Abstract
Sleep surgery is part of a continuum of care for obstructive sleep apnea (OSA) that involves medical, pharmacologic, and behavioral therapy. Upper airway surgery for OSA can significantly improve stability by way of modulating the critical negative closing pressure. This is the same mechanism of action as positive airway pressure or oral appliance therapy. The updated surgical algorithm in this review adds precision in three areas: patient selection, identification of previously unaddressed anatomic phenotypes with associated treatment modality, and improved techniques of previously established procedures. While the original Riley and Powell phase 1 and 2 approach to sleep surgery has focused on individual surgical success rate, this algorithm strives for an overall treatment success with multi-modal and patient-centric treatments.
Sleep surgery has come a long way in the three decades since the advent of the original phase 1 and 2 Stanford algorithm by Riley and Powell [1]. Phase 1 involves multi-level surgery including tonsillectomy and uvulopalatal flap with genioglossus advancement (GA) [1,2]. Inadequate responders to phase 1 are recommended phase 2 surgery: maxillomandibular advancement (MMA) [3]. This protocol compared favorably to positive airway pressure (PAP) therapy, especially with the inclusion of MMA [4]. The drawback to the algorithm is its unidirectional nature. It assumes that obstructive sleep apnea (OSA) does not recur if a cure, defined as Apnea Hypopnea Index (AHI) less than five events per hour, is achieved at one point. It also does not take into account patient preferences of elective procedures. Finally, it does not incorporate multi-modal treatment such as PAP and oral appliance therapy (OAT).
Sleep surgery is part of a continuum of care for OSA that involves medical, pharmacologic, and behavioral therapy. Upper airway surgery for OSA may not alter arousal threshold, loop gain, or muscle tone. It can, however, significantly change the critical negative closing pressure [5]. This is the same mechanism of action as PAP or OAT. The updated surgical algorithm adds precision in three areas: (1) patient selection, (2) identification of previously unaddressed anatomic phenotypes, and (3) surgical techniques [6,7]. Conceptually, while the focus has been on individual surgical success rate, this algorithm strives for an overall treatment success. The growing understanding of the pathophysiology of OSA and development of new methods for airway evaluation allow improved phenotyping of the upper airway and customization of surgical treatment. This review provides an update the original surgical algorithm for OSA.
Precise phenotyping of patients with polysomnographic findings, targeted physical examination, and diagnostic tools are critical to optimizing surgical success in patients with OSA. Sleep surgeons should consider the patient’s preferences, expectations, associated comorbidities, OSA severity, and individual anatomy.
A meticulous sleep-specific history, full head and neck examination including the nasal airway, velopharynx, pharyngeal wall, tongue base, and epiglottis, and facial skeletal relationship should be performed to facilitate surgical planning [6]. Nasal obstruction may be the main cause of PAP intolerance [8,9]. Therefore, care should be taken to assess anatomical abnormalities causing nasal obstruction, including posterior septal deviation [10,11]. Exam should involve endoscopic examination to identify all possible anatomic and functional causes of nasal obstruction [10]. While there is only modest correlation between the severity of disease and grading of airway collapse during Muller’s maneuver, the negative pressure maneuver is a quick method to assess upper airway collapsibility [12]. A mandibular protrusion maneuver can be performed at the same time to visualize the degree of lateral pharyngeal wall dilation and tongue base advancement. Nasal examination is not limited to the nose alone. Long-term nasal obstruction leads to facial changes, most often in the appearance of a long midface, open bite, and retruded mandible. Intraoral examination tends to show narrow, high-arch maxilla with the appearance of a relatively large tongue and redundant soft palatal tissue (Fig. 1). This is the classic adenoid facies associated with chronic mouth breathing [13-15]. The internal nasal valve (INV), the most restrictive part for nasal airflow, is a target for intervention. The INV includes the septum, the inferior turbinates, the upper lateral cartilage, and the nasal floor.
Overnight polysomnography (PSG) is the gold standard for diagnosing and evaluating the severity of OSA, although it may not reflect a patient’s status over a long period of time [16]. The severity of OSA is measured by the AHI. Beyond the AHI, oxygen desaturation nadir also reflects the severity of OSA. Some studies have shown that the oxygen desaturation index correlates more strongly with cardiovascular morbidity of OSA than the AHI alone [17,18]. Recently, ambulatory sleep study is increasingly replacing level 1 PSG. It must be noted that ambulatory sleep testing tends to underestimate OSA severity [19-21]. Surgical success should be compared using the same diagnostic study and hypopnea criteria before and after surgery [6,22].
Various diagnostic tools including cephalometric X-ray [23], awake flexible laryngoscope [24], acoustic analysis [25], computed tomography (CT) scan [26], and dynamic magnetic resonance imaging [27] have been introduced to identify and phenotype the upper airway anatomy among OSA patients. However, most airway assessment techniques are performed in the awake and static state, which does not reflect sleep-disordered breathing [28]. Dynamic airway assessment has been augmented with examination under drug-induced sedation (sleep) endoscopy (DISE) [29,30]. DISE is a useful tool to visualize and phenotype this pharyngeal muscle buttress system that varies in length, lateral dimension, antero-posterior diameter, and structural elements, and is influenced by the skeletal structure within which it is located [28].
There is no consensus regarding standardized protocols for DISE. The procedure is usually performed in the supine position in an outpatient surgery setting with monitoring of oxygen saturation, heart rate, blood pressure, and sometimes, Bispectral index score. Propofol, dexmedetomidine, and midazolam are commonly used for induction of sedation. Propofol has the benefit of rapid onset of action and recovery with minimal side effects [31]. The genioglossus muscle tone is decreased by up to 10% of the maximum awake activity in healthy individuals during propofol unconscious sedation [32,33]. Currently, the effect of midazolam and dexmedetomidine on genioglossus muscle tone is unknown [34]. Midazolam has a greater therapeutic range but is limited by its slow onset and potential to cause respiratory depression. Dexmedetomidine has the characteristics of rapid onset and small therapeutic range, with less respiratory side effects [35]. The depth of sedation is critical and evaluated by the onset of disordered breathing or the Bispectral index score [32].
Several classification systems have been introduced to characterize DISE findings [36-40]. The VOTE classification system, comprised of the Velum, Oropharyngeal (lateral walls), Tongue, and Epiglottis, is widely used for DISE scoring. The most common finding from DISE is multilevel collapse, despite heterogeneity among studies [41,42]. The patterns of complete concentric collapse (CCC), multilevel collapse, and tongue base collapse are associated with higher AHI [41,42]. CCC has been associated with poor surgical outcomes in multilevel surgery and upper airway stimulation (UAS) [43,44], but is well-addressed by MMA [45].
Nasal breathing is an important factor for sleep quality, and nasal obstruction does contribute to the pathogenesis of OSA [46,47]. Septal deviation, turbinate hypertrophy, and valve dysfunction can result in increased nasal resistance and subsequent mouth breathing. Increased nasal resistance leads to downstream inspiratory collapse of the oropharynx or hypopharynx in susceptible OSA patients [48,49]. Mouth breathing can also cause posterior displacement of the base of the tongue and consequent narrowing of the hypopharyngeal airway [7]. Nasal surgery including septoplasty, turbinoplasty, or valve reconstruction can restore nasal airway patency and reduce nasal resistance and mouth breathing. Although nasal surgery alone shows limited efficacy in terms of AHI [50], it improves sleep quality, OSA-related sleep symptoms, and PAP compliance [51-53]. Nasal surgery is important in the multilevel treatment plan for OSA [54].
Expansion of the adult nasal floor is useful for OSA patients who present with narrow and high-arch maxilla [15]. Patients with this phenotype tend struggle with both nasal obstruction and lack of intraoral volume for the tongue during sleep. Maxillary expansion directed at the nasal floor by way of distraction osteogenesis with maxillary expansion (distraction osteogenesis maxillary expansion [DOME]) has shown promise [55-58]. Minimally invasive osteotomies can be made at the LeFort I level via an intranasal incision. An expander is anchored to the roof of maxilla intraorally. The patient turns the expander once a day, which translates to an expansion of 0.025 mm. This generally results in 8 to 10 mm of widened nasal floor at the INV in a month (Fig. 2). Orthodontic treatment restores the occlusion. Conceptually similar to pediatric rapid maxillary expansion, DOME effectively addresses the same anatomic phenotype in adults [58-62].
Uvulopalatopharyngoplasty (UPPP) remains the most commonly performed sleep surgical procedure worldwide [63]. Most surgeons specialized in OSA have stopped performing earlier methods of UPPP, which tend to be ablative in nature including resection of the uvula. This is particularly true in procedures such as the laser-assisted UPPP which worsens AHI in 44% of patients based on meta-analysis [64]. Isolated soft palate surgery has the highest success rate in Friedman stage I patients [38]. In clinical practice, various forms of UPPP are often performed as part of multi-level surgery to maximize surgical success [1,65,66]. In the Riley-Powell sleep surgery algorithm, uvulopalatal flap is part of multi-level surgery with GA during phase 1. The uvulopalatal flap was designed as a reversible soft palate procedure in the event of velopharyngeal insufficiency [2]. Most forms of contemporary UPPP focus on palatal muscle expansion and stabilization with targeted vectors during suturing [67-70].
Various techniques of palatopharyngoplasty such as lateral pharyngoplasty, expansion sphincter pharyngoplasty, and transpalatal advancement pharyngoplasty have been introduced to resolve the limitations of classic UPPP. Collectively, they have shown more successful outcomes than the classic UPPP [28,71-75]. Sleep surgeons can individualize the options according to the pattern of collapse. For example, lateral pharyngoplasty, expansion sphincter pharyngoplasty, or similar procedures can be applied to lateral pharyngeal collapse, while transpalatal advancement pharyngoplasty can be utilized in anteroposterior narrowing [76].
An indication for isolated UPPP is part of a phased approach towards UAS. CCC of the soft palate (velum) seen during DISE is an exclusion criteria for UAS, palatopharyngoplasty can reverse this collapse pattern and increase candidacy for UAS [77].
Untreated retrolingual obstruction is well recognized as a major cause of surgical failure [78]. Removal of the lingual tonsils and base of tongue fat may involve the use of coblation, laser, or robotic assistance per surgeon preference [79-81]. The removal of tissue in this area can be supplemented by an anterior anchorage of the epiglottis to the base of tongue for epiglottis collapse. With high quality optics for improved visualization and instrumentation, robotics was adapted and introduced to target the posterior tongue [81,82]. While transoral robotic surgery (TORS) offers unparalleled visualization, the use of multi-armed robots originally designed for the abdominal cavity can be cumbersome for the upper airway. Results for the use of TORS as part of a multilevel surgical approach for OSA are promising for select patients. Success rate of TORS was higher than 75% in non-obese patients and 50% in obese patients with OSA [83].
The cost and morbidity may be greater than with other techniques offsetting its advantages in visualization and precision [84]. On comparing the surgical outcomes between TORS (n=820) and coblation (n=262), the mean rate of failure was found to be 34.4% in TORS and 38.5% in the coblation group. The postoperative complication rates were 21.3% and 8.4% [85]. The advent of single port robot system which is designed for single cavity operative sites is promising [86]. Augmented reality assisted TORS using a single-port robot will reduce morbidity such as bleeding and increase precision in distinguishing fat from muscle [87].
Classic GA was designed by Powell and Riley as part of phase 1 algorithm. GA is usually performed in conjunction with other procedures (UPPP, MMA) [88]. The genioglossus muscle, a powerful dilator muscle of the upper airway, is attached to the genial tubercles. In advancing the genial tubercles, the genioglossus muscle strengthens over time and allows greater tongue advancement during sleep [89]. With the wide availability of CT scan, virtual surgical planning and osteotomy guides allow contemporary GA to be considerably more precise (Fig. 3) [90]. GA and genioplasty can often be performed in conjunction to improve facial balance in retrognathic patients [91].This combination also exerts strengthening effects on suprahyoid muscles.
At the time of publication, there is only one Food and Drug Administration-approved UAS device (Inspire Medical Systems, Maple Grove, MN, USA) for OSA. It generates a unilateral respiration-synchronized stimulation of the medial hypoglossal nerve branches and C1 nerve, leading to tongue stiffening and protrusion during sleep via the genioglossus and geniohyoid muscles (Fig. 4). The hypoglossal nerve (CN XII) innervates both the tongue protrusor (genioglossus) and retrusor (styloglossus and hyoglossus) muscles through its medial and lateral divisions. Selective stimulation of the protrusor muscles leads to anterior movement of the tongue, resulting in increased airflow and reduced pharyngeal collapse during sleep [92]. Selective stimulation of the deep and horizontally oriented genioglossus fibers results in curling and stiffening of the tongue, further expanding the upper airway [93].
The current selection criteria requires DISE to rule out CCC of the velum. There is a body mass index (BMI) requirement of 32 kg/m2 and below, and an AHI range from 15 to 65. There is a 25% cutoff for central apneas. Implanted patients undergo inlab titration of UAS approximately 2 months after implantation.
The Stimulation Therapy for Apnea Reduction (STAR) trial found UAS to be successful with a median decrease of 68% in AHI [94]. Recent meta-analyses show that UAS is a safe and effective for selected patients with moderate to severe OSA [95]. A study including 102 patients revealed that 22.6% of the patients used UAS therapy for less than 4 hours per night, 77.4% for 4 hours or more per night, and 55.7% of the patients for more than 6 hours per night [96]. UAS can improve AHI as well as sleep architecture in responders. Arousal index and N1 sleep were reduced while time spent in N2 and slow wave sleep increased after UAS. There are no significant changes to rapid eye movement (REM) sleep [97].
MMA is pioneered by Riley and Powell at Stanford Hospital in the late 1980’s, and addresses the entire upper airway that can contribute to OSA. It remains one of the most effective surgical intervention for patients with OSA, and has compared favorably to continuous positive airway pressure (CPAP) in a variety of studies including a prospective, randomized controlled trial [1,3,4,89,98-101]. MMA involves osteotomies of the maxilla and mandible, followed by their advancement that is frequently accompanied with counterclockwise rotation (Fig. 5) [102,103]. The net effect includes greater volume for intraoral soft tissue structures and stability of the upper airway dilator muscles [7,45,104,105]. Generally, indications for MMA are : (1) moderate to severe OSA with our without history of phase 1 surgery, (2) OSA of all severity if there is comorbid dentofacial deformity, and (3) concentric and lateral pharyngeal wall collapse seen with DISE [6,7,103]. Age of patient and severity of OSA have not been shown to impact the technical aspects of MMA in a high volume center [106].
Meta-analysis by Holty and Guilleminault [107] examined 22 studies involving 627 patients who underwent MMA, reporting mean AHI decrease from 63.9 to 9.5 events per hour. The authors defined surgical success with the Sher criteria: a minimum of 50% reduction with a final AHI less than 20. The surgical success rate was 86.0% and the cure rate (AHI <5) was 43.2%. The predictive factors for surgical success were younger age, lower BMI, and greater degree of maxillary advancement. The major and minor complication rates were 1.0% and 3.1%, respectively. Zaghi et al. [108] updated the meta-analsysis with 45 studies and 528 patients reporting success and cure rates of 85.5% and 38%, respectively. In 40 patients who underwent MMA with average follow-up of 4.2 years (range, 1 to 12 years), 36 patients (90%) maintained a significant reduction in respiratory disturbance index from 71.2 to 7.6 events per hour with improvement in daytime sleepiness [109]. In another study with mean follow-up of 12.5 years, surgical success rate maintained at 100% in patients less than 45 years old, and who had BMI less than 25 kg/m2 [110].
Surgical algorithm for OSA considers anatomic abnormality, disease severity, and patient preference. The balance between these three factors and morbidity of surgery must be emphasized. Riley et al. [89] developed the original sleep surgery algorithm where soft tissue and skeletal framework were targets for intervention. The updated algorithm reflects the contemporary needs of improved precision on: (1) patient phenotyping, (2) new procedures to address distinct phenotypes, and (3) improved methods for previously established operations [6,7,103]. The updated algorithm is shown in Fig. 6.
In clinic, after a thorough history, physical exam, review of PSG, and nasopharyngoscopy, the first decision is made regarding optimization of PAP or OAT use. Of note, the efficacy of upper airway surgery for OSA begins to drop as BMI increases. Bariatric surgical evaluation and treatment should precede upper airway surgery in select candidates. Use of imaging or DISE to help determine treatment course is made judiciously on an individual basis, as they are supplemental tools.
MMA and UAS have been highly effective surgical options for the treatment of OSA. Both have shown predictably high success rates with low morbidity. They differ in strengths and limitations, and may complement each other. DISE is currently required to rule out CCC of the velum for UAS. Concentric collapse and lateral pharyngeal wall collapse from DISE are associated with low success rate of soft tissue surgery [112,113]. MMA is more reliable in reversing these collapse patterns [45,105]. MMA can also be a first-line consideration for patients with any degree of OSA with dentofacial deformity. If MMA is performed first, the patient is still eligible, and may need, phase 1 procedures. UAS or PAP following MMA relapse are safe options especially in patients with advanced age [114].
The growing understanding of OSA pathophysiology, new methods for airway anatomic phenotyping, and individualized surgical modalities optimize surgical management of OSA. As comprehensive as this updated algorithm directs surgical care, the most pressing need is its integration with physiologic phenotyping.. The combination of contemporary sleep surgery with advances in modulation of arousal threshold, loop gain, and muscle tone will truly define precision in OSA care.
▪ The growing understanding of the pathophysiology of obstructive sleep apnea (OSA) and development of new methods for airway evaluation allow improved phenotyping of the upper airway and customization of surgical treatment.
▪ Dynamic airway assessment has been augmented with examination under drug-induced sedation (sleep) endoscopy.
▪ Conceptually similar to pediatric rapid maxillary expansion, distraction osteogenesis maxillary expansion effectively addresses the same anatomic phenotype in adults.
▪ Maxillomandibular advancement and upper airway stimulation have been highly effective surgical options for the treatment of OSA, but they differ in strengths and limitations, and may complement each other.
▪ The combination of contemporary sleep surgery with advances in modulation of arousal threshold, loop gain, and muscle tone will truly define precision in OSA care.
REFERENCES
1. Riley RW, Powell NB, Guilleminault C. Obstructive sleep apnea syndrome: a surgical protocol for dynamic upper airway reconstruction. J Oral Maxillofac Surg. 1993; Jul. 51(7):742–9.


2. Powell N, Riley R, Guilleminault C, Troell R. A reversible uvulopalatal flap for snoring and sleep apnea syndrome. Sleep. 1996; Sep. 19(7):593–9.


3. Riley RW, Powell NB, Guilleminault C, Nino-Murcia G. Maxillary, mandibular, and hyoid advancement: an alternative to tracheostomy in obstructive sleep apnea syndrome. Otolaryngol Head Neck Surg. 1986; Jun. 94(5):584–8.


4. Riley RW, Powell NB, Guilleminault C. Maxillofacial surgery and nasal CPAP: a comparison of treatment for obstructive sleep apnea syndrome. Chest. 1990; Dec. 98(6):1421–5.
5. Decker M, Yamauchi M, Strohl KP. Keep the airway open and let the brain sleep. Am J Respir Crit Care Med. 2014; Dec. 190(11):1207–9.


6. Liu SY, Awad M, Riley R, Capasso R. The role of the revised Stanford protocol in today’s precision medicine. Sleep Med Clin. 2019; Mar. 14(1):99–107.


7. Liu SY, Powell NB, Riley RW. Algorithm for multilevel treatment: the Riley, Powell, and Liu Stanford experience. In : Friedman M, Jacobowitz O, editors. Sleep apnea and snoring: surgical and non-surgical therapy. 2nd ed. Edinburgh: Elsevier;2020. p. 104–9.
8. Zozula R, Rosen R. Compliance with continuous positive airway pressure therapy: assessing and improving treatment outcomes. Curr Opin Pulm Med. 2001; Nov. 7(6):391–8.


9. Chervin RD, Theut S, Bassetti C, Aldrich MS. Compliance with nasal CPAP can be improved by simple interventions. Sleep. 1997; Apr. 20(4):284–9.


10. Torre C, Capasso R, Zaghi S, Williams R, Liu SY. High incidence of posterior nasal cavity obstruction in obstructive sleep apnea patients. Sleep Sci Pract. 2017; Mar. 1(1):8.


11. Camacho M, Zaghi S, Certal V, Abdullatif J, Means C, Acevedo J, et al. Inferior turbinate classification system, grades 1 to 4: development and validation study. Laryngoscope. 2015; Feb. 125(2):296–302.


12. Terris DJ, Hanasono MM, Liu YC. Reliability of the Muller maneuver and its association with sleep-disordered breathing. Laryngoscope. 2000; Nov. 110(11):1819–23.


13. Huynh NT, Morton PD, Rompre PH, Papadakis A, Remise C. Associations between sleep-disordered breathing symptoms and facial and dental morphometry, assessed with screening examinations. Am J Orthod Dentofacial Orthop. 2011; Dec. 140(6):762–70.


14. Kim JH, Guilleminault C. The nasomaxillary complex, the mandible, and sleep-disordered breathing. Sleep Breath. 2011; May. 15(2):185–93.


15. Williams R, Patel V, Chen YF, Tangbumrungtham N, Thamboo A, Most SP, et al. The upper airway nasal complex: structural contribution to persistent nasal obstruction. Otolaryngol Head Neck Surg. 2019; Jul. 161(1):171–7.


16. Goldberg AN. Obstructive sleep apnea: decision making and treatment algorithm. In : Friedman M, Jacobowitz O, editors. Sleep apnea and snoring E-Book: surgical and non-surgical therapy. Philadelphia (PA): Elsevier;2008. p. 45.
17. Punjabi NM, Newman AB, Young TB, Resnick HE, Sanders MH. Sleep-disordered breathing and cardiovascular disease: an outcomebased definition of hypopneas. Am J Respir Crit Care Med. 2008; May. 177(10):1150–5.
18. Kendzerska T, Gershon AS, Hawker G, Leung RS, Tomlinson G. Obstructive sleep apnea and risk of cardiovascular events and allcause mortality: a decade-long historical cohort study. PLoS Med. 2014; Feb. 11(2):e1001599.


19. Aurora RN, Swartz R, Punjabi NM. Misclassification of OSA severity with automated scoring of home sleep recordings. Chest. 2015; Mar. 147(3):719–27.


20. Kapoor M, Greenough G. Home sleep tests for Obstructive Sleep Apnea (OSA). J Am Board Fam Med. 2015; Jul-Aug. 28(4):504–9.


21. Nerfeldt P, Aoki F, Friberg D. Polygraphy vs. polysomnography: missing OSAs in symptomatic snorers: a reminder for clinicians. Sleep Breath. 2014; May. 18(2):297–303.
22. Watson NF. Health care savings: the economic value of diagnostic and therapeutic care for obstructive sleep apnea. J Clin Sleep Med. 2016; Aug. 12(8):1075–7.


23. Scannone A, Tosta M, Suarez A, Otero L. Cephalometric and dental measures as diagnostic tools for the obstructive sleep apnea. J Sleep Disor Treat Care. 2017; Jan. 6(4):


24. Torre C, Zaghi S, Camacho M, Capasso R, Liu SY. Hypopharyngeal evaluation in obstructive sleep apnea with awake flexible laryngoscopy: validation and updates to Cormack-Lehane and modified Cormack-Lehane scoring systems. Clin Otolaryngol. 2018; Jun. 43(3):823–7.


25. Kim JW, Kim J, Kim T, Lee K, Kim S, Bae M. Extraction of acoustic biomarkers from PSG to detect OSA. Sleep Med. 2017; Dec. 40:e160.


26. Kim WY, Hong SN, Yang SK, Nam KJ, Lim KH, Hwang SJ, et al. The effect of body position on airway patency in obstructive sleep apnea: CT imaging analysis. Sleep Breath. 2019; Sep. 23(3):911–6.


27. Huon LK, Liu SY, Shih TT, Chen YJ, Lo MT, Wang PC. Dynamic upper airway collapse observed from sleep MRI: BMI-matched severe and mild OSA patients. Eur Arch Otorhinolaryngol. 2016; Nov. 273(11):4021–6.


28. Soose RJ. Novel surgical approaches for the treatment of obstructive sleep apnea. Sleep Med Clin. 2016; Jun. 11(2):189–202.


29. Kent DT, Rogers R, Soose RJ. Drug-induced sedation endoscopy in the evaluation of OSA patients with incomplete oral appliance therapy response. Otolaryngol Head Neck Surg. 2015; Aug. 153(2):302–7.


30. Vroegop AV, Vanderveken OM, Verbraecken JA. Drug-induced sleep endoscopy: evaluation of a selection tool for treatment modalities for obstructive sleep apnea. Respiration. 2020; 99(5):451–7.


31. Glen JB. The development of ‘Diprifusor’: a TCI system for propofol. Anaesthesia. 1998; Apr. 53 Suppl 1:13–21.


32. Hillman DR, Walsh JH, Maddison KJ, Platt PR, Kirkness JP, Noffsinger WJ, et al. Evolution of changes in upper airway collapsibility during slow induction of anesthesia with propofol. Anesthesiology. 2009; Jul. 111(1):63–71.


33. Eastwood PR, Platt PR, Shepherd K, Maddison K, Hillman DR. Collapsibility of the upper airway at different concentrations of propofol anesthesia. Anesthesiology. 2005; Sep. 103(3):470–7.


34. Chattopadhyay U, Mallik S, Ghosh S, Bhattacharya S, Bisai S, Biswas H. Comparison between propofol and dexmedetomidine on depth of anesthesia: a prospective randomized trial. J Anaesthesiol Clin Pharmacol. 2014; Oct. 30(4):550–4.


35. Charakorn N, Kezirian EJ. Drug-induced sleep endoscopy. Otolaryngol Clin North Am. 2016; Dec. 49(6):1359–72.


36. Croft CB, Pringle M. Sleep nasendoscopy: a technique of assessment in snoring and obstructive sleep apnoea. Clin Otolaryngol Allied Sci. 1991; Oct. 16(5):504–9.
37. Kezirian EJ, Hohenhorst W, de Vries N. Drug-induced sleep endoscopy: the VOTE classification. Eur Arch Otorhinolaryngol. 2011; Aug. 268(8):1233–6.


38. Friedman M, Ibrahim H, Bass L. Clinical staging for sleep-disordered breathing. Otolaryngol Head Neck Surg. 2002; Jul. 127(1):13–21.


39. Iwanaga K, Hasegawa K, Shibata N, Kawakatsu K, Akita Y, Suzuki K, et al. Endoscopic examination of obstructive sleep apnea syndrome patients during drug-induced sleep. Acta Otolaryngol Suppl. 2003; (550):36–40.


40. Vicini C, de Vito A, Benazzo M, Frassineti S, Campanini A, Frasconi P, et al. The nose oropharynx hypopharynx and larynx (NOHL) classification: a new system of diagnostic standardized examination for OSAHS patients. Eur Arch Otorhinolaryngol. 2012; Apr. 269(4):1297–300.


41. Vroegop AV, Vanderveken OM, Boudewyns AN, Scholman J, Saldien V, Wouters K, et al. Drug-induced sleep endoscopy in sleep-disordered breathing: report on 1,249 cases. Laryngoscope. 2014; Mar. 124(3):797–802.


42. Ravesloot MJ, de Vries N. One hundred consecutive patients undergoing drug-induced sleep endoscopy: results and evaluation. Laryngoscope. 2011; Dec. 121(12):2710–6.


43. Vanderveken OM, Maurer JT, Hohenhorst W, Hamans E, Lin HS, Vroegop AV, et al. Evaluation of drug-induced sleep endoscopy as a patient selection tool for implanted upper airway stimulation for obstructive sleep apnea. J Clin Sleep Med. 2013; May. 9(5):433–8.


44. Zhang P, Ye J, Pan C, Sun N, Kang D. The role of obstruction length and height in predicting outcome of velopharyngeal surgery. Otolaryngol Head Neck Surg. 2015; Jul. 153(1):144–9.


45. Liu SY, Huon LK, Iwasaki T, Yoon A, Riley R, Powell N, et al. Efficacy of maxillomandibular advancement examined with drug-induced sleep endoscopy and computational fluid dynamics airflow modeling. Otolaryngol Head Neck Surg. 2016; Jan. 154(1):189–95.


46. Lavie P. Rediscovering the importance of nasal breathing in sleep or, shut your mouth and save your sleep. J Laryngol Otol. 1987; Jun. 101(6):558–63.


47. Busaba NY. The nose in snoring and obstructive sleep apnea. Curr Opin Otolaryngol Head Neck Surg. 1999; Feb. 7(1):11.


48. Olsen KD, Kern EB, Westbrook PR. Sleep and breathing disturbance secondary to nasal obstruction. Otolaryngol Head Neck Surg. 1981; Sep-Oct. 89(5):804–10.


49. Cole P, Haight JS. Mechanisms of nasal obstruction in sleep. Laryngoscope. 1984; Dec. 94(12 Pt 1):1557–9.


50. Verse T, Maurer JT, Pirsig W. Effect of nasal surgery on sleep-related breathing disorders. Laryngoscope. 2002; Jan. 112(1):64–8.


51. Camacho M, Riaz M, Capasso R, Ruoff CM, Guilleminault C, Kushida CA, et al. The effect of nasal surgery on continuous positive airway pressure device use and therapeutic treatment pressures: a systematic review and meta-analysis. Sleep. 2015; Feb. 38(2):279–86.


52. Trindade SH, Tagliarini JV, Elly I, Trindade K, Weber SA. Nasal septoplasty in patients with obstructive sleep apnea syndrome: effects on polysomnographic parameters. J Sleep Med Disord. 2017; Jun. 4(2):1077.
53. Elhabashy M, Abdelfatah A, Abdelaziz A, Omar H, Abdelwhab S. Effectiveness of septoplasty with or without inferior turbinate reduction in patients with obstructive sleep apnea. Eur Respir J. 2019; 54(Suppl 63):PA4163.


54. Friedman M, Salapatas AM. Algorithm for multilevel treatment: Friedman experience. In : Friedman M, Jacobowitz O, editors. Sleep apnea and snoring: surgical and non-surgical therapy. 2nd ed. Edinburgh: Elsevier;2020. p. 120–6.
55. Abdelwahab M, Yoon A, Okland T, Poomkonsarn S, Gouveia C, Liu SY. Impact of distraction osteogenesis maxillary expansion on the internal nasal valve in obstructive sleep apnea. Otolaryngol Head Neck Surg. 2019; Aug. 161(2):362–7.


56. Iwasaki T, Yoon A, Guilleminault C, Yamasaki Y, Liu SY. How does distraction osteogenesis maxillary expansion (DOME) reduce severity of obstructive sleep apnea? Sleep Breath. 2020; Mar. 24(1):287–96.


57. Liu SY, Guilleminault C, Huon LK, Yoon A. Distraction Osteogenesis Maxillary Expansion (DOME) for adult obstructive sleep apnea patients with high arched palate. Otolaryngol Head Neck Surg. 2017; Aug. 157(2):345–8.


58. Yoon A, Guilleminault C, Zaghi S, Liu SY. Distraction Osteogenesis Maxillary Expansion (DOME) for adult obstructive sleep apnea patients with narrow maxilla and nasal floor. Sleep Med. 2020; Jan. 65:172–6.


59. Camacho M, Chang ET, Song SA, Abdullatif J, Zaghi S, Pirelli P, et al. Rapid maxillary expansion for pediatric obstructive sleep apnea: a systematic review and meta-analysis. Laryngoscope. 2017; Jul. 127(7):1712–9.


60. Iwasaki T, Saitoh I, Takemoto Y, Inada E, Kanomi R, Hayasaki H, et al. Improvement of nasal airway ventilation after rapid maxillary expansion evaluated with computational fluid dynamics. Am J Orthod Dentofacial Orthop. 2012; Mar. 141(3):269–78.


61. Iwasaki T, Takemoto Y, Inada E, Sato H, Suga H, Saitoh I, et al. The effect of rapid maxillary expansion on pharyngeal airway pressure during inspiration evaluated using computational fluid dynamics. Int J Pediatr Otorhinolaryngol. 2014; Aug. 78(8):1258–64.


62. Villa MP, Malagola C, Pagani J, Montesano M, Rizzoli A, Guilleminault C, et al. Rapid maxillary expansion in children with obstructive sleep apnea syndrome: 12-month follow-up. Sleep Med. 2007; Mar. 8(2):128–34.


63. Ishman SL, Ishii LE, Gourin CG. Temporal trends in sleep apnea surgery: 1993-2010. Laryngoscope. 2014; 124(5):1251–8.


64. Camacho M, Nesbitt NB, Lambert E, Song SA, Chang ET, Liu SY, et al. Laser-assisted uvulopalatoplasty for obstructive sleep apnea: a systematic review and meta-analysis. Sleep. 2017; Mar. 40(3):


65. Thaler ER, Rassekh CH, Lee JM, Weinstein GS, O’Malley BW Jr. Outcomes for multilevel surgery for sleep apnea: obstructive sleep apnea, transoral robotic surgery, and uvulopalatopharyngoplasty. Laryngoscope. 2016; Jan. 126(1):266–9.


66. Lin HC, Friedman M, Chang HW, Gurpinar B. The efficacy of multilevel surgery of the upper airway in adults with obstructive sleep apnea/hypopnea syndrome. Laryngoscope. 2008; May. 118(5):902–8.


67. Li HY. Palatal surgery for obstructive sleep apnea: from ablation to reconstruction. Sleep Med Clin. 2019; Mar. 14(1):51–8.
68. Awad M, Gouveia C, Capasso R, Liu SY. Tonsillectomy and pharyngoplasty: tissue-preserving techniques. Atlas Oral Maxillofac Surg Clin North Am. 2019; Mar. 27(1):17–22.
69. Camacho M, Zaghi S, Piccin O, Certal V. Expansion sphincter pharyngoplasty for obstructive sleep apnea: an update to the recent meta-analysis. Eur Arch Otorhinolaryngol. 2016; Sep. 273(9):2857–8.


70. Vicini C, Hendawy E, Campanini A, Eesa M, Bahgat A, AlGhamdi S, et al. Barbed reposition pharyngoplasty (BRP) for OSAHS: a feasibility, safety, efficacy and teachability pilot study: “we are on the giant’s shoulders”. Eur Arch Otorhinolaryngol. 2015; Oct. 272(10):3065–70.


71. Pang KP, Plaza G, Baptista J PM, O’Connor Reina C, Chan YH, Pang KA, et al. Palate surgery for obstructive sleep apnea: a 17-year meta-analysis. Eur Arch Otorhinolaryngol. 2018; Jul. 275(7):1697–707.


72. Hsu YS, Jacobowitz O. Does sleep endoscopy staging pattern correlate with outcome of advanced palatopharyngoplasty for moderate to severe obstructive sleep apnea? J Clin Sleep Med. 2017; Oct. 13(10):1137–44.


73. Pang KP, Woodson BT. Expansion sphincter pharyngoplasty: a new technique for the treatment of obstructive sleep apnea. Otolaryngol Head Neck Surg. 2007; Jul. 137(1):110–4.


74. Cahali MB, Formigoni GG, Gebrim EM, Miziara ID. Lateral pharyngoplasty versus uvulopalatopharyngoplasty: a clinical, polysomnographic and computed tomography measurement comparison. Sleep. 2004; Aug. 27(5):942–50.


75. Woodson BT, Robinson S, Lim HJ. Transpalatal advancement pharyngoplasty outcomes compared with uvulopalatopharygoplasty. Otolaryngol Head Neck Surg. 2005; Aug. 133(2):211–7.


76. Jacobowitz O. Algorithm for surgery: palatopharyngoplasty first and foremost. In : Friedman M, Jacobowitz O, editors. Sleep apnea and snoring: surgical and non-surgical therapy. 2nd ed. Edinburgh: Elsevier;2020. p. 99–103.
77. Liu SY, Hutz MJ, Poomkonsarn S, Chang CP, Awad M, Capasso R. Palatopharyngoplasty resolves concentric collapse in patients ineligible for upper airway stimulation. Laryngoscope. 2020; Feb. 28. [Epub]. https://doi.org/10.1002/lary.28595.


78. Campanini A, Canzi P, de Vito A, Dallan I, Montevecchi F, Vicini C. Awake versus sleep endoscopy: personal experience in 250 OSAHS patients. Acta Otorhinolaryngol Ital. 2010; Apr. 30(2):73–7.
79. Friedman M, Soans R, Gurpinar B, Lin HC, Joseph N. Evaluation of submucosal minimally invasive lingual excision technique for treatment of obstructive sleep apnea/hypopnea syndrome. Otolaryngol Head Neck Surg. 2008; Sep. 139(3):378–85.


80. Li HY, Lee LA, Kezirian EJ. Coblation endoscopic lingual lightening (CELL) for obstructive sleep apnea. Eur Arch Otorhinolaryngol. 2016; Jan. 273(1):231–6.


81. Montevecchi F, Cammaroto G, Meccariello G, Hoff PT, Corso RM, Galletti C, et al. Transoral robotic surgery (TORS): a new tool for high risk tracheostomy decannulation. Acta Otorhinolaryngol Ital. 2017; Feb. 37(1):46–50.


82. Cammaroto G, Meccariello G, Costantini M, Stomeo F, Hoff P, Montevecchi F, et al. Trans-oral robotic tongue reduction for OSA: does lingual anatomy influence the surgical outcome? J Clin Sleep Med. 2018; Aug. 14(8):1347–51.


83. Camacho M, Certal V, Capasso R. Comprehensive review of surgeries for obstructive sleep apnea syndrome. Braz J Otorhinolaryngol. 2013; Nov-Dec. 79(6):780–8.


84. Justin GA, Chang ET, Camacho M, Brietzke SE. Transoral robotic surgery for obstructive sleep apnea: a systematic review and meta-analysis. Otolaryngol Head Neck Surg. 2016; May. 154(5):835–46.
85. Vicini C, Montevecchi F, Meccariello G, Cammaroto G. Base of tongue surgery. In : Salman SO, editor. Modern management of obstructive sleep apnea. 1st ed. Cham: Springer;2019. p. 59–68.
86. Chan JY, Wong EW, Tsang RK, Holsinger FC, Tong MC, Chiu PW, et al. Early results of a safety and feasibility clinical trial of a novel single-port flexible robot for transoral robotic surgery. Eur Arch Otorhinolaryngol. 2017; Nov. 274(11):3993–6.


87. Chan JY, Holsinger FC, Liu S, Sorger JM, Azizian M, Tsang RK. Augmented reality for image guidance in transoral robotic surgery. J Robot Surg. 2020; Aug. 14:579–83.


88. Song SA, Chang ET, Certal V, Del Do M, Zaghi S, Liu SY, et al. Genial tubercle advancement and genioplasty for obstructive sleep apnea: a systematic review and meta-analysis. Laryngoscope. 2017; Apr. 127(4):984–92.


89. Riley RW, Powell NB, Guilleminault C. Obstructive sleep apnea syndrome: a review of 306 consecutively treated surgical patients. Otolaryngol Head Neck Surg. 1993; Feb. 108(2):117–25.


90. Liu SY, Huon LK, Zaghi S, Riley R, Torre C. An accurate method of designing and performing individual-specific genioglossus advancement. Otolaryngol Head Neck Surg. 2017; Jan. 156(1):194–7.


91. Cheng A. Genioglossus and genioplasty advancement. Atlas Oral Maxillofac Surg Clin North Am. 2019; Mar. 27(1):23–8.


92. Oliven A, Odeh M, Geitini L, Oliven R, Steinfeld U, Schwartz AR, et al. Effect of coactivation of tongue protrusor and retractor muscles on pharyngeal lumen and airflow in sleep apnea patients. J Appl Physiol (1985). 2007; Nov. 103(5):1662–8.


93. Dedhia RC, Strollo PJ, Soose RJ. Upper airway stimulation for obstructive sleep apnea: past, present, and future. Sleep. 2015; Jun. 38(6):899–906.


94. Strollo PJ Jr, Soose RJ, Maurer JT, de Vries N, Cornelius J, Froymovich O, et al. Upper-airway stimulation for obstructive sleep apnea. N Engl J Med. 2014; Jan. 370(2):139–49.


95. Costantino A, Rinaldi V, Moffa A, Luccarelli V, Bressi F, Cassano M, et al. Hypoglossal nerve stimulation long-term clinical outcomes: a systematic review and meta-analysis. Sleep Breath. 2020; Jun. 24(2):399–411.


96. Hofauer B, Steffen A, Knopf A, Hasselbacher K, Heiser C. Adherence to upper-airway stimulation in the treatment of OSA. Chest. 2018; Feb. 153(2):574–5.


97. Bohorquez D, Mahmoud AF, Yu JL, Thaler ER. Upper airway stimulation therapy and sleep architecture in patients with obstructive sleep apnea. Laryngoscope. 2020; Apr. 130(4):1085–9.


98. Powell N, Riley RW. A surgical protocol for sleep disordered breathing. Oral Maxillofac Surg Clin North Am. 1995; May. 7(3):345–56.


99. Riley RW, Powell NB. Maxillofacial surgery and obstructive sleep apnea syndrome. Otolaryngol Clin North Am. 1990; Aug. 23(4):809–26.
100. Riley RW, Powell NB, Guilleminault C. Maxillary, mandibular, and hyoid advancement for treatment of obstructive sleep apnea: a review of 40 patients. J Oral Maxillofac Surg. 1990; Jan. 48(1):20–6.


101. Vicini C, Dallan I, Campanini A, de Vito A, Barbanti F, Giorgiomarrano G, et al. Surgery vs ventilation in adult severe obstructive sleep apnea syndrome. Am J Otolaryngol. 2010; Jan-Feb. 31(1):14–20.


102. Camacho M, Liu SY, Certal V, Capasso R, Powell NB, Riley RW. Large maxillomandibular advancements for obstructive sleep apnea: an operative technique evolved over 30 years. J Craniomaxillofac Surg. 2015; Sep. 43(7):1113–8.
103. Liu SY, Awad M, Riley RW. Maxillomandibular advancement: contemporary approach at Stanford. Atlas Oral Maxillofac Surg Clin North Am. 2019; Mar. 27(1):29–36.
104. Boyd SB. Management of obstructive sleep apnea by maxillomandibular advancement. Oral Maxillofac Surg Clin North Am. 2009; Nov. 21(4):447–57.


105. Liu SY, Huon LK, Powell NB, Riley R, Cho HG, Torre C, et al. Lateral pharyngeal wall tension after maxillomandibular advancement for obstructive sleep apnea is a marker for surgical success: observations from drug-induced sleep endoscopy. J Oral Maxillofac Surg. 2015; Aug. 73(8):1575–82.


106. Chen YF, Ko EC, Zaghi S, Yoon A, Williams R, Riley R, et al. Optimizing mandibular sagittal split of large maxillomandibular advancements for obstructive sleep apnea: patient and surgical factors. Clin Oral Investig. 2020; Mar. 24(3):1359–67.


107. Holty JE, Guilleminault C. Maxillomandibular advancement for the treatment of obstructive sleep apnea: a systematic review and meta-analysis. Sleep Med Rev. 2010; Oct. 14(5):287–97.


108. Zaghi S, Holty JE, Certal V, Abdullatif J, Guilleminault C, Powell NB, et al. Maxillomandibular advancement for treatment of obstructive sleep apnea: a meta-analysis. JAMA Otolaryngol Head Neck Surg. 2016; Jan. 142(1):58–66.
109. Riley RW, Powell NB, Li KK, Troell RJ, Guilleminault C. Surgery and obstructive sleep apnea: long-term clinical outcomes. Otolaryngol Head Neck Surg. 2000; Mar. 122(3):415–21.


110. Vigneron A, Tamisier R, Orset E, Pepin JL, Bettega G. Maxillomandibular advancement for obstructive sleep apnea syndrome treatment: long-term results. J Craniomaxillofac Surg. 2017; Feb. 45(2):183–91.


111. Boyd SB, Chigurupati R, Cillo JE Jr, Eskes G, Goodday R, Meisami T, et al. Maxillomandibular advancement improves multiple health-related and functional outcomes in patients with obstructive sleep apnea: a multicenter study. J Oral Maxillofac Surg. 2019; Feb. 77(2):352–70.


112. Green KK, Kent DT, D’Agostino MA, Hoff PT, Lin HS, Soose RJ, et al. Drug-induced sleep endoscopy and surgical outcomes: a multicenter cohort study. Laryngoscope. 2019; Mar. 129(3):761–70.


Fig. 1.
Obstructive sleep apnea patient with high-arched palate (A) and narrow nasal floor (B). Narrow and high-arched hard palate indicates a disproportion between the volume of tongue and the size of the oral cavity.
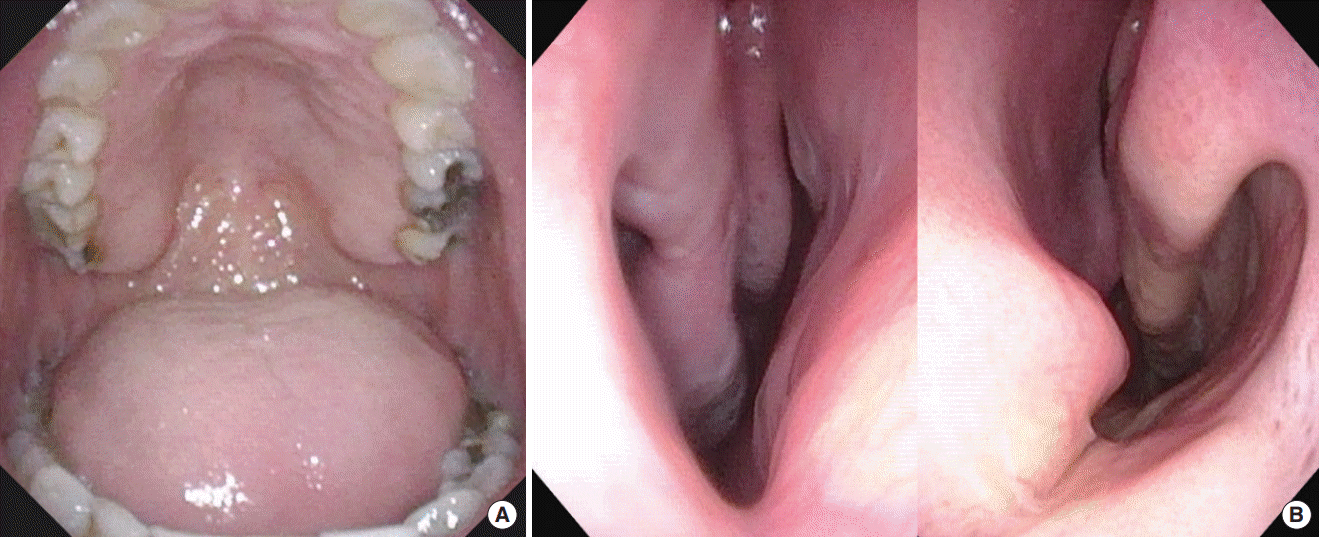
Fig. 2.
Distraction Osteogenesis Maxillary Expansion: maxillary expansion results in widening of nasal floor and internal nasal valve.
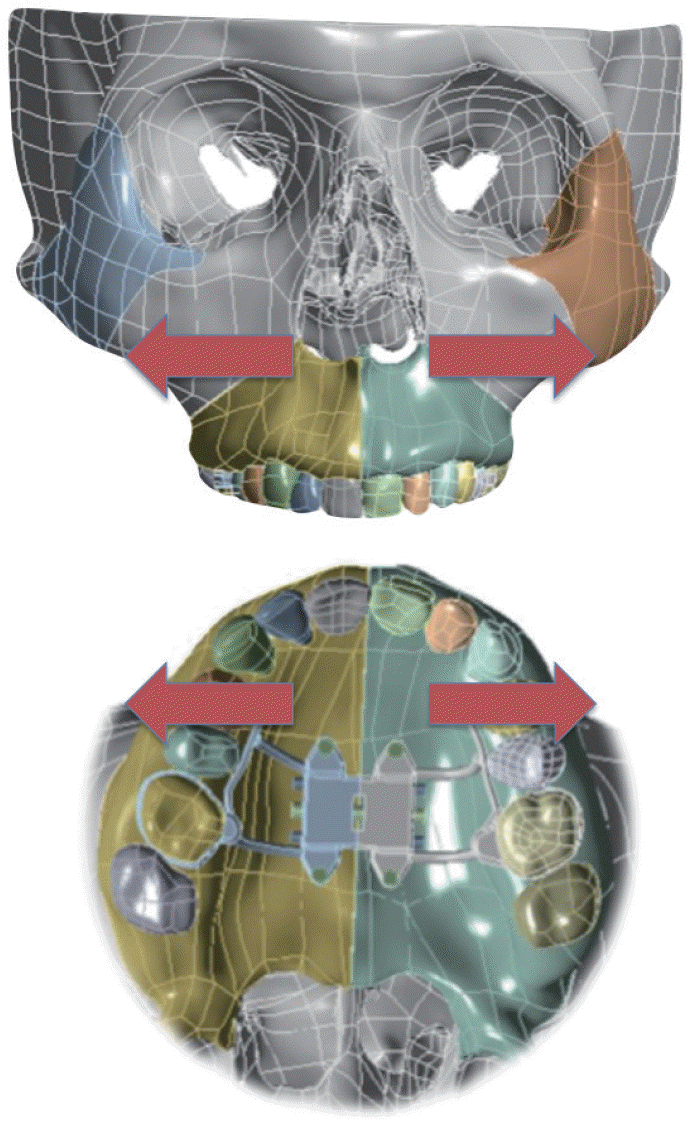
Fig. 3.
Virtual surgical planning of genioglossus advancement: the osteotomy guide is designed to capture the genial tubercle.
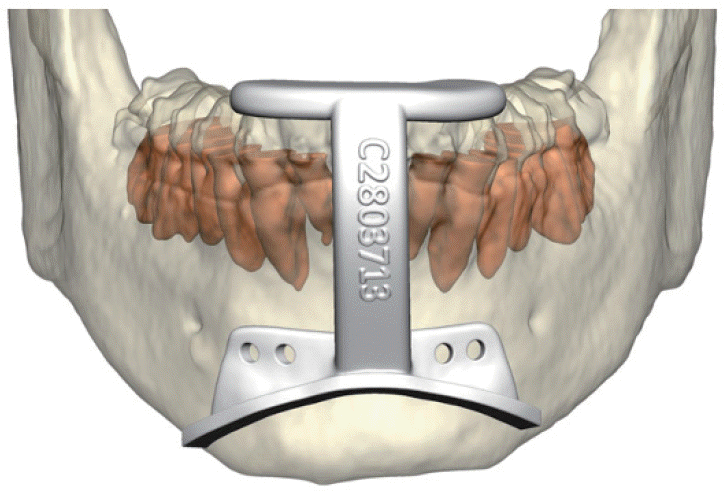
Fig. 4.
Upper airway stimulation (UAS): the medial branch of hypoglossal nerve is identified (A), sensing lead is placed between internal and external intercostal muscles (B).
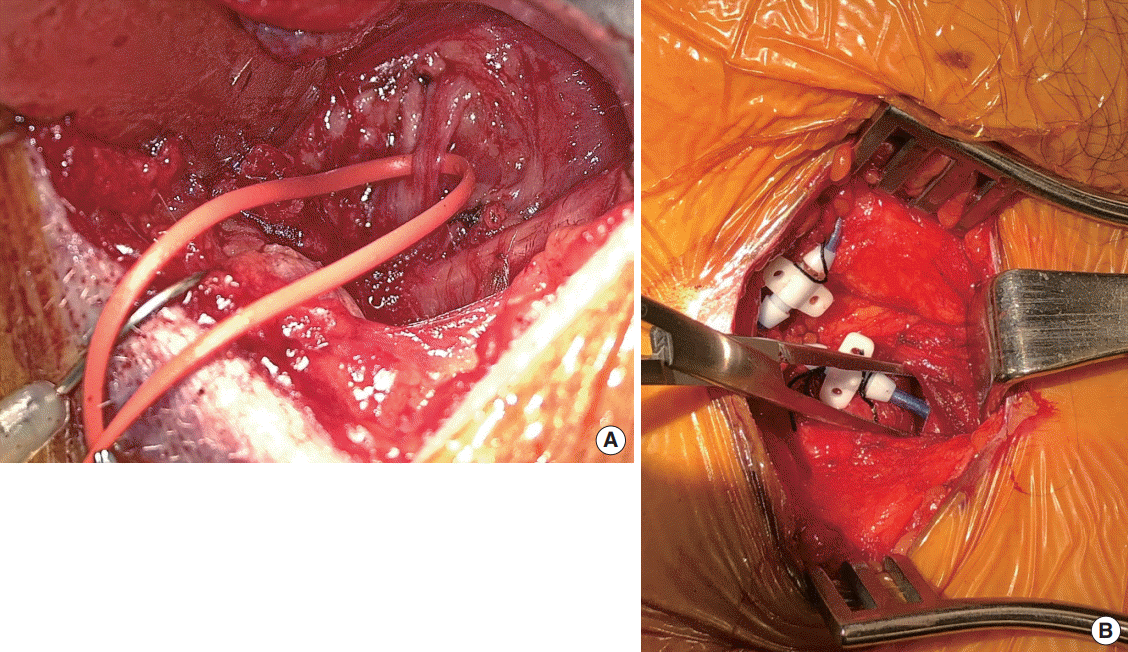
Fig. 5.
Counterclockwise maxillomandibular advancement allows a greater advancement of mandible than maxilla, to maximize airway and facial esthetics.
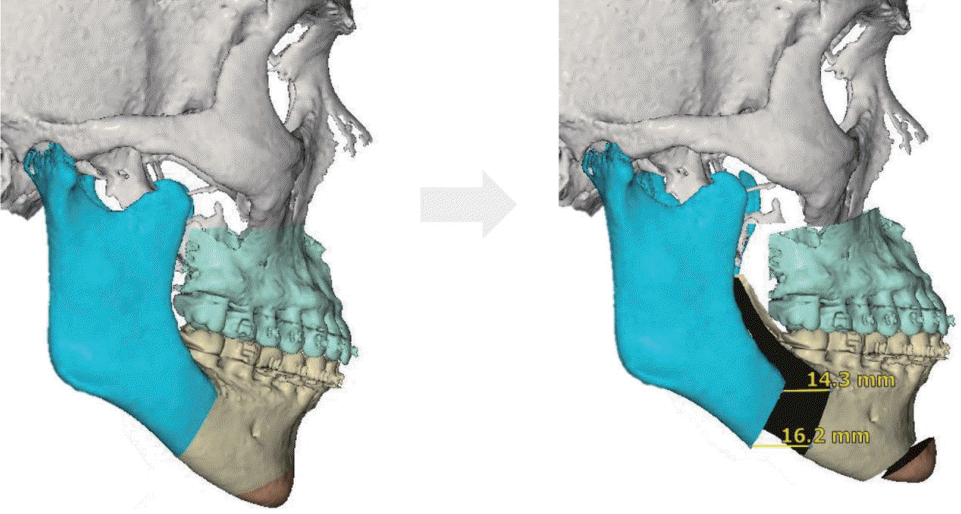
Fig. 6.
Updated Stanford sleep surgery algorithm. PE, physical examination; PSG, polysomnography; BMI, body mass index; PAP, positive airway pressure; OAT, oral appliance therapy; NP, nasopharyngoscopy; DISE, drug-induced sedation (sleep) endoscopy; CCC, complete concentric collapse; LPW, lateral pharyngeal wall; DOME, distraction osteogenesis maxillary expansion; TBR, tongue base reduction; TORS, transoral robotic surgery; GGA, genioglossus advancement; UAS, upper airway stimulation.
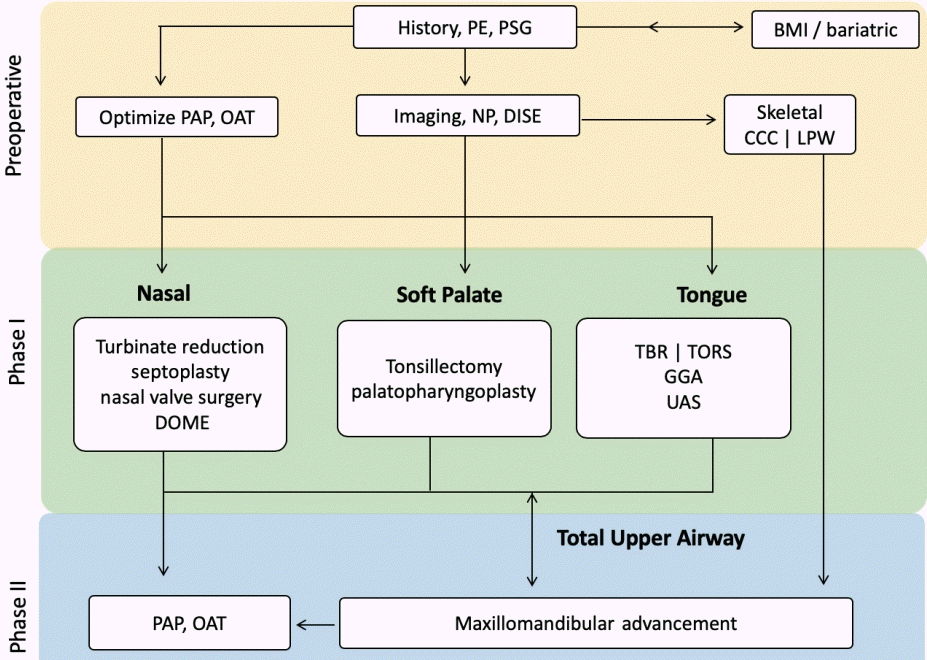