van der Zee R, Li T, Witzenbichler B, Schatteman G, Isner JM. 1997; Isolation of putative progenitor endothelial cells for angiogenesis. Science. 275:964–967. DOI: 10.1126/science.275.5302.964. PMID: 9020076.


33. Krause DS, Theise ND, Collector MI, Henegariu O, Hwang S, Gardner R, Neutzel S, Sharkis SJ. 2001; Multi-organ, multi-lineage engraftment by a single bone marrow-derived stem cell. Cell. 105:369–377. DOI: 10.1016/S0092-8674(01)00328-2. PMID: 11348593.


34. Lagasse E, Connors H, Al-Dhalimy M, Reitsma M, Dohse M, Osborne L, Wang X, Finegold M, Weissman IL, Grompe M. 2000; Purified hematopoietic stem cells can differentiate into hepatocytes in vivo. Nat Med. 6:1229–1234. DOI: 10.1038/81326. PMID: 11062533.


35. Wagers AJ, Sherwood RI, Christensen JL, Weissman IL. 2002; Little evidence for developmental plasticity of adult hematopoietic stem cells. Science. 297:2256–2259. DOI: 10.1126/science.1074807. PMID: 12215650.


36. Zaret KS, Grompe M. 2008; Generation and regeneration of cells of the liver and pancreas. Science. 322:1490–1494. DOI: 10.1126/science.1161431. PMID: 19056973. PMCID: PMC2641009.


37. Takahashi T, Kalka C, Masuda H, Chen D, Silver M, Kearney M, Magner M, Isner JM, Asahara T. 1999; Ischemia- and cytokine-induced mobilization of bone marrow-derived endothelial progenitor cells for neovascularization. Nat Med. 5:434–438. DOI: 10.1038/7434. PMID: 10202935.


38. Eglitis MA, Mezey E. 1997; Hematopoietic cells differentiate into both microglia and macroglia in the brains of adult mice. Proc Natl Acad Sci U S A. 94:4080–4085. DOI: 10.1073/pnas.94.8.4080. PMID: 9108108. PMCID: PMC20571.


39. Ferrari G, Cusella-De Angelis G, Coletta M, Paolucci E, Stornaiuolo A, Cossu G, Mavilio F. 1998; Muscle regeneration by bone marrow-derived myogenic progenitors. Science. 279:1528–1530. DOI: 10.1126/science.279.5356.1528. PMID: 9488650.


40. Asahara T, Masuda H, Takahashi T, Kalka C, Pastore C, Silver M, Kearne M, Magner M, Isner JM. 1999; Bone marrow origin of endothelial progenitor cells responsible for postnatal vasculogenesis in physiological and pathological neovascularization. Circ Res. 85:221–228. DOI: 10.1161/01.RES.85.3.221. PMID: 10436164.


41. Petersen BE, Bowen WC, Patrene KD, Mars WM, Sullivan AK, Murase N, Boggs SS, Greenberger JS, Goff JP. 1999; Bone marrow as a potential source of hepatic oval cells. Science. 284:1168–1170. DOI: 10.1126/science.284.5417.1168. PMID: 10325227.


42. Mezey E, Chandross KJ, Harta G, Maki RA, McKercher SR. 2000; Turning blood into brain: cells bearing neuronal antigens generated in vivo from bone marrow. Science. 290:1779–1782. DOI: 10.1126/science.290.5497.1779. PMID: 11099419.


43. Alison MR, Poulsom R, Jeffery R, Dhillon AP, Quaglia A, Jacob J, Novelli M, Prentice G, Williamson J, Wright NA. 2000; Hepatocytes from non-hepatic adult stem cells. Nature. 406:257. DOI: 10.1038/35018642. PMID: 10917519.


44. Orlic D, Kajstura J, Chimenti S, Bodine DM, Leri A, Anversa P. 2001; Transplanted adult bone marrow cells repair myocardial infarcts in mice. Ann N Y Acad Sci. 938:221–229. discussion 229-230. DOI: 10.1111/j.1749-6632.2001.tb03592.x. PMID: 11458511.


45. Jackson KA, Majka SM, Wang H, Pocius J, Hartley CJ, Majesky MW, Entman ML, Michael LH, Hirschi KK, Goodell MA. 2001; Regeneration of ischemic cardiac muscle and vascular endothelium by adult stem cells. J Clin Invest. 107:1395–1402. DOI: 10.1172/JCI12150. PMID: 11390421. PMCID: PMC209322.


46. Körbling M, Katz RL, Khanna A, Ruifrok AC, Rondon G, Albitar M, Champlin RE, Estrov Z. 2002; Hepatocytes and epithelial cells of donor origin in recipients of peripheral-blood stem cells. N Engl J Med. 346:738–746. DOI: 10.1056/NEJMoa3461002. PMID: 11882729.


47. Yoon YS, Wecker A, Heyd L, Park JS, Tkebuchava T, Kusano K, Hanley A, Scadova H, Qin G, Cha DH, Johnson KL, Aikawa R, Asahara T, Losordo DW. 2005; Clonally expanded novel multipotent stem cells from human bone marrow regenerate myocardium after myocardial infarction. J Clin Invest. 115:326–338. DOI: 10.1172/JCI200522326. PMID: 15690083. PMCID: PMC546424.


48. Lee JY, Park C, Cho YP, Lee E, Kim H, Kim P, Yun SH, Yoon YS. 2010; Podoplanin-expressing cells derived from bone marrow play a crucial role in postnatal lymphatic neovascularization. Circulation. 122:1413–1425. DOI: 10.1161/CIRCULATIONAHA.110.941468. PMID: 20855662. PMCID: PMC2989430.


49. Duran JM, Makarewich CA, Sharp TE, Starosta T, Zhu F, Hoffman NE, Chiba Y, Madesh M, Berretta RM, Kubo H, Houser SR. 2013; Bone-derived stem cells repair the heart after myocardial infarction through transdifferentiation and paracrine signaling mechanisms. Circ Res. 113:539–552. DOI: 10.1161/CIRCRESAHA.113.301202. PMID: 23801066. PMCID: PMC3822430.


50. Lee SG, Moon SH, Kim HJ, Lee JY, Park SJ, Chung HM, Ha TY, Song GW, Jung DH, Park H, Kwon TW, Cho YP. 2015; Bone marrow-derived progenitor cells in de novo liver regeneration in liver transplant. Liver Transpl. 21:1186–1194. DOI: 10.1002/lt.24099. PMID: 25761987.


51. Michalopoulos GK, DeFrances MC. 1997; Liver regeneration. Science. 276:60–66. DOI: 10.1126/science.276.5309.60. PMID: 9082986.


52. Kakinuma S, Nakauchi H, Watanabe M. 2009; Hepatic stem/progenitor cells and stem-cell transplantation for the treatment of liver disease. J Gastroenterol. 44:167–172. DOI: 10.1007/s00535-008-2297-z. PMID: 19214659.


53. Taniguchi H, Toyoshima T, Fukao K, Nakauchi H. 1996; Presence of hematopoietic stem cells in the adult liver. Nat Med. 2:198–203. DOI: 10.1038/nm0296-198. PMID: 8574965.


54. Starzl TE. 2002; The saga of liver replacement, with particular reference to the reciprocal influence of liver and kidney transplantation (1955-1967). J Am Coll Surg. 195:587–610. DOI: 10.1016/S1072-7515(02)01498-9. PMID: 12437245. PMCID: PMC2993503.


55. Theise ND, Badve S, Saxena R, Henegariu O, Sell S, Crawford JM, Krause DS. 2000; Derivation of hepatocytes from bone marrow cells in mice after radiation-induced myeloablation. Hepatology. 31:235–240. DOI: 10.1002/hep.510310135. PMID: 10613752.


56. Shimano K, Satake M, Okaya A, Kitanaka J, Kitanaka N, Takemura M, Sakagami M, Terada N, Tsujimura T. 2003; Hepatic oval cells have the side population phenotype defined by expression of ATP-binding cassette transporter ABCG2/BCRP1. Am J Pathol. 163:3–9. DOI: 10.1016/S0002-9440(10)63624-3. PMID: 12819005. PMCID: PMC1868160.


57. Abkowitz JL. 2002; Can human hematopoietic stem cells become skin, gut, or liver cells? N Engl J Med. 346:770–772. DOI: 10.1056/NEJM200203073461012. PMID: 11882735.


58. Wang X, Willenbring H, Akkari Y, Torimaru Y, Foster M, Al-Dhalimy M, Lagasse E, Finegold M, Olson S, Grompe M. 2003; Cell fusion is the principal source of bone-marrow-derived hepatocytes. Nature. 422:897–901. DOI: 10.1038/nature01531. PMID: 12665832.


59. Terai S, Ishikawa T, Omori K, Aoyama K, Marumoto Y, Urata Y, Yokoyama Y, Uchida K, Yamasaki T, Fujii Y, Okita K, Sakaida I. 2006; Improved liver function in patients with liver cirrhosis after autologous bone marrow cell infusion therapy. Stem Cells. 24:2292–2298. DOI: 10.1634/stemcells.2005-0542. PMID: 16778155.


60. Yannaki E, Anagnostopoulos A, Kapetanos D, Xagorari A, Iordanidis F, Batsis I, Kaloyannidis P, Athanasiou E, Dourvas G, Kitis G, Fassas A. 2006; Lasting amelioration in the clinical course of decompensated alcoholic cirrhosis with boost infusions of mobilized peripheral blood stem cells. Exp Hematol. 34:1583–1587. DOI: 10.1016/j.exphem.2006.06.012. PMID: 17046578.


61. Gaia S, Smedile A, Omedè P, Olivero A, Sanavio F, Balzola F, Ottobrelli A, Abate ML, Marzano A, Rizzetto M, Tarella C. 2006; Feasibility and safety of G-CSF administration to induce bone marrow-derived cells mobilization in patients with end stage liver disease. J Hepatol. 45:13–19. DOI: 10.1016/j.jhep.2006.02.018. PMID: 16635534.


62. Gordon MY, Levicar N, Pai M, Bachellier P, Dimarakis I, Al-Allaf F, M'Hamdi H, Thalji T, Welsh JP, Marley SB, Davies J, Dazzi F, Marelli-Berg F, Tait P, Playford R, Jiao L, Jensen S, Nicholls JP, Ayav A, Nohandani M, Farzaneh F, Gaken J, Dodge R, Alison M, Apperley JF, Lechler R, Habib NA. 2006; Characterization and clinical application of human CD34+ stem/progenitor cell populations mobilized into the blood by granulocyte colony-stimulating factor. Stem Cells. 24:1822–1830. DOI: 10.1634/stemcells.2005-0629. PMID: 16556705.


63. Mohamadnejad M, Namiri M, Bagheri M, Hashemi SM, Ghanaati H, Zare Mehrjardi N, Kazemi Ashtiani S, Malekzadeh R, Baharvand H. 2007; Phase 1 human trial of autologous bone marrow-hematopoietic stem cell transplantation in patients with decompensated cirrhosis. World J Gastroenterol. 13:3359–3363. DOI: 10.3748/wjg.v13.i24.3359. PMID: 17659676. PMCID: PMC4172717.


64. Lyra AC, Soares MB, da Silva LF, Fortes MF, Silva AG, Mota AC, Oliveira SA, Braga EL, de Carvalho WA, Genser B, dos Santos RR, Lyra LG. 2007; Feasibility and safety of autologous bone marrow mononuclear cell transplantation in patients with advanced chronic liver disease. World J Gastroenterol. 13:1067–1073. DOI: 10.3748/wjg.v13.i7.1067. PMID: 17373741. PMCID: PMC4146869.


65. Yan L, Han Y, Wang J, Liu J, Hong L, Fan D. 2007; Peripheral blood monocytes from patients with HBV related decompensated liver cirrhosis can differentiate into functional hepatocytes. Am J Hematol. 82:949–954. DOI: 10.1002/ajh.21030. PMID: 17724706.


66. Khan AA, Parveen N, Mahaboob VS, Rajendraprasad A, Ravindraprakash HR, Venkateswarlu J, Rao SG, Narusu ML, Khaja MN, Pramila R, Habeeb A, Habibullah CM. 2008; Safety and efficacy of autologous bone marrow stem cell transplantation through hepatic artery for the treatment of chronic liver failure: a preliminary study. Transplant Proc. 40:1140–1144. DOI: 10.1016/j.transproceed.2008.03.111. PMID: 18555134.


67. Levicar N, Pai M, Habib NA, Tait P, Jiao LR, Marley SB, Davis J, Dazzi F, Smadja C, Jensen SL, Nicholls JP, Apperley JF, Gordon MY. 2008; Long-term clinical results of autologous infusion of mobilized adult bone marrow derived CD34+ cells in patients with chronic liver disease. Cell Prolif. 41(Suppl 1):115–125. DOI: 10.1111/j.1365-2184.2008.00491.x. PMID: 18181952. PMCID: PMC6496663.


68. Han Y, Yan L, Han G, Zhou X, Hong L, Yin Z, Zhang X, Wang S, Wang J, Sun A, Liu Z, Xie H, Wu K, Ding J, Fan D. 2008; Controlled trials in hepatitis B virus-related decompensate liver cirrhosis: peripheral blood monocyte transplant versus granulocyte-colony-stimulating factor mobilization therapy. Cytotherapy. 10:390–396. DOI: 10.1080/14653240802129901. PMID: 18574771.


69. Pai M, Zacharoulis D, Milicevic MN, Helmy S, Jiao LR, Levicar N, Tait P, Scott M, Marley SB, Jestice K, Glibetic M, Bansi D, Khan SA, Kyriakou D, Rountas C, Thillainayagam A, Nicholls JP, Jensen S, Apperley JF, Gordon MY, Habib NA. 2008; Autologous infusion of expanded mobilized adult bone marrow-derived CD34+ cells into patients with alcoholic liver cirrhosis. Am J Gastroenterol. 103:1952–1958. DOI: 10.1111/j.1572-0241.2008.01993.x. PMID: 18637092.


70. Salama H, Zekri AR, Bahnassy AA, Medhat E, Halim HA, Ahmed OS, Mohamed G, Al Alim SA, Sherif GM. 2010; Autologous CD34+ and CD133+ stem cells transplantation in patients with end stage liver disease. World J Gastroenterol. 16:5297–5305. DOI: 10.3748/wjg.v16.i42.5297. PMID: 21072892. PMCID: PMC2980678.


71. Kim JK, Park YN, Kim JS, Park MS, Paik YH, Seok JY, Chung YE, Kim HO, Kim KS, Ahn SH, Kim DY, Kim MJ, Lee KS, Chon CY, Kim SJ, Terai S, Sakaida I, Han KH. 2010; Autologous bone marrow infusion activates the progenitor cell compartment in patients with advanced liver cirrhosis. Cell Transplant. 19:1237–1246. DOI: 10.3727/096368910X506863. PMID: 20525430.


72. Lyra AC, Soares MB, da Silva LF, Braga EL, Oliveira SA, Fortes MF, Silva AG, Brustolim D, Genser B, Dos Santos RR, Lyra LG. 2010; Infusion of autologous bone marrow mononuclear cells through hepatic artery results in a short-term improvement of liver function in patients with chronic liver disease: a pilot randomized controlled study. Eur J Gastroenterol Hepatol. 22:33–42. DOI: 10.1097/MEG.0b013e32832eb69a. PMID: 19654548.


73. Khan AA, Shaik MV, Parveen N, Rajendraprasad A, Aleem MA, Habeeb MA, Srinivas G, Raj TA, Tiwari SK, Kumaresan K, Venkateswarlu J, Pande G, Habibullah CM. 2010; Human fetal liver-derived stem cell transplantation as supportive modality in the management of end-stage decompensated liver cirrhosis. Cell Transplant. 19:409–418. DOI: 10.3727/096368909X484707a. PMID: 20447340.


74. Nikeghbalian S, Pournasr B, Aghdami N, Rasekhi A, Geramizadeh B, Hosseini Asl SM, Ramzi M, Kakaei F, Namiri M, Malekzadeh R, Vosough Dizaj A, Malek-Hosseini SA, Baharvand H. 2011; Autologous transplantation of bone marrow-derived mononuclear and CD133(+) cells in patients with decompensated cirrhosis. Arch Iran Med. 14:12–17. PMID: 21194255.
75. Saito T, Okumoto K, Haga H, Nishise Y, Ishii R, Sato C, Watanabe H, Okada A, Ikeda M, Togashi H, Ishikawa T, Terai S, Sakaida I, Kawata S. 2011; Potential therapeutic application of intravenous autologous bone marrow infusion in patients with alcoholic liver cirrhosis. Stem Cells Dev. 20:1503–1510. DOI: 10.1089/scd.2011.0074. PMID: 21417817.


76. Amer ME, El-Sayed SZ, El-Kheir WA, Gabr H, Gomaa AA, El-Noomani N, Hegazy M. 2011; Clinical and laboratory evaluation of patients with end-stage liver cell failure injected with bone marrow-derived hepatocyte-like cells. Eur J Gastroenterol Hepatol. 23:936–941. DOI: 10.1097/MEG.0b013e3283488b00. PMID: 21900788.


77. Garg V, Garg H, Khan A, Trehanpati N, Kumar A, Sharma BC, Sakhuja P, Sarin SK. 2012; Granulocyte colony-stimulating factor mobilizes CD34(+) cells and improves survival of patients with acute-on-chronic liver failure. Gastroenterology. 142:505–512.e1. DOI: 10.1053/j.gastro.2011.11.027. PMID: 22119930.


78. Spahr L, Chalandon Y, Terraz S, Kindler V, Rubbia-Brandt L, Frossard JL, Breguet R, Lanthier N, Farina A, Passweg J, Becker CD, Hadengue A. 2013; Autologous bone marrow mononuclear cell transplantation in patients with decompensated alcoholic liver disease: a randomized controlled trial. PLoS One. 8:e53719. DOI: 10.1371/journal.pone.0053719. PMID: 23341981. PMCID: PMC3544843.


79. Andreone P, Catani L, Margini C, Brodosi L, Lorenzini S, Sollazzo D, Nicolini B, Giordano R, Montemurro T, Rizzi S, Dan E, Giudice V, Viganò M, Casadei A, Foschi FG, Malvi D, Bernardi M, Conti F, Lemoli RM. 2015; Reinfusion of highly purified CD133+ bone marrow-derived stem/progenitor cells in patients with end-stage liver disease: A phase I clinical trial. Dig Liver Dis. 47:1059–1066. DOI: 10.1016/j.dld.2015.08.018. PMID: 26427587.


80. King A, Barton D, Beard HA, Than N, Moore J, Corbett C, Thomas J, Guo K, Guha I, Hollyman D, Stocken D, Yap C, Fox R, Forbes SJ, Newsome PN. 2015; Repeated AutoLogous Infusions of STem cells In Cirrhosis (REALISTIC): a multicentre, phase II, open-label, randomised controlled trial of repeated autologous infusions of granulocyte colony-stimulating factor (GCSF) mobilised CD133+ bone marrow stem cells in patients with cirrhosis. A study protocol for a randomised controlled trial. BMJ Open. 5:e007700. DOI: 10.1136/bmjopen-2015-007700. PMID: 25795699. PMCID: PMC4368910.


81. Sharma M, Rao PN, Sasikala M, Kuncharam MR, Reddy C, Gokak V, Raju B, Singh JR, Nag P, Nageshwar Reddy D. 2015; Autologous mobilized peripheral blood CD34(+) cell infusion in non-viral decompensated liver cirrhosis. World J Gastroenterol. 21:7264–7271. DOI: 10.3748/wjg.v21.i23.7264. PMID: 26109814. PMCID: PMC4476889.


82. Mohamadnejad M, Vosough M, Moossavi S, Nikfam S, Mardpour S, Akhlaghpoor S, Ashrafi M, Azimian V, Jarughi N, Hosseini SE, Moeininia F, Bagheri M, Sharafkhah M, Aghdami N, Malekzadeh R, Baharvand H. 2016; Intraportal infusion of bone marrow mononuclear or CD133+ cells in patients with decompensated cirrhosis: a double-blind randomized controlled trial. Stem Cells Transl Med. 5:87–94. DOI: 10.5966/sctm.2015-0004. PMID: 26659833. PMCID: PMC4704869.


83. Yu SJ, Yoon JH, Kim W, Lee JM, Lee YB, Cho Y, Lee DH, Lee M, Yoo JJ, Cho EJ, Lee JH, Kim YJ, Kim CY. 2017; Ultrasound-guided percutaneous portal transplantation of peripheral blood monocytes in patients with liver cirrhosis. Korean J Intern Med. 32:261–268. DOI: 10.3904/kjim.2015.267. PMID: 27044856. PMCID: PMC5339463.


Fig. 1
Schematic illustration showed hematopoiesis in adult BM. HSCs have self-renewal activity and differentiation capability into blood cell lineages. LT-HSCs are quiescent and sustained by arrested cell division in endosteal niche, whereas ST-HSCs are promptly differentiated into myeloid and lymphoid lineage cells in vascular niche, and then lineage cells exit into periphery.
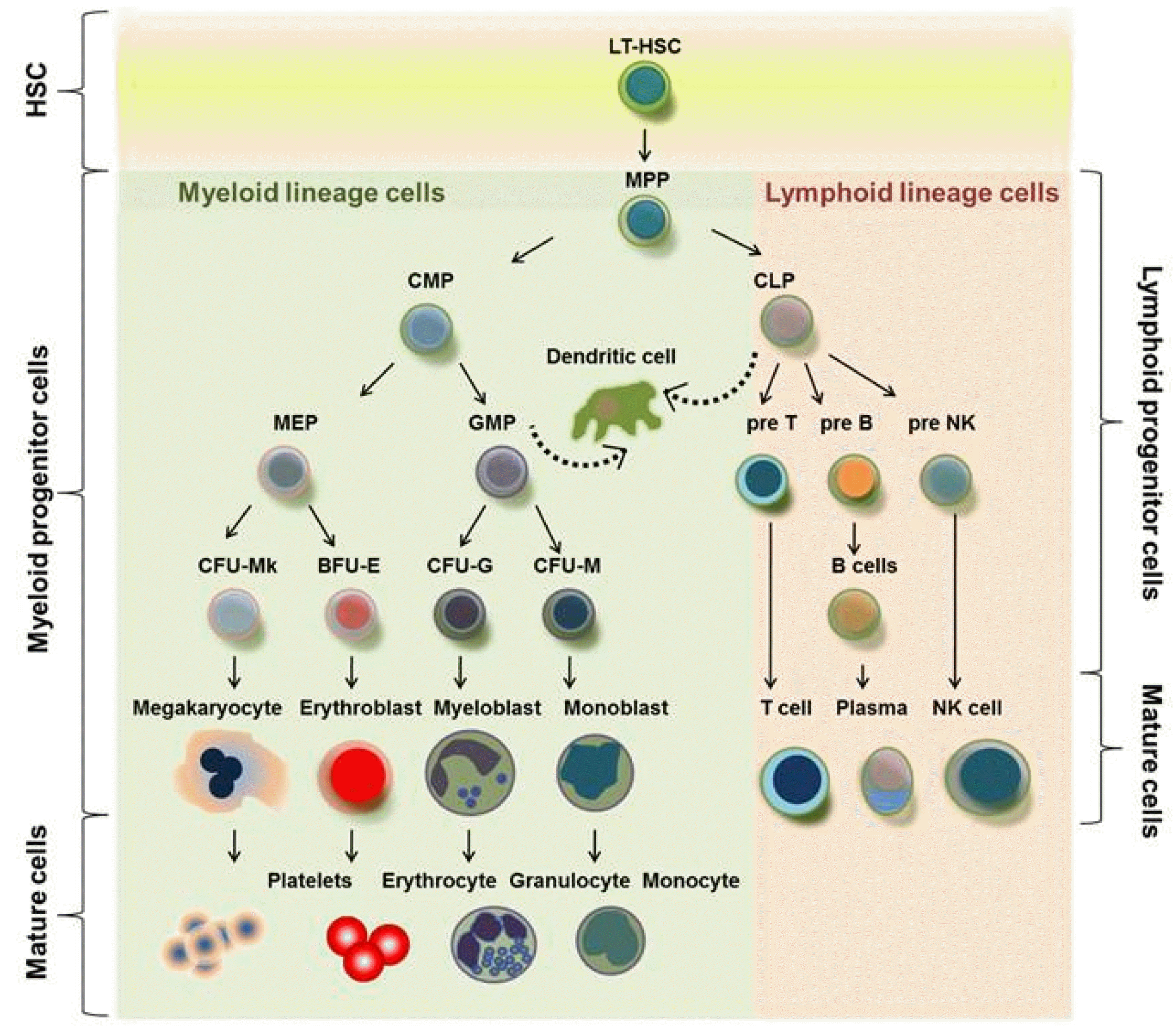
Table 1
Contribution of HSCs/progenitor cells in regeneration of non-hematopoietic cells
Source of stem cells | Differentiation of HSCs/progenitors | Year | Reference | |
---|---|---|---|---|
1 | BM-hematopoietic cells | Microglia/Macroglia | 1997 | (38) |
2 | BM-myogenic progenitors | Muscle cells | 1998 | (39) |
3 | BM-Stem cells | Endothelial precursors | 1999 | (40) |
4 | BM-Stem cells | Hepatic oval cells | 1999 | (41) |
5 | BM-Stem cells | Neuronal cells | 2000 | (42) |
6 | BM-Stem cells | Hepatocytes | 2000 | (43) |
7 | Hematopoietic stem cells | hepatocytes | 2000 | (34) |
8 | BM-Stem cells | Cardiac muscle | 2001 | (44) |
9 | BM-side population cells | Cardiac muscle, endothelium | 2001 | (45) |
10 | PB-stem cells | Hepatocytes/epithelial cells | 2002 | (46) |
11 | BM-Stem cells | Cardiac muscle | 2005 | (47) |
12 | BM-Stem cells | Lymphatic endothelial progenitors | 2010 | (48) |
13 | Corticalbone-derived stemcell | Myocytes/vascular cells | 2013 | (49) |
Table 2
Clinical trials on stem cell therapy in liver disease
Source of stem cells | No. of patients | Year | Reference | |
---|---|---|---|---|
1 | BM-MNCs | 9 | 2006 | (59) |
2 | PB-MNCs, G-CSF | 2 | 2006 | (60) |
3 | BM-MSCs, G-CSF | Active Treatment: 8 (5 male) | 2006 | (61) |
4 | CD34+ cells | 5 | 2006 | (62) |
5 | CD34+ cells | 4 | 2007 | (63) |
6 | BM-MSCs | 10 | 2007 | (64) |
7 | PB-monocytes, G-CSF | 2 | 2007 | (65) |
8 | CD34+ cells | 4 | 2008 | (66) |
9 | CD34+ cells | 5 | 2008 | (67) |
10 | PB-MSCs, G-CSF | 40 | 2008 | (68) |
11 | CD34+ cells | 9 | 2008 | (69) |
12 | CD34+, CD133+ HSCs | Active Treatment: 90 (78 male) | 2010 | (70) |
13 | BM-MNCs | 10 | 2010 | (71) |
14 | BM-MNCs | 15 | 2010 | (72) |
15 | hHPCs | Active Treatment: 4 | 2010 | (73) |
16 | BM-MNCs, HSCs | 6 | 2011 | (74) |
17 | BM-MNCs | 5 | 2011 | (75) |
18 | BM-MNCs (BM-derived hepatocytes) | 20 | 2011 | (76) |
19 | CD34+ cells, G-CSF | 23 | 2012 | (77) |
20 | BM-MSCs, G-CSF | 28 | 2013 | (78) |
21 | BM CD133+ cells | 16 | 2015 | (79) |
22 | BM CD134+ cells, G-CSF | 81 | 2015 | (80) |
23 | PB CD34+ cells | 22 | 2015 | (81) |
24 | BM CD133+ cells, MNCs | 12 | 2016 | (82) |
25 | PB monocytes | 9 | 2017 | (83) |
|
||||
Source of stem cells | Clinicaltrials.gov identifier | Year | Phase | |
|
||||
26 | Autologous expanded CD34+ HSCs | NCT00655707 | 2008∼2015 | Phase2 |
27 | Autologous BM cells | NCT02943707 | 2016∼2020 | Phase2 |
28 | Autologous BM-derived CD133 stem cells | NCT01120925 | 2010∼2014 | Phase2 |
29 | BM cells | NCT01412593 | 2011∼2013 | Phase2 |
30 | Autologous BM-derived CD133 stem cells | NCT00713934 | 2008∼2010 | Phase1 |
31 | Adult stem cells | NCT00147034 | 2005∼2016 | Phase1 |
32 | Autologous BM-derived CD133 stem cells | NCT01025622 | 2009∼2010 | Phase1 |
33 | Allogenic BMSCs | NCT01221454 | 2010 | Phase2 |
34 | Allogenic BMSCs | NCT01223664 | 2010 | Phase2 |
35 | Human BMSCs | NCT01724697 | 2012 | Phase1 |
36 | Autologous BMSCs | NCT02943707 | 2016 | Phase2 |