Abstract
Objectives
Trichostatin A (TSA), an inhibitor of histone deacetylase, has been shown to play an important role in attenuating asthmatic inflammation. However, the effect of TSA in allergic rhinitis is not known. The aims of this study were to investigate the effect of TSA on allergic nasal inflammation and on the induction of regulatory T cells in a murine model of allergic rhinitis.
Methods
BALB/c mice were sensitized intraperitoneally with ovalbumin (OVA) and then challenged intranasally with OVA. TSA (1 mg/kg) was given to the treatment group, and multiple parameters of allergic responses were evaluated to determine the effects of TSA on allergic rhinitis. Allergic nasal symptom scores, including frequency of rubbing and sneezing, were checked. Eosinophil infiltrations were stained with Chromotrope 2R, and the expression levels of OVA-specific IgE, T-helper 1 (Th1) cytokine (interferon-gamma [IFN-γ]), Th2 cytokines (interleukin [IL] 4 and IL-5) and Treg (Foxp3, IL-10, and transforming growth factor-beta [TGF-β]) were measured by quantitative reverse transcription-polymerase chain reaction or enzyme-linked immunosorbent assay.
Results
TSA reduced the scores of allergic nasal symptoms and the amount of eosinophil infiltration into the nasal mucosa. TSA suppressed OVA-specific IgE levels and reduced expression of the IL-4 and IL-5. However, the expression of IFN-γ was unchanged in the treatment group. The levels of Foxp3, IL-10, and TGF-β were increased in pretreatment with TSA as compared to control group.
Allergic rhinitis is a common inflammatory disease of the nasal mucosa that is characterized by pruritus, sneezing, rhinorrhea, and nasal obstruction. The prevalence of allergic rhinitis has continually increased in the developed world over the last decades [1]. Allergic rhinitis is one of type I allergic disorders characterized by an aberrant immune response to environmental antigens and is caused by an IgE-mediated humoral immune response [23]. It is characterized by an infiltration of interleukin-producing inflammatory cells, including eosinophils and T-helper 2 (Th2) cells, that produce interleukins interleukin [IL] 4, IL-5, and IL-13 [4]. The production of these cytokines is the initial event and the key factor in the development of allergic responses. In addition, eosinophilic infiltration contributes to persistent nasal inflammation, remodeling and nasal hyperresponsiveness in patients with allergic rhinitis [56].
Epigenetics, DNA methylation and chromatin modifications, are critical for generating cellular diversity and maintaining distinct gene expression profiles. Histone acetylation, one type of chromatin modification, is regulated by histone acetyltransferase and histone deacetylase (HDAC) [78]. Histone acetyltransferase permits gene accessibility to transcription factors, allowing for gene expression. However, HDAC leads to gene silencing, which is caused by a condensation of the DNA structure. The balance of histone acetyltransferase and HDAC has been shown to regulate the expression of target genes. Among the HDACs, HDAC2 activity has been shown to be decreased in peripheral lung and bronchial biopsies and in alveolar macrophages from chronic obstructive pulmonary disease patients [8]. Using specific reversible trichostatin A (TSA), a known HDAC inhibitor, the relationship between histone acetylation status and the control of gene expression has been elucidated in nasal tissue [910].
Regulatory T cells (Tregs) effect the suppressive properties of the T-cell response, and act to suppress autoreactive lymphocytes in order to control innate and adaptive immunity during inflammation [11]. Tregs are Foxp3+CD4+CD25+ T cells that secrete inhibitory cytokines such as IL-10 and transforming growth factor-beta (TGF-β). The inhibitory cytokines IL-10 and TGF-β, in turn, can induce Tregs from naive CD4+ T cells and suppress other types of immune cells [12]. Tregs employ a broad range of soluble and membrane-bound suppressor factors, such as IL-10, TGF-β, cyototoxic T lymphocyte antigen-4, or histamine receptor 2 [1213].
According to recent findings, TSA attenuates airway inflammation in mouse models of asthma. However, the inhibitory effect of TSA on inflammation in a murine model of allergic rhinitis has yet to be described. In the present study, we have examined whether TSA inhibits ovalbumin (OVA)-induced allergic nasal symptoms and inflammatory cytokines in a murine model of allergic rhinitis.
TSA and OVA (grade V) were purchased from Sigma (St. Louis, MO, USA). TSA was dissolved in dimethylsulfoxide and then diluted to the desired concentrations.
All animal experiments in this study followed the guidelines for Institutional Animal Care at the Clinical Research Institute of Korea University Guro Hospital. Female BALB/c mice (weight, 18-22 g; Orient, Seongnam, Korea) were divided into three groups (6 mice/group). Control mice were sensitized and challenged with phosphate buffered saline (PBS, negative control); OVA mice were sensitized and challenged with OVA; TSA-treated, OVA+TSA mice were sensitized and treated with TSA, then challenged with OVA (OVA+TSA). Mice were sensitized with 25-µg OVA with or without 2 mg of alum hydroxide (Imject Alum, Thermo Scientific, Waltham, MA, USA) dissolved in PBS, and a total of 300 µL was injected intraperitoneally on days 0, 7, and 14. Five days after the final injection of OVA, mice were injected with TSA (1 mg/kg) by an intraperitoneal injection for six days. One week after the final injection, mice were challenged by intranasal instillation with 200 µg of OVA solubilized in PBS (10 µL/nostril) once daily for 4 days. Control mice were treated by intranasal instillation with PBS. All mice were sacrificed 24 hours after the final instillation (Fig. 1).
Nasal symptoms were evaluated by counting the frequency of nasal rubbing and sneezing that occurred in the 30-minute observation period. Assessors were blinded to the treatment protocol.
The transtracheal technique was performed on mice 24 hours after the final allergen challenge to obtain nasal lavage samples. A polyethylene 22-gauge catheter connected to a syringe was inserted intratracheally into the choana following the tracheotomy of each mouse. One-mL aliquot of PBS at 37℃ was slowly injected into each of the nasal cavities, and nasal lavage fluid was collected from the nares.
All tissues are fixed with 4% paraformaldehyde overnight and embedded in paraffin. Sections were mounted on slides, deparaffinized with xylene, rehydrated through graded alcohols, and stained with Chromotrope 2R (Sigma). The stained slides were dehydrated, mounted, and observed under a light microscope (Olympus IX71, Olympus, Tokyo, Japan). Chromotrope 2R-positive cells were counted blind in a coded random order by two observers. The total number of Chromotrope 2R-positive cells was counted in high-power fields (×400).
Total RNA from spleen tissues was extracted using NucleoSpin RNA II (Macherey-Nagel, Düren, Germany) according to the manufacturer's instructions. Two micrograms of RNA was reverse-transcribed with ReverTra Ace qPCR RT Kit (Toyobo, Osaka, Japan) following the manufacturer's protocols. The primer pairs used for targeting specific genes as follows: IFN-γ (sense sequence, 5'-CGG CAC AGT CAT TGA AAG CCT A-3'; anti-sense sequence, 5'-GTT GCT GAT GGC CTG ATT GTC T-3'; 199 bp), IL-4 (sense sequence, 5'- TCG GCA TTT TGA ACG AGG TC -3'; antisense sequence, 5'- CGA AAA GCC CGA AAG AGT CTC-3'; 217 bp), IL-5 (sense sequence, 5'-CTC ACC GAG CTC TGT TGA CAA-3'; antisense sequence, 5'-CCA CAC TTC TCT TTT TGG CGG-3'; 202 bp), Foxp3 (sense sequence, 5'- CCC ATC CCC AGG AGT CTT G -3'; antisense sequence, 5'- ACC ATG ACT AGG GGC ACT GTA -3'; 183 bp), GAPDH (sense sequence, 5'-GTA TGA TGA CAT CAA GAA GGT GGT G-3'; antisense sequence, 5'-ATA CCA GGA AAT GAG CTT GAC AAA G-3'; 181 bp). The cDNA were amplified with an initial 5-minute denaturation step at 94℃, followed by 30 cycles at 94℃ for 45 seconds, 55℃-65℃ for 45 seconds, 72℃ for 45 seconds and an extension step at 74℃ for 5 minutes. PCR products were visualized in 2% agarose gel and analyzed with Molecular Imager ChemiDoc XRS+ (Bio-Rad, Hercules, CA, USA).
The levels of OVA-specific IgE in the serum were measured using an ELISA kit (BioLegend Inc., San Diego, CA, USA). The levels of IFN-γ for the Th1 cytokine and IL-4 and IL-5 for the Th2 cytokines in nasal lavage fluid were measured using IFN-γ, IL-4, and IL-5 ELISA quantitation kits (R&D systems, Minneapolis, MN, USA). The levels of IL-10 and TGF-β for the Treg cytokines were determined by ELISA quantitation kits.
The statistical significance of the differences between control and experimental data was analyzed using unpaired t-tests or one-way analysis of variance followed by Tukey test (GraphPad Prism ver. 5, GraphPad Software Inc., San Diego, CA, USA). Significance was established at the 95% confidence level. Values of P<0.05 were accepted as statistically significant.
The frequency of nasal rubbing and sneezing increased significantly in the OVA treatment group versus the control group. However, the OVA+TSA group exhibited a significant decrease in the frequency of nasal rubbing and sneezing compared to the group undergoing treatment with OVA only (Fig. 2), demonstrating that TSA inhibits OVA-induced allergic nasal symptoms.
Serum OVA-specific IgE levels were increased in the OVA group compared to levels in the control. OVA-specific IgE levels of OVA+TSA group were decreased compared to OVA group. These results demonstrate that TSA significantly reduced serum OVA-specific IgE levels (Fig. 3).
Histological analyses of nasal mucosa from the OVA group revealed typical pathologic features of allergic rhinitis-eosinophil infiltration of the nasal mucosa (Fig. 4A). The number of eosinophils in nasal mucosa was significantly elevated in the OVA group compared to the control group. A significant reduction in eosinophil numbers was observed in the mucosa from OVA+TSA group (Fig. 4B).
In the evaluation of the allergic reaction, level of the Th1 cytokine IFN-γ showed no significant difference in all groups (Fig. 5A, B). Allergic asthmatic inflammation and remodeling are caused by the secretion of a series of Th2 cytokines, particularly IL-4 and IL-5. Therefore, we examined whether the levels of these cytokine were altered in OVA+TSA-treated mice. IL-4 and IL-5 levels in the OVA group were significantly higher than in the control group. However, the levels of IL-4 and IL-5 cytokines were significantly reduced in the OVA+TSA group (Fig. 5C-F).
Treg cells express CD4, CD25, and Foxp3. Foxp3 plays as the key transcription factor controlling Treg development and function. To investigate effect of TSA on Treg cells, we measured the mRNA expression level of Foxp3. The mRNA expression level of Foxp3 was higher in the OVA group compared to the control group, and also the mRNA of Foxp3 more significantly increased in OVA+TSA group compared to the OVA group (Fig. 6A). The immunosuppressive cytokines TGF-β and IL-10 have also been implicated in the function of Tregs. To determine effect of TSA on Treg cells, we evaluated the expression levels of TGF-β and IL-10 in each treatment group. The expression levels of TGF-β and IL-10 significantly increased in the OVA+TSA group compared to the control group (Fig. 6B, C).
In this study, we have shown that TSA inhibits OVA-induced inflammation in a murine model of allergic rhinitis. TSA inhibited allergic nasal symptoms, including rubbing and sneezing, and reduced the numbers of eosinophils in nasal mucosa. TSA decreased OVA-specific IgE, IL-4, and IL-5 levels. Furthermore, OVA-induced cytokine levels of Tregs were significantly increased further by TSA treatment.
Allergic rhinitis has been defined as an inflammatory disease of the nasal mucosa, and is characterized by the following symptoms: nasal obstruction, sneezing, and rhinorrhea [12]. The symptoms of allergic rhinitis arise as a result of an IgE-mediated inflammation of the nasal mucosa, which is distinguished by an infiltration of inflammatory cells such as eosinophils and lymphocytes. Eosinophils are considered to be the major inflammatory cell type contributing to allergic rhinitis, since they release proinflammatory mediators such as leukotriene C4 and platelet-activating factor, which increase permeability and are a potent chemotactic factor for further eosinophil infiltration. Eosinophils also produce a number of potent, highly positively charged proteins that are toxic to the respiratory epithelium [5]. Th2 cells produce Th2 cytokines, which include IL-4, IL-5, and IL-13 [4]. IL-4 promotes T-cell activation and differentiation into the Th2 subtype, while both IL-4 and IL-13 promote IgE production in B cells and mucus production in the airways [1415]. IFN-γ affects Th1/Th2 differentiation, the suppression of Th2 cytokines released from activated T cells, and the inhibition of effector cell recruitment to the site of inflammation. IFN-γ is the principal Th1 effector cytokine and has a crucial role in the resolution of many allergy-associated disorders.
Tregs, a unique subpopulation of CD4+ CD25+ Foxp3+ T cells, prevent immune responses and play a crucial role in the maintenance of self-tolerance. Tregs crucially protect the host from autoimmunity by inhibiting the response of self-reactive T cells and are essential for preventing immunopathology in immune responses directed against foreign antigens [13]. Mouse CD4+ CD25+ Foxp3+ T cells have been shown to suppress the in vitro differentiation of Th2 cells from naive CD4+ T cells, but required preactivation to inhibit cytokine production from differentiated Th2 cells [16]. While Tregs normally prevent IgE responses [17], the depletion of CD4+ CD25+ Foxp3+ T cells was found to reduce airway inflammation in a murine model of allergic sensitization [18]. In another report, the transfer of CD4+ CD25+ Foxp3+ T cells seemed to have no effect on inflammation or airway hyperresponsiveness [19]. These studies suggest that the role of Tregs in murine models of allergic rhinitis is controversial.
Epigenetic regulation, classified as DNA methylation and chromatin modifications, is critical for generating cellular diversity and maintaining distinct gene expression profiles. Histone acetylation, one type of chromatin modification, is regulated by histone acetyltransferases and HDACs [78]. HDAC inhibitors modulate transcriptional activity by interfering with the removal of acetyl groups from histones, thereby altering gene expression. HDAC inhibitors were recently found to alter the expression of several genes related to inflammatory processes, including inducible nitric oxide synthase, macrophage inflammatory protein-2, IL-8, tumor necrosis factor-α, IL-1β, IL-6, and IFN-γ [20]. Recent studies in murine models of allergic airway disease have demonstrated that the HDAC inhibitors TSA has potent anti-inflammatory effects [21]. In these studies, TSA treatment inhibited Th2 responses, reducing IL-4, IL-5, and IgE, and attenuated airway inflammation [22]. This is likely due to the inhibition of T-cell recruitment and Th2 cytokine production in acute models of allergic airway disease. Further recent studies have shown that TSA promotes FoxP3+ regulatory T cell [23]. Taken together, these findings suggest that inhibition of HDAC may reduce allergic rhinitis. In the present study, we have examined the effect of HDAC inhibition by TSA on OVA-induced nasal inflammation via Treg cells in a murine model of allergic rhinitis.
Our data has demonstrated that TSA inhibits allergic nasal symptoms, eosinophil infiltration, and production of OVA-specific IgE, Th2-type cytokines and Treg cytokines in a murine model of allergic rhinitis. These findings imply that TSA has a potential anti-inflammatory role by inhibiting OVA-specific allergic responses in nasal mucosa. Further studies are needed to better evaluate the role of TSA on mediating inflammation and to determine potential HDAC inhibition by TSA
In conclusion, TSA suppresses allergic nasal symptoms, allergic cytokines and Treg cytokines in a murine model of allergic rhinitis. TSA was shown to decrease eosinophil migration into the nasal mucosa and inhibit serum IgE and nasal IL-4 and IL-5 levels. Our study provides evidence of the antiallergic efficacy of TSA in an in vivo model of allergic rhinitis. Our findings suggest that the use of TSA results in a beneficial pharmacological effect on the induction of Tregs in a mouse model of allergic rhinitis. Thus, TSA could be considered a potential therapeutic agent in treating allergic rhinitis in humans.
ACKNOWLEDGMENTS
This study was supported by a grant from the Basic Science Research Program through the National Research Foundation of Korea (NRF), which is funded by the Ministry of Education, Science, and Technology (2010-0010279) and Korea University Grant.
References
1. Hellings PW, Ceuppens JL. Mouse models of global airway allergy: what have we learned and what should we do next? Allergy. 2004; 9. 59(9):914–919. PMID: 15291897.


2. Jeffery PK, Haahtela T. Allergic rhinitis and asthma: inflammation in a one-airway condition. BMC Pulm Med. 2006; 11. 6(Suppl 1):S5. PMID: 17140423.


3. Melvin TA, Ramanathan M Jr. Role of innate immunity in the pathogenesis of allergic rhinitis. Curr Opin Otolaryngol Head Neck Surg. 2012; 6. 20(3):194–198. PMID: 22569404.


4. Yssel H, Groux H. Characterization of T cell subpopulations involved in the pathogenesis of asthma and allergic diseases. Int Arch Allergy Immunol. 2000; 1. 121(1):10–18. PMID: 10686504.


5. Possa SS, Leick EA, Prado CM, Martins MA, Tiberio IF. Eosinophilic inflammation in allergic asthma. Front Pharmacol. 2013; 4. 4:46. PMID: 23616768.


6. Varga EM, Jacobson MR, Till SJ, Masuyama K, O'Brien F, Rak S, et al. Cellular infiltration and cytokine mRNA expression in perennial allergic rhinitis. Allergy. 1999; 4. 54(4):338–345. PMID: 10371092.


7. Bhavsar P, Ahmad T, Adcock IM. The role of histone deacetylases in asthma and allergic diseases. J Allergy Clin Immunol. 2008; 3. 121(3):580–584. PMID: 18234319.


8. Barnes PJ, Adcock IM, Ito K. Histone acetylation and deacetylation: importance in inflammatory lung diseases. Eur Respir J. 2005; 3. 25(3):552–563. PMID: 15738302.


9. Cho JS, Moon YM, Park IH, Um JY, Moon JH, Park SJ, et al. Epigenetic regulation of myofibroblast differentiation and extracellular matrix production in nasal polyp-derived fibroblasts. Clin Exp Allergy. 2012; 6. 42(6):872–882. PMID: 22239687.


10. Cho JS, Moon YM, Park IH, Um JY, Kang JH, Kim TH, et al. Effects of histone deacetylase inhibitor on extracellular matrix production in human nasal polyp organ cultures. Am J Rhinol Allergy. 2013; 1. 27(1):18–23. PMID: 23406592.


11. Akdis M, Verhagen J, Taylor A, Karamloo F, Karagiannidis C, Crameri R, et al. Immune responses in healthy and allergic individuals are characterized by a fine balance between allergen-specific T regulatory 1 and T helper 2 cells. J Exp Med. 2004; 6. 199(11):1567–1575. PMID: 15173208.


12. Meiler F, Zumkehr J, Klunker S, Ruckert B, Akdis CA, Akdis M. In vivo switch to IL-10-secreting T regulatory cells in high dose allergen exposure. J Exp Med. 2008; 11. 205(12):2887–2898. PMID: 19001136.


13. Sakaguchi S, Wing K, Yamaguchi T. Dynamics of peripheral tolerance and immune regulation mediated by Treg. Eur J Immunol. 2009; 9. 39(9):2331–2336. PMID: 19662638.


14. Shim JJ, Dabbagh K, Ueki IF, Dao-Pick T, Burgel PR, Takeyama K, et al. IL-13 induces mucin production by stimulating epidermal growth factor receptors and by activating neutrophils. Am J Physiol Lung Cell Mol Physiol. 2001; 1. 280(1):L134–L140. PMID: 11133503.


15. Frew AJ. The immunology of respiratory allergies. Toxicol Lett. 1996; 8. 86(2-3):65–72. PMID: 8711778.


16. Stassen M, Jonuleit H, Muller C, Klein M, Richter C, Bopp T, et al. Differential regulatory capacity of CD25+ T regulatory cells and preactivated CD25+ T regulatory cells on development, functional activation, and proliferation of Th2 cells. J Immunol. 2004; 7. 173(1):267–274. PMID: 15210784.
17. Curotto de Lafaille MA, Muriglan S, Sunshine MJ, Lei Y, Kutchukhidze N, Furtado GC, et al. Hyper immunoglobulin E response in mice with monoclonal populations of B and T lymphocytes. J Exp Med. 2001; 11. 194(9):1349–1359. PMID: 11696599.
18. Suto A, Nakajima H, Kagami SI, Suzuki K, Saito Y, Iwamoto I. Role of CD4(+) CD25(+) regulatory T cells in T helper 2 cell-mediated allergic inflammation in the airways. Am J Respir Crit Care Med. 2001; 8. 164(4):680–687. PMID: 11520737.
19. Jaffar Z, Sivakuru T, Roberts K. CD4+CD25+ T cells regulate airway eosinophilic inflammation by modulating the Th2 cell phenotype. J Immunol. 2004; 3. 172(6):3842–3849. PMID: 15004191.
20. Leoni F, Zaliani A, Bertolini G, Porro G, Pagani P, Pozzi P, et al. The antitumor histone deacetylase inhibitor suberoylanilide hydroxamic acid exhibits antiinflammatory properties via suppression of cytokines. Proc Natl Acad Sci U S A. 2002; 3. 99(5):2995–3000. PMID: 11867742.


21. Xu WS, Parmigiani RB, Marks PA. Histone deacetylase inhibitors: molecular mechanisms of action. Oncogene. 2007; 8. 26(37):5541–5552. PMID: 17694093.


22. Choi JH, Oh SW, Kang MS, Kwon HJ, Oh GT, Kim DY. Trichostatin A attenuates airway inflammation in mouse asthma model. Clin Exp Allergy. 2005; 1. 35(1):89–96. PMID: 15649272.


23. Guo X, Jie Y, Ren D, Zeng H, Zhang Y, He Y, et al. Histone deacetylase inhibitors promote mice corneal allograft survival through alteration of CD4+ effector T cells and induction of Foxp3+ regulatory T cells. Cell Immunol. 2012; May-Jun. 277(1-2):8–13. PMID: 22776176.
Fig. 1
Schematic representation of the murine model of allergic rhinitis and treatment protocol. BALB/c mice were sensitized with ovalbumin (OVA) and 1 mg of aluminum hydroxide (Alum) on days 0, 7, and 14. All groups except control received intranasal OVA from 21 days to 24 days. In addition to sensitization and challenge, TSA+OVA mice were injected with TSA (1 mg/kg/day). TSA, trichostatin A.
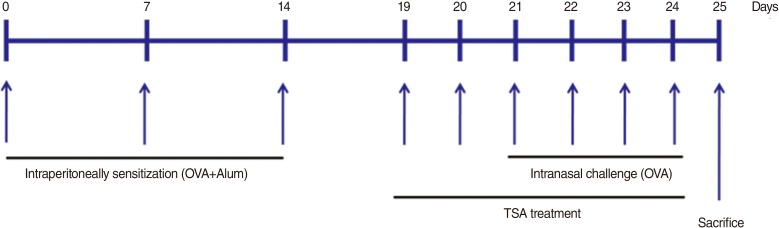
Fig. 2
Nasal symptom scores. (A) Rubbing score. (B) Sneezing score. OVA, ovalbumin; TSA, trichostatin A. *P<0.05 and **P<0.01 compared to control. †P<0.05 compared to OVA.
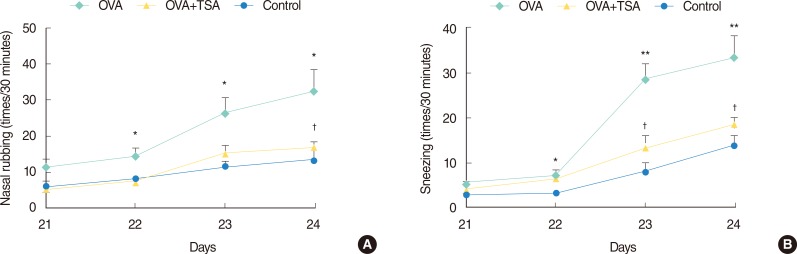
Fig. 3
Serum OVA-specific IgE levels. Serum OVA-specific IgE levels were measured by enzyme-linked immunosorbent assay. Increased level of serum OVA-specific IgE in OVA group was decreased in OVA+TSA group. OVA, ovalbumin; TSA, trichostatin A. *P<0.05 compared with control. †P<0.05 compared with OVA.
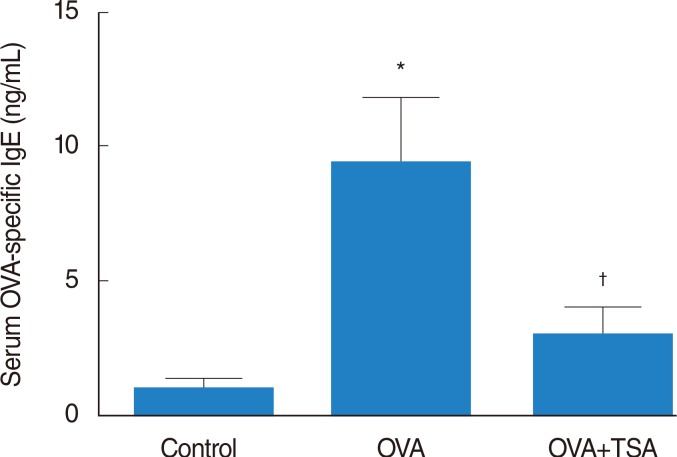
Fig. 4
Infiltration of eosinophils in the nasal mucosa. (A) Chromotrophe 2R staining of the nasal mucosa in each group. Level of eosinophil infiltration (red color) markedly decreased in OVA+TSA group compared with OVA group. (B) Eosinophil counts in the nasal mucosa. Eosinophil count was significantly reduced in OVA+TSA group compared with OVA group (×400). OVA, ovalbumin; TSA, trichostatin A. *P<0.05 compared to control. †P<0.05 compared to OVA.
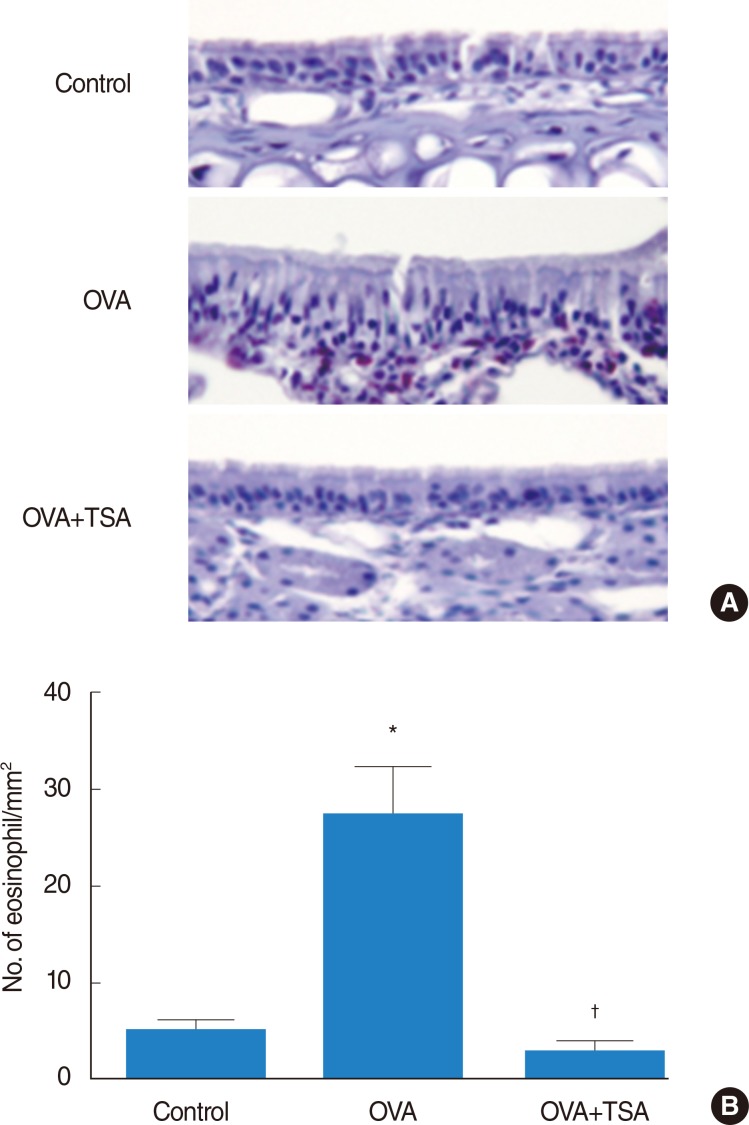
Fig. 5
IFN-γ, IL-4, and IL-5 expression levels. Messenger RNA levels of IFN-γ (A), IL-4 (C), and IL-5 (E) were measured by quantitative reverse transcription-polymerase chain reaction. Protein levels of IFN-γ (B), IL-4 (D), and IL-5 (F) were measured by enzyme-linked immunosorbent assay. Levels of IL-4 and IL-5 were inhibited in OVA+TSA group compared with OVA group. However, IFN-γ level did not increase in OVA group. IFN-γ, interferon-gamma; IL, interleukin; OVA, ovalbumin; TSA, trichostatin A. *P<0.05 compared to controls. †P<0.05 compared to OVA.
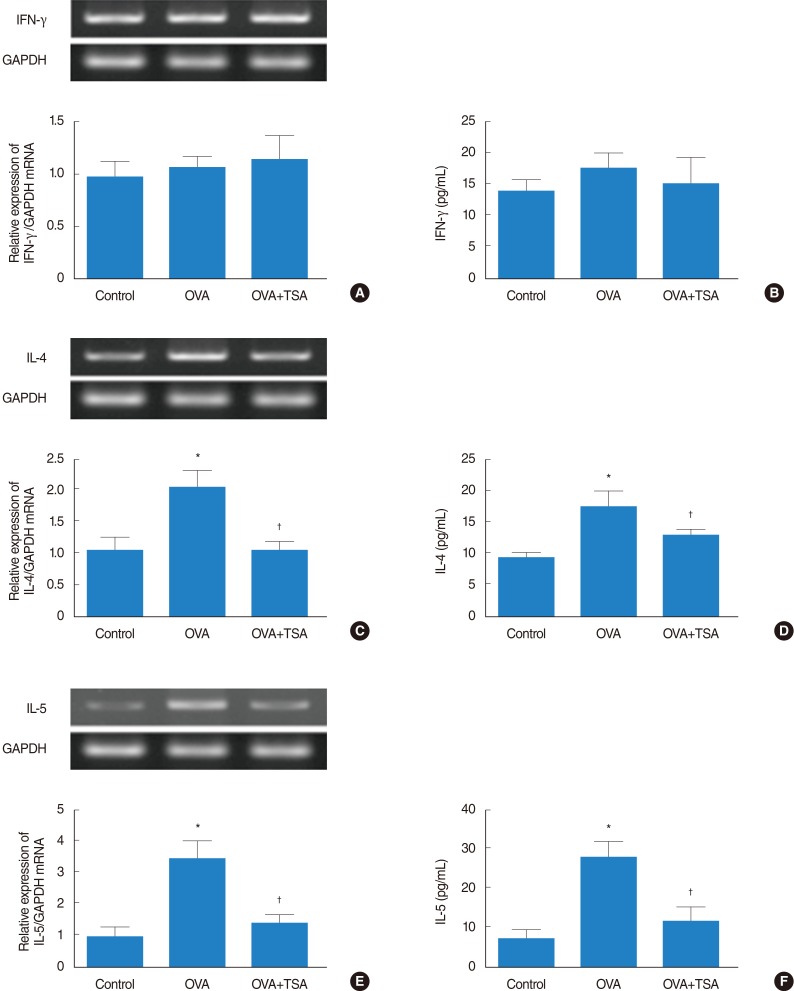
Fig. 6
Activation of regulatory T cells. (A) mRNA level of Foxp3 was analyzed by quantitative reverse transcription-polymerase chain reaction. Protein levels of IL-10 (B) and TGF-β (C) were significantly increased in OVA+TSA group, as measured by enzyme-linked immunosorbent assay. OVA, ovalbumin; TSA, trichostatin A; IL, interleukin; TGF-β, transforming growth factor-beta. *P<0.05 and **P<0.01 compared to controls.
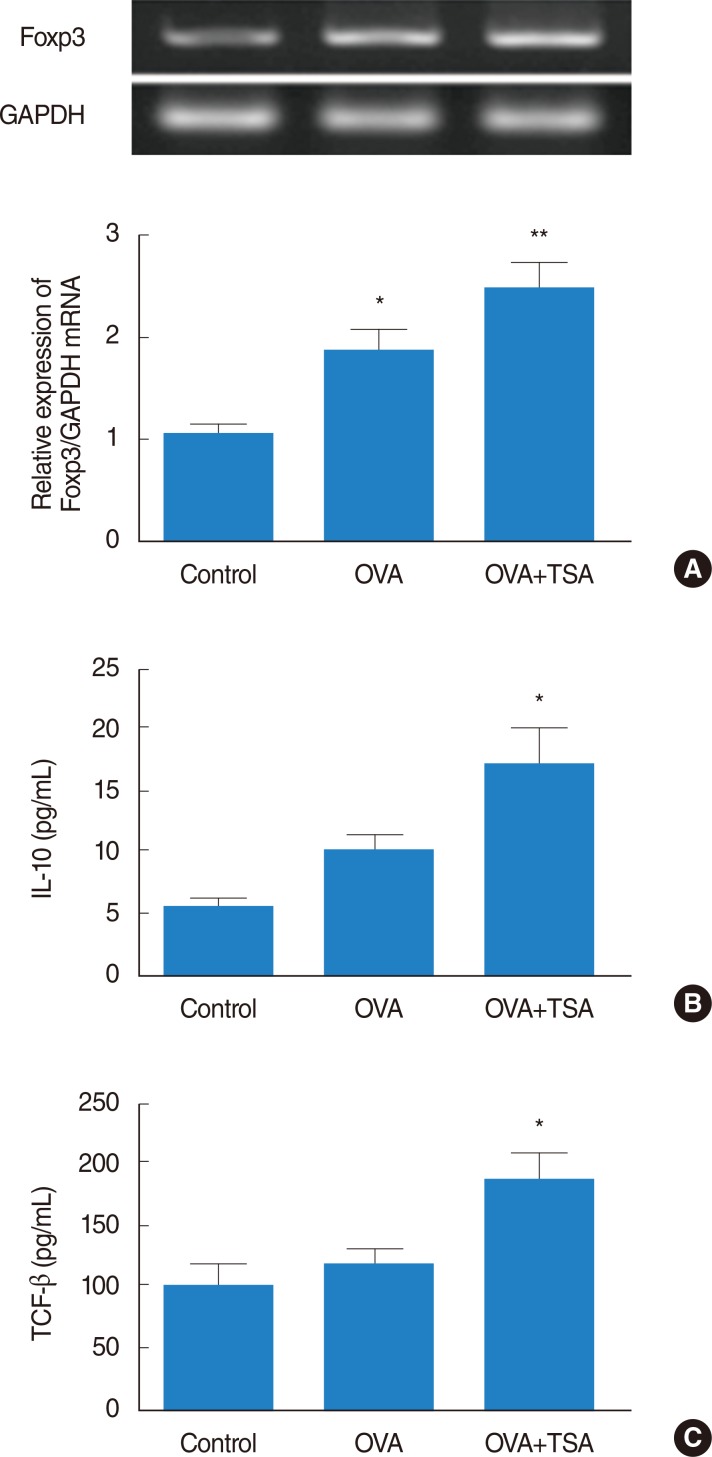