Abstract
Objectives
From our previous study about the weak expressions of potassium-chloride (KCC2) and sodium-potassium-2 chloride (NKCC1) co-transporters in the lateral superior olive (LSO) in circling mice, we hypothesized that partially damaged cochlea of circling mice might be a cause of the weak expressions of KCC2 or NKCC1. To test this possibility, we reproduced the altered expressions of KCC2 and NKCC1 in the LSO of rats, whose cochleae were partially destroyed with kanamycin.
Methods
Rat pups were treated with kanamycin from postnatal (P)3 to P8 (700 mg/kg, subcutaneous injection, twice a day) and sacrificed for immunohistochemical analysis, scanning electron microscope (SEM) and auditory brain stem response.
Results
The SEM study revealed partially missing hair cells in P9 rats treated with kanamycin, and the hearing threshold was elevated to 63.8±2.5 dB SPL (4 ears) at P16. Both KCC2 and NKCC1 immunoreactivities were more prominent in control rats on P16. On 9 paired slices, the mean densities of NKCC1 immunoreactivities were 118.0±1.0 (control) and 112.2±1.2 (kanamycin treated), whereas those of KCC2 were 115.7±1.5 (control) and 112.0±0.8 (kanamycin treated).
The lateral superior olive (LSO) is a binaural auditory nucleus in the auditory brainstem and receives gamma-aminobutyric acid (GABA)/glycinergic inhibitory input from the contralateral ear, via the medial nucleus of trapezoid body (MNTB) [1]. In rats, the MNTB-LSO synapses undergo a depolarizing-hyperpolarizing shift in the first postnatal week [1, 2], similar to other immature neuronal systems, such as the hippocampus [3], hypothalamus [4], neocortex [5], and spinal and cranial motor neurons [6, 7]. This depolarizing-hyperpolarizing shift depends on the changes of Cl- reversal potentials relative to resting membrane potentials, and is mainly caused by temporal changes in the internal concentration of Cl- ([Cl-]in). Two cation-chloride co-transporters, potassium-chloride (KCC2) and sodium-potassium-2 chloride (NKCC1), are major candidates for the changes of [Cl-]in in developing neurons [8, 9]. Immature neurons accumulate Clthrough NKCC1, resulting in a depolarizing action of GABA [10], whereas later in development, gradual up-regulation of KCC2 results in Cl- extrusion and a hyperpolarizing action of GABA [9].
We previously reported altered function and expression of
KCC2 and NKCC1 in the circling mouse [11], a recently developed animal model for human non-syndromic hearing loss (DFNB6), inherited in an autosomal recessive mode with 100% penetrance [12-14]. In that report, we demonstrated the less prominent immunoreactivities of KCC2 and NKCC1 on postnatal (P)16, and weak or absent responses of Cl- reversal potential to either bumetanide (a known NKCC1 blocker) or furosemide (a known KCC2 blocker), in P9-P12 homozygous (cir/cir) mice (circling mice), while sequential treatment of bumetanide and furosemide shifted the chloride reversal potential to hyperpolarization followed by depolarization, in P9-P12 heterozygous (+/cir) mice [11]. As spontaneous cochlear degeneration occurs relatively early in circling mice, we hypothesized that altered expression and function of KCC2 and NKCC1 might be related to cochlear degeneration. However, we could not argue convincingly that they were cochlear-dependent, because circling mice are genetically abnormal and the hair cells are relatively intact at the time of investigation (P9 to P12) [12]. To prove the cochlear dependency of KCC2 or NKCC1 expression in circling mice, we had to reproduce the altered expression of NKCC1 and KCC2 in genetically normal animals, whose cochleae were partially, not totally destroyed.
Aminoglycosides have been reported to be not as strongly effective during the first postnatal week [15-18] and we already demonstrated that large doses of kanamycin produced partial cochlear damage in developing rats [19]. Therefore, in this study, we reproduced the altered expression of KCC2 and NKCC1 using rat pups treated with high dose kanamycin from P3 to P8. Consequently, we observed the alterations of KCC2 and NKCC1 expression in kanamycin-treated rat pups, which were similar to those observed in circling mice. The detailed results and meaning are discussed.
Pregnant female Sprague-Dawley rats were purchased from a domestic company (Samtako BioKorea, Osan, Korea) and their pups were used. The pups were treated with kanamycin (700 mg/kg, subcutaneous injection, twice a day) from P3 to P8 and were evaluated after P9. For the sham operation, control pups were injected with distilled water from P3 to P8. The animals were maintained in the Animal Care Facility of Dankook University. The Dankook University Institutional Animal Care and Use Committee approved this study.
Immunohistochemical staining was performed on P16, as described previously [20]. Briefly, after washing with PBS, treatment with 1% H2O2 was followed by incubation for 48 hours at 4℃ with either rabbit polyclonal anti-KCC2 (07-432; Millipore, Temecula, CA, USA) or rabbit polyclonal anti-NKCC1 (AB59791; Abcam, Cambridge, UK) in blocking buffer (2% bovine serum albumin, 0.3% Triton X-100, 1% horse serum, and 0.1 M PBS). The dilution ratios of KCC2 and NKCC1 were 1:70,000 and 1:2,000, respectively. The sections were evaluated using an Olympus BX51 microscope and photographs of the sections were obtained with a microscope digital camera system (DP50; Olympus, Tokyo, Japan). The NIH image program (Scion Image) was used to determine the staining densities. Comparisons were made using the Mann-Whitney U-test (SPSS ver. 12.0; SPSS Inc., Chicago, IL, USA), and a P<0.05 was considered statistically significant.
For the scanning electron microscope (SEM) study, intracardiac perfusions were performed at P9 with 4% paraformaldehyde, while the animals were under deep anesthesia with zoletil (Virbac, Carros, France). After perfusion, animals were decapitated and the cochleae were removed immediately. The bony capsule was removed and the lateral wall tissues, as well as the membranous structures, were separated. The dissected specimens were rinsed with 0.1 M PBS and fixed in 1% osmium tetroxide. After that, the specimens were gently rinsed with 0.1 M PBS and dehydrated in graded series of ethanol. After immersing in absolute ethanol for 1 hour, the specimens were dried in a critical point dryer (HCP-2; Hitachi, Tokyo, Japan). The dried specimens were attached to aluminum stubs and coated with platinum-palladium, using the E-1030 ion sputter (Hitachi). The surfaces of the organ of Corti were examined with S-4300 SEM (Hitachi). Two different cochleae were evaluated with the SEM. The percent distance from the apex was 12.6-22.3% (17.5±4.3%) for the apical turn, 20.7-54.2% (47.4±5.5%) for the middle turn and 66.0-85.6% (75.8±13.8%) for the basal turn. The number of hair cells was counted from two different sites for each turn, except for the basal turn. The location for evaluation was almost identical in each SEM observation.
For hearing assessment, the threshold of auditory brainstem response (ABR) was measured in 4 ears (2 different animals) at P16. The rats were anaesthetized and placed in a soundproof booth. Following anesthesia, needle electrodes were placed subcutaneously at the vertex (active electrode) and beneath the pinna (reference and ground electrode, respectively). Click stimulus was presented to the animals. ABRs were measured with the BiosigRP Stimulate/Record System ver. 4.4.1 (Tucker-Davis Technologies, Alachua, FL, USA).
Rats were treated with high-dose kanamycin from P3 to P8 and the cochlear damage was evaluated with SEM at P9. The SEM study revealed partially missing hair cells in the apical to middle turn (n=2) (Fig. 1). The SEM study revealed that some outer hair cells comprising 3 outer rows were missing intermittently from apical to middle turn. The percentage ratio of missing hair cells were 7.5±5.5% (n=2) in the apical turn, 3.5±6.9% (n=2) in the middle turn. To further investigate the effect of kanamycin, we also tested ABR at P16. While the hearing threshold was near 10-20 dB SPL in the control animals, it was elevated to 60 dB SPL after kanamycin treatment (Fig. 2). The average hearing threshold was 63.8±2.5 dB SPL in four ears of kanamycin-treated rats.
As NKCC1 develops late (around P12) compared to KCC2 in rats [21], we investigated the immunohistochemistry of KCC2 and NKCC1 in LSO neurons of kanamycin-treated rats at P16. On P16, KCC2 immunoreactivities were observed in the LSO of both control and kanamycin-treated rats (Fig. 3A, B). In the LSO, KCC2 immunoreactivities were more prominent in control rats. In 9 paired slices from 6 rats (3 control and 3 kanamycin treated), the mean densities of KCC2 immunoreactivities on P16 were 115.7±1.5 mm-2 (control) and 112.0±0.8 mm-2 (kanamycin-treated). The difference was statistically significant (P=0.015). We also found more prominent immunoreactivities of NKCC1 in control rats than in kanamycin-treated rats (Fig. 4A, B). In addition, the LSO neurons of control rats were more darkly stained. In 9 paired slices from 6 rats (3 control and 3 kanamycin treated), the mean densitites of NKCCl immunoreactivities on P16 were 118.0±1.0 mm-2 (control) and 112.2±1.2 mm-2 (kanamycin-treated). The two values were significantly different (P=0.004).
Experimental evidence suggested that the susceptible period to kanamycin starts around the time of onset of auditory function. In rats, the susceptible period starts around P10 [16, 18]. Therefore, it has been generally accepted that systemic administration of kanamycin before P10 does not induce any detectable damages in cochlear hair cells in rats [17]. However, we demonstrated partial cochlear hair cell damages in rats treated with kanamycin from P3 to P8 [19]. The only methodological difference lies in the dosage of kanamycin. In the previous study, 400 mg/kg (daily) has been used [17]; however, in this study, we used 700 mg/kg (subcutaneous injection, twice a day).
The only reason for us to use high dose kanamycin in the non-susceptible period (P3 to P8) was to induce partial hair cell damages. We previously reported weak immunoreactivities of KCC2 and NKCC1 in circling mice [11], in which hair cells are relatively intact and only minor morphologic changes were observed [12] when the expressions of KCC2 and NKCC1 were evaluated. Therefore, to prove the cochlear dependency of KCC2 and NKCC1 expressions, we had to reproduce similar immunohistochemical data in genetically normal animals of similar ages, whose cochleae were only partially destroyed.
In this study, although both KCC2 and NKCC1 immunodensity differences between control and kanamycin-treated rats were not great, they were statistically different. As younger rats are less-susceptible to kanamycin than older ones, the period that KCC2 is effectively affected by kanamycin might be shorter than the whole treatment period (P3 to P8). This might lead to the partial destruction of cochleae and the small difference of KCC2 immunodensity in LSO between control and kanamycin-treated rats. In developing rats, KCC2 develops earlier than NKCC1 [21]. KCC2 mRNA was detected in LSO neurons during both depolarizing and hyperpolarizing periods, whereas NKCC1 mRNA was detected only in the hyperpolarizing period [21]. In our previous study, we demonstrated in circling mice that the immunodensity difference of KCC2 was relatively greater than that of NKCC1. In 15 paired slices, KCC2 immunoreactivities were 92.1±2.7 mm-2 (control) and 82.2±4.1 mm-2 (circling mice), whereas NKCC1 immunoreactivities were 118.72±3.27 mm-2 (control) and 109.06±1.08 mm-2 (circling mice) on P16 [11]. We do not know the reason, but it seems that the early developing KCC2 is affected more than NKCC1 by cochlear destruction. This might partly explain the small but significant difference of NKCC expression between control and kanamycin-treated rats. In our previous study using kanamycin-treated rats [19], we did not observe any neurotransmitter changes in MNTB-LSO synapses when the dose of kanamycin was reduced to 400 mg/kg/day, a dose which has been reported to produce no changes in the brainstem auditory potential [18]. Although we could not rule out the possibility that kanamycin itself affected KCC2 or NKCC1 expression, this seems unlikely at present.
Cochlear activity has been suggested to play a crucial role in the function or expression of KCC2, even before hearing onset. Bilateral cochlear ablation before the onset of hearing abolished the expression of KCC2 mRNA in the LSO of P14 to P15 rats; LSO neurons isolated from strychnine-reared rats at P14 to P16 had a relatively high [Cl-]in compared to neurons from control rats [22]. In the inferior colliculus of gerbils, bilateral cochlear ablations before the onset of hearing also reduced the ability of neurons to transport Cl-, although the levels of NKCC1 and KCC2 mRNA were unchanged [23]. These reports indicate the involvement of afferent synaptic activity in the development of KCC2 and support our conclusion.
The developing GABA/glycinergic MNTB-LSO synapses of circling mice have two characteristic features. One is the enhanced glutamatergic transmission and the other is the underdevelopment of glycine receptors of LSO neurons [20]. We recently reported the underdevelopment of glycine receptors and enhanced glutamatergic transmission in kanamycin-treated rats [19]. The sustained glutamatergic co-transmission in kanamycin-treated rats might provide a favorable condition for the KCC2 underdevelopment. The enhanced glutamatergic transmission with underdevelopment of glycine receptors will raise the excitability of MNTB-LSO synapses and will lead to elevation of intracellular Ca2+ concentration. Indeed, in vitro, in dissociated cultures of mature hippocampal neurons, it has been reported that Ca2+ influx through NMDA receptors is a cause of down-regulation of KCC2 [24]. Thus, it might be suggested that the enhanced glutamatergic co-transmission, induced by partial cochlear damage, may play a key role in the expression of KCC2 or NKCC1 in circling mice and/or kanamycin-treated rats.
In this study, we demonstrated the less prominent immunoreactivities of KCC2 and NKCC1 in kanamycin-treated rats than in control rats. As those phenomena were observed under partial damage of cochlear hair cells, it might be suggested that the expression of KCC2 or NKCC1 in circling mice is also cochlear-dependent. As the responses in kanamycin-treated rats were quite similar to those observed in circling mice, circling mice and kanamycin-treated rats might share common mechanisms related to KCC2 or NKCC1 expression. As kanamycin treatment is relatively non-invasive and the kanamycin-treated rat model is easy to reproduce, it will be of help to elucidate the regulating factors of KCC2 or NKCC1 expression in future studies.
ACKNOWLEDGMENTS
The present research was conducted by the research fund of Dankook University in 2012. We deeply thank Dr. Dhiraj Maskey for his technical assistance.
References
1. Kandler K, Friauf E. Development of glycinergic and glutamatergic synaptic transmission in the auditory brainstem of perinatal rats. J Neurosci. 1995; 10. 15(10):6890–6904. PMID: 7472446.


2. Lohrke S, Srinivasan G, Oberhofer M, Doncheva E, Friauf E. Shift from depolarizing to hyperpolarizing glycine action occurs at different perinatal ages in superior olivary complex nuclei. Eur J Neurosci. 2005; 12. 22(11):2708–2722. PMID: 16324105.
3. Cherubini E, Rovira C, Gaiarsa JL, Corradetti R, Ben Ari Y. GABA mediated excitation in immature rat CA3 hippocampal neurons. Int J Dev Neurosci. 1990; 8(4):481–490. PMID: 2174638.


4. Chen G, Trombley PQ, van den Pol AN. Excitatory actions of GABA in developing rat hypothalamic neurones. J Physiol. 1996; 7. 494(Pt 2):451–464. PMID: 8842004.


5. Luhmann HJ, Prince DA. Postnatal maturation of the GABAergic system in rat neocortex. J Neurophysiol. 1991; 2. 65(2):247–263. PMID: 1673153.


6. Singer JH, Talley EM, Bayliss DA, Berger AJ. Development of glycinergic synaptic transmission to rat brain stem motoneurons. J Neurophysiol. 1998; 11. 80(5):2608–2620. PMID: 9819267.


7. Wu WL, Ziskind-Conhaim L, Sweet MA. Early development of glycine- and GABA-mediated synapses in rat spinal cord. J Neurosci. 1992; 10. 12(10):3935–3945. PMID: 1403091.


8. Plotkin MD, Snyder EY, Hebert SC, Delpire E. Expression of the Na-K-2Cl cotransporter is developmentally regulated in postnatal rat brains: a possible mechanism underlying GABA's excitatory role in immature brain. J Neurobiol. 1997; 11. 33(6):781–795. PMID: 9369151.


9. Rivera C, Voipio J, Payne JA, Ruusuvuori E, Lahtinen H, Lamsa K, et al. The K+/Cl- co-transporter KCC2 renders GABA hyperpolarizing during neuronal maturation. Nature. 1999; 1. 397(6716):251–255. PMID: 9930699.


10. Yamada J, Okabe A, Toyoda H, Kilb W, Luhmann HJ, Fukuda A. Cl- uptake promoting depolarizing GABA actions in immature rat neocortical neurones is mediated by NKCC1. J Physiol. 2004; 6. 557(Pt 3):829–841. PMID: 15090604.
11. Pradhan J, Maskey D, Park KS, Kim MJ, Ahn SC. Decreased immunoreactivities and functions of the chloride transporters, KCC2 and NKCC1, in the lateral superior olive neurons of circling mice. Clin Exp Otorhinolaryngol. 2011; 3. 4(1):18–23. PMID: 21461058.


12. Chung WH, Kim KR, Cho YS, Cho DY, Woo JH, Ryoo ZY, et al. Cochlear pathology of the circling mouse: a new mouse model of DFNB6. Acta Otolaryngol. 2007; 3. 127(3):244–251. PMID: 17364360.


13. Lee JW, Lee EJ, Hong SH, Chung WH, Lee HT, Lee TW, et al. Circling mouse: possible animal model for deafness. Comp Med. 2001; 12. 51(6):550–554. PMID: 11924819.
14. Lee JW, Ryoo ZY, Lee EJ, Hong SH, Chung WH, Lee HT, et al. Circling mouse, a spontaneous mutant in the inner ear. Exp Anim. 2002; 4. 51(2):167–171. PMID: 12012726.


15. Carlier E, Pujol R. Supra-normal sensitivity to ototoxic antibiotic of the developing rat cochlea. Arch Otorhinolaryngol. 1980; 226(3):129–133. PMID: 7458747.


16. Marot M, Uziel A, Romand R. Ototoxicity of kanamycin in developing rats: relationship with the onset of the auditory function. Hear Res. 1980; 3. 2(2):111–113. PMID: 7364666.


17. Onejeme AU, Khan KM. Morphologic study of effects of kanamycin on the developing cochlea of the rat. Teratology. 1984; 2. 29(1):57–71. PMID: 6701807.


18. Osako S, Tokimoto T, Matsuura S. Effects of kanamycin on the auditory evoked responses during postnatal development of the hearing of the rat. Acta Otolaryngol. 1979; 88(5-6):359–368. PMID: 532611.


19. Lee JH, Pradhan J, Maskey D, Park KS, Hong SH, Suh MW, et al. Glutamate co-transmission from developing medial nucleus of the trapezoid body: lateral superior olive synapses is cochlear dependent in kanamycin-treated rats. Biochem Biophys Res Commun. 2011; 2. 405(2):162–167. PMID: 21215254.
20. Hong SH, Kim MJ, Ahn SC. Glutamatergic transmission is sustained at a later period of development of medial nucleus of the trapezoid body-lateral superior olive synapses in circling mice. J Neurosci. 2008; 11. 28(48):13003–13007. PMID: 19036993.


21. Balakrishnan V, Becker M, Lohrke S, Nothwang HG, Guresir E, Friauf E. Expression and function of chloride transporters during development of inhibitory neurotransmission in the auditory brainstem. J Neurosci. 2003; 5. 23(10):4134–4145. PMID: 12764101.


22. Shibata S, Kakazu Y, Okabe A, Fukuda A, Nabekura J. Experience-dependent changes in intracellular Cl- regulation in developing auditory neurons. Neurosci Res. 2004; 2. 48(2):211–220. PMID: 14741396.


23. Vale C, Schoorlemmer J, Sanes DH. Deafness disrupts chloride transporter function and inhibitory synaptic transmission. J Neurosci. 2003; 8. 23(20):7516–7524. PMID: 12930790.


24. Kitamura A, Ishibashi H, Watanabe M, Takatsuru Y, Brodwick M, Nabekura J. Sustained depolarizing shift of the GABA reversal potential by glutamate receptor activation in hippocampal neurons. Neurosci Res. 2008; 12. 62(4):270–277. PMID: 18840481.


Fig. 1
Scanning electron microscopic image of the cochlea on postnatal (P)9, after 6 days of kanamycin treatment (P3 to P8). In the picture at the top, box areas indicate the areas where the lower photographs (A-C) were taken. Missing hair cells were identified in several areas of the apical (A) and middle (B) turn. In contrast, the hair cells in basal (C) turn were relatively preserved. In the top picture, '1.5 mm' indicates the length between the wedge shape marks at the lateral margins of the picture.

Fig. 2
The threshold change of auditory brainstem response (ABR), after 6 days of kanamycin treatment (postnatal 3 to postnatal 8). According to waves III and V of ABR, the hearing threshold was near 10-20 dB SPL in the control (A) and near 60 dB SPL in the kanamycin-treated rat (B).

Fig. 3
The localization of potassium-chloride (KCC2) immunoreactivity in the lateral superior olive (LSO) of control (A, C) and kanamycin-treated rat (B, D). At postnatal 16, KCC2 immunoreactivities were observed in LSO of both groups (A, B). The selected areas (dashed-line box) in A and B are magnified in C and D, respectively. The arrows indicate that KCC2 immunoreactivity was confined to the cell membrane (C, D). A&B scale bar=100 µm; C&D=50 µm.
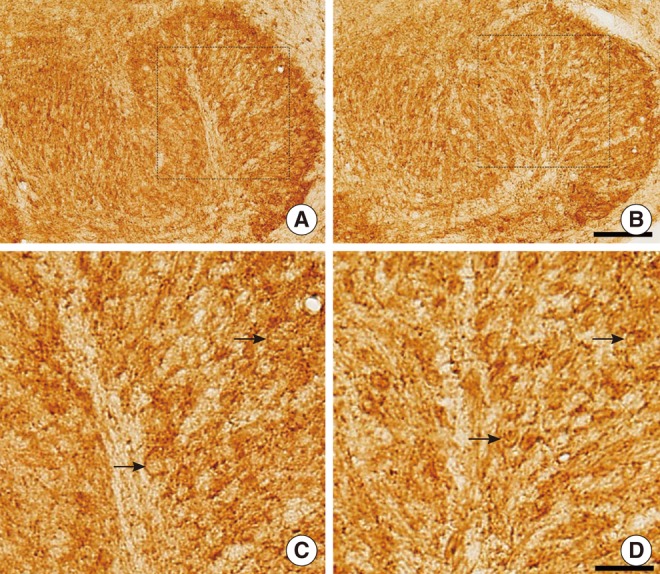
Fig. 4
The localization of sodium-potassium-2 chloride (NKCC1) immunoreactivity in the lateral superior olive (LSO) of control (A, C) and kanamycin-treated rat (B, D). At postnatal 16, NKCC1 immunoreactivities were observed in LSO of both groups (A, B). The selected areas (dashed-line box) in A and B are magnified in C and D, respectively. A&B scale bar=100 µm; C&D=50 µm.
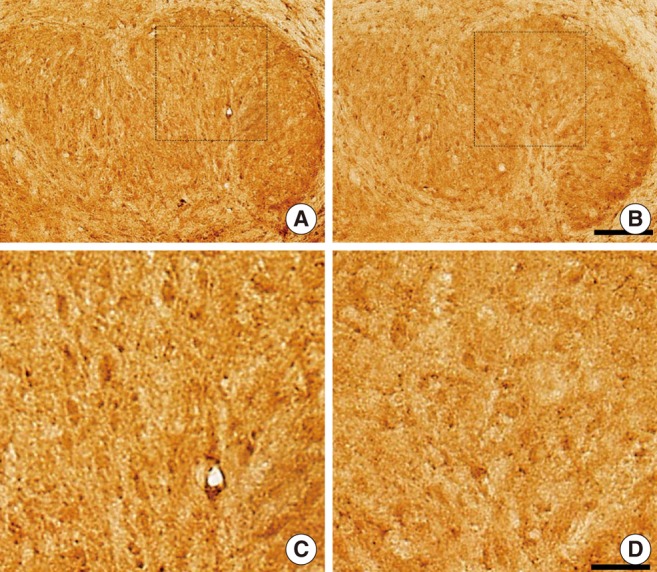