Abstract
Objectives
In this study, we evaluated the efficacy of nebulized bovine pulmonary surfactant on experimentally induced otitis media with effusion (OME) in guinea pigs.
Methods
Twenty guinea pigs were divided into three groups. Four untreated animals served as normal controls. Experimental OME was established in both ears of the remaining 16 animals by a transbullar injection of 10 µL of Pseudomonas aeruginosa lipopolysaccharide in saline. Thereafter, the guinea pigs received nebulized phosphate buffered saline (n=8) or nebulized bovine pulmonary surfactant (n=8). Nebulization was given daily for 7 days. On day 8, all the animals' passive opening pressure (POP) of the Eustachian tube was measured and histopathological observations of the bulla were made by light microscopy.
Otitis media with effusion (OME) has a multifactorial pathogenesis. OME can occur during the resolution of acute otitis media once the acute inflammation has resolved. The classic explanation proposes that dysfunction of the Eustachian tube is the necessary precursor for OME. The dysfunction can be caused a number of circumstances, including anatomic blockage, inflammation secondary to upper respiratory tract infection, allergies and trauma. Once the acute inflammation and bacterial infection have resolved, failure of the middle ear's clearance mechanism allows the middle ear effusion to persist. Dysfunction of the Eustachian tube has long been suggested to be a predisposing factor for the failure of the middle ear's clearance mechanism (1).
The presence of the surfactant in the Eustachian tube and middle ear effusions in humans has been confirmed after the possible existence of surfactants in the function of the Eustachian tube was suggested (2-6). Exogenous natural (7-9) and synthetic (10-12) surfactant preparations can reduce the passive opening pressure (POP), which is the pressure required to force the Eustachian tube open. Over 90% of the known surfactants are phospholipids whose chemical structure possesses both a hydrophilic and hydrophobic character in different regions of the compounds. Of these phospholipids, dipalmitoylphospatidylchloine comprises 50% of the relevant pulmonary surfactants, with the protein surfactants comprising the remaining 10%. In addition to their surface tension-lowering activity, pulmonary surfactants also display host defense capacities. Surfactant associated protein (SP), and especially SP-A and SP-D, has immune modulating properties (13-15). Intranasally-metered dose inhalation of aerosolized synthetic surfactant is effective in reducing the Eustachian tube POP (10-12), but the synthetic surfactants lack SPs. Although clinical trials have demonstrated that both synthetic surfactants and natural pulmonary surfactant preparations are effective to relieve lung disease, comparisons in animal models has suggested that the natural surfactant products may provide greater efficacy, and perhaps this is due to their protein content (16).
Two previous studies (8, 9) using animal models of induced OME have reported that nebulized surfactants improve the Eustachian tube function. While the results were encouraging, the small number of studies makes evaluating the significance of the results somewhat tenuous. As well, one of the studies suffered from a lack of a control (8), while in the other study the change of the middle ear pressure was observed by using a tympanometer with a 220 Hz probe tone instead of measuring the POPs of the Eustachian tube (9). Because the length and volume of the external ear canal and the middle ear of small animals are different from humans, the relevance of determining the maximal amplitudes by using a tympanometer with a 220 Hz probe tone is questionable (17). Moreover, the resonant frequency of the middle ear in guinea pigs and rats is higher than that in humans (18).
To better relate the results from animal models to human OME, we evaluated the efficacy of a nebulized natural bovine pulmonary surfactant in experimental OME by measuring the POP and performing light microscopy histopathologic examination of the bulla mucosa.
Twenty male guinea pigs (250-300 g) with normal eardrums and normal Preyer reflexes were used for the experiment. They were housed separately in sterile cages. The animals were divided into three groups. Four guinea pigs that were untreated served as the normal controls. The remaining 16 guinea pigs were used to establish experimental OME in both ears by the transbullar injection of 10 µL of Pseudomonas aeruginosa lipolysaccharide (LPS, 1 mg/mL; Sigma-Aldrich, St. Louis, MO, USA) in saline with using a 25-gauge needle via a postauricular approach. There were no constitutional symptoms in any animals after the transbullar injection of LPS. On the third day, the animals were anesthetized using a mixture of ketamine hydrochloride (40 mg/kg) and xylazine hydrochloride (8 mg/kg) that was administered intramuscularly. The presence of OME was confirmed in all the animals as a clouded, bulging tympanic membrane and the presence of middle ear effusion on a otomicroscopic examination. The experimental group 1 (n=8) was then nebulized with 5 mL/day of phosphate buffered saline (PBS) and the experimental group 2 (n=8) was nebulized with 240 mg of a natural bovine pulmonary surfactant/280 mL saline (Newfactant, Yuhan, Seoul, Korea). The experimental animals received nebulized bovine surfactant every 60 min for 7 successive days. The dose of surfactant solution used for nebulization each time was 5 mL/5 mg. Nebulization was performed by use of a nebulizer (NE-C29, Omron, Kyoto, Japan). Nebulization was repeated daily for 7 days in both experimental groups.
Thermal myringotomy using a 1 mm bovie tip (ITS, Seoul, Korea) at the anterior quadrant of the tympanic membrane was done. The baseline POP measurements were made by a modification of a previously described technique (2). A 6F latex Foley catheter (Lifetech, Seoul, Korea) was inserted to the external auditory canal of each ear. An air-tight seal was created in each canal by inflation of the balloon. The Foley catheter was then connected to an air infusion pump and a pressure transducer (AD Instruments, Castle Hill, Australia) by use of a three-way connector. The middle ear cavity was inflated by a constant infusion of air (20 mL/min) via a syringe connected to the infusion pump. The POP was recorded in millimeters of mercury (mm Hg). The pressure transducer was connected to a Grass Quad Model 15LT amplifier (Grass Technologies, West Warwick, RI, USA), and the pressure signals were recorded using a Power-Lab computerized data acquisition system (AD Instruments) (Fig. 1). The POPs were compared by the Mann-Whitney U-test. Statistical significance was set at P-values less than 0.05.
The temporal bone was dissected from the skull, denuded of adhering tissues and then further processed for light microscopy. The temporal bones harvested from each animal were preserved in 4% paraformaldehyde solution for 24 hr and then they were placed in RapidCal™ decalcifying solution (BBC Biochemical Co, Washington, USA) for 2 days. Each paraffin-embedded specimen was then sectioned and the sections were stained with hematoxylin and eosin.
After the thermal myringotomy for measuring the POP, the middle ear cavity was observed by otomicroscopic examination. In the experimental group 1, mucosal thickening with effusions on the epithelial surface were observed. However, no effusion and a normal gross appearance of the middle ear epithelium were observed in the surfactant nebulized group.
All the OME models produced a POP and the threshold of the POP was similar to that of the PBS nebulized group. The POPs of the normal control group were similar (18.2 mmHg), while that of the experimental group 1 animals (68.4 mmHg) was significantly greater. However, the group 2 animals who received the nebulized natural bovine pulmonary surfactant displayed POPs (25.3 mmHg) that were significantly lower than the POPs of the PBS nebulized experimental group 1 animals (P<0.05) (Figs. 2, 3). The subepithelial layer of the bullar mucosa of the experimental group 1 animals was thickened with inflammatory cells. However, the group 2 animals generally showed less edema and reduced subepithelial thickening (Fig. 4).
The present study evaluated the efficacy of nebulized natural bovine pulmonary surfactant on experimentally induced OME. In this study, we used guinea pigs instead of rats because of the high incidence of natural otitis media in rats. The high incidence of natural otitis media in rats may be related to its small auditory bulla and horizontal Eustachian tube. It is well established that humans with small middle ear cavities, a more horizontal Eustachian tube and a greater density of goblet cells are prone to otitis (19, 20). Yoon et al. (21) reported on the distribution of elastic fibers in the Eustachian tubes of rats. The tubal cartilage has a considerable content of elastic tissue in all regions. The mesh of elastic fibers of the rat Eustachian tube is most abundant at the hinge portion and the density gradually decreases in the middle portion. These finding suggest that elastic fibers may play an important role on the closure of the Eustachian tube following its opening by contraction of the tensor veli palatini muscle.
Nebulization with natural bovine pulmonary surfactant markedly reduced the POP of the guinea pigs with OME compared to that of the the guinea pigs with OME and who received nebulized PBS. Our results can be explained by the improved Eustachian tubal function by the phospholipids and an anti-inflammatory effect by the protein constituent of the natural bovine pulmonary surfactant. However, nebulized PBS failed to show any beneficial effect. In this study, we administered the natural surfactant by transnasal nebulization. Approximately 90% of the natural surfactant complex in the Eustachian tube is composed of phospholipids. The proteins that make up the remaining 10% are SP-A, SP-B, SP-C, and SP-D. SP-A and SP-D opsonize, aggregate and enhance phagocytosis of microbes by the resident macrophages (22-24), and they may also directly kill bacteria by permeabilizing their bacterial membranes (25). SP-B was recently shown to share features with antimicrobial peptides, and native SP-B isolated from human bronchioalveolar lavage fluid was demonstrated to kill clinical isolates of gram-positive and gram-negative bacteria in a dose-dependent manner (26). Inhalation delivery of an aerosolized metered dose of synthetic surfactant comprised of phospholipid and protein is effective in reducing the POPs in animals with OME or acute otitis media (10-12). The primary function of the synthetic surfactant is obviation of adhesion (12). Therefore, based on the present results, a natural surfactant would seem to be a more desirable choice when compared to the currently available synthetic surfactants.
The advantage of transnasal nebulization is the noninvasive access to the middle ear, without the necessity of performing myringotomy. Nebulization enables the delivery of a high concentration of the therapeutic agent to the topical mucosa. In one study, nebulized antibiotic was not detected in the serum in most cases after nebulization via the nose (27), which may result in a lower incidence or absence of general side effects due to the nebulized drug. In this study, none of the guinea pigs experienced visible discomfort during nebulization.
Nebulization of natural bovine pulmonary surfactant plays an important role in treating otitis media with effusion in guinea pigs. It is effective in reducing the Eustachian tube's passive opening pressure and the edematous inflamed bullar mucosa. Our results suggest that the chosen nebulized natural surfactant can be of clinical benefit for treating OME in the future.
ACKNOWLEDGEMENTS
This study was supported by a National Research Foundation (N RF) grant funded from the Korea government (MEST) through the Research Center for Resistant Cells (R13-2003-009) and by a grant (CRI09084-1) from Chonnam National University Hospital Research Institute of Clinical Medicine.
References
1. Bluestone CD. Eustachian tube function: physiology, pathophysiology, and role of allergy in pathogenesis of otitis media. J Allergy Clin Immunol. 1983; 9. 72(3):242–251. PMID: 6886258.


2. Flisberg K, Ingelstedt S, Ortegren U. The valve and "locking" mechanisms of the eustachian tube. Acta Otolaryngol Suppl. 1963; 182:57–68.


3. Birken EA, Brookler KH. Surface tension lowering substance of the canine Eustachian tube. Ann Otol Rhinol Laryngol. 1972; 4. 81(2):268–271. PMID: 5067550.


4. Rapport PN, Lim DJ, Weiss HS. Surface-active agent in Eustachian Tube Function. Arch Otolaryngol. 1975; 5. 101(5):305–311. PMID: 1131089.


5. Maves MD, Patil GS, Lim DJ. Surface-active substances of the guinea pig tubotympanum: a chemical and physical analysis. Otolaryngol Head Neck Surg. 1981; Mar–Apr. 89(2):307–316. PMID: 6787533.


6. Grace A, Kwok P, Hawke M. Surfactant in middle ear effusions. Otolaryngol Head Neck Surg. 1987; 4. 96(4):336–340. PMID: 3108821.


7. White P, Hermansson A, Svinhufvud M. Surfactant and isoprenaline effect on eustachian tube opening in rats with acute otitis media. Am J Otolaryngol. 1990; Nov–Dec. 11(6):389–392. PMID: 2281840.


8. Nemechek AJ, Pahlavan N, Cote DN. Nebulized surfactant for experimentally induced otitis media with effusion. Otolaryngol Head Neck Surg. 1997; 11. 117(5):475–479. PMID: 9374170.


9. Koten M, Uzun C, Yagiz R, Adali MK, Karasalihoglu AR, Tatman-Otkun M, et al. Nebulized surfactant as a treatment choice for otitis media with effusion: an experimental study in the rabbit. J Laryngol Otol. 2001; 5. 115(5):363–368. PMID: 11410125.


10. Venkatayan N, Troublefield YL, Connelly PE, Mautone AJ, Chandrasekhar SS. Intranasal surfactant aerosol therapy for otitis media with effusion. Laryngoscope. 2000; 11. 110(11):1857–1860. PMID: 11081599.


11. Chandrasekhar SS, Connelly PE, Venkatayan N, el-Sherif Ammar M, Tabor M, Mautone AJ. Intranasal metered dose aerosolized surfactant reduces passive opening pressure of the eustachian tube: comparison study in two animal models. Otol Neurotol. 2002; 1. 23(1):3–7. PMID: 11773836.


12. Chandrasekhar SS, Mautone AJ. Otitis media: treatment with intranasal aerosolized surfactant. Laryngoscope. 2004; 3. 114(3):472–485. PMID: 15091221.


13. Tegtmeyer FK, Gortner L, Ludwig A, Brandt E. In vitro modulation of induced neutrophil activation by different surfactant preparations. Eur Respir J. 1996; 4. 9(4):752–757. PMID: 8726941.
14. Reiss I, Kuntz S, Schmidt R, Kunz C, Gortner L, Rudloff S. Effect of pulmonary surfactant on TNF-alpha-activated endothelial cells and neutrophil adhesion in vitro. Immunobiology. 2004; 209(3):235–244. PMID: 15518335.
15. Chabot S, Salez L, McCormack FX, Touqui L, Chignard M. Surfactant protein A inhibits lipopolysaccharide-induced in vivo production of interleukin-10 by mononuclear phagocytes during lung inflammation. Am J Respir Cell Mol Biol. 2003; 3. 28(3):347–353. PMID: 12594061.
16. Tooley WH, Clements JA, Muramatsu K, Brown CL, Schlueter MA. Lung function in prematurely delivered rabbits treated with a synthetic surfactant. Am Rev Respir Dis. 1987; 9. 136(3):651–656. PMID: 3307569.


17. Counter SA, Borg E, Engstrom B. Acoustic middle ear reflexes in laboratory animals using clinical equipment: technical considerations. Audiology. 1989; 28(3):135–143. PMID: 2735848.


18. Moller AR. An experimental study of the acoustic impedance of the middle ear and its transmission properties. Acta Otolaryngol. 1965; Jul–Aug. 60(1):129–149. PMID: 14337949.
19. Daniel HJ 3rd, Fulghum RS, Brinn JE, Barrett KA. Comparative anatomy of eustachian tube and middle ear cavity in animal models for otitis media. Ann Otol Rhinol Laryngol. 1982; Jan–Feb. 91(1 Pt 1):82–89. PMID: 7073182.


20. Tos M, Bak-Pedersen K. Goblet cell population in the pathological middle ear and eustachian tube of children and adults. Ann Otol Rhinol Laryngol. 1977; Mar–Apr. 86(2 Pt 1):209–218. PMID: 848832.


21. Yoon YJ, Jeong HS, Kim YK, Lee BK. The distribution of elastic fibers in the Eustachian tube of the rat. Korean J Otolaryngol-Head Neck Surg. 1996; 4. 39(4):568–575.
22. Kabha K, Schmegner J, Keisari Y, Parolis H, Schlepper-Schaeffer J, Ofek I. SP-A enhances phagocytosis of Klebsiella by interaction with capsular polysaccharides and alveolar macrophages. Am J Physiol. 1997; 2. 272(2 Pt 1):L344–L352. PMID: 9124386.


23. McNeely TB, Coonrod JD. Comparison of the opsonic activity of human surfactant protein A for Staphylococcus aureus and Streptococcus pneumoniae with rabbit and human macrophages. J Infect Dis. 1993; 1. 167(1):91–97. PMID: 8418186.


24. Ofek I, Mesika A, Kalina M, Keisari Y, Podschun R, Sahly H, et al. Surfactant protein D enhances phagocytosis and killing of unencapsulated phase variants of Klebsiella pneumoniae. Infect Immun. 2001; 1. 69(1):24–33. PMID: 11119485.
25. Wu H, Kuzmenko A, Wan S, Schaffer L, Weiss A, Fisher JH, et al. Surfactant proteins A and D inhibit the growth of Gram-negative bacteria by increasing membrane permeability. J Clin Invest. 2003; 5. 111(10):1589–1602. PMID: 12750409.


26. Ryan MA, Akinbi HT, Serrano AG, Perez-Gil J, Wu H, McCormack FX, et al. Antimicrobial activity of native and synthetic surfactant protein B peptides. J Immunol. 2006; 1. 176(1):416–425. PMID: 16365435.


27. Kondo H, Suzuki K, Takagi I, Miyamoto N, Baba S, Kobayashi T, et al. Transitional concentration of antibacterial agent to the maxillary sinus via a nebuliser. Acta Otolaryngol Suppl. 1996; 525:64–67. PMID: 8908273.
Fig. 1
Passive opening pressure (POP) measurement in the guinea pig Eustachian tube. Schematic view of measuring the POP.
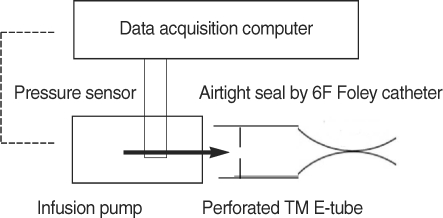
Fig. 2
Computerized recording of the passive opening pressure (POP). (A) Normal control group, (B) Phosphate buffered saline nebulized group, (C) surfactant nebulized group. The asterisk represents the POP of the nebulization with natural surfactant group.
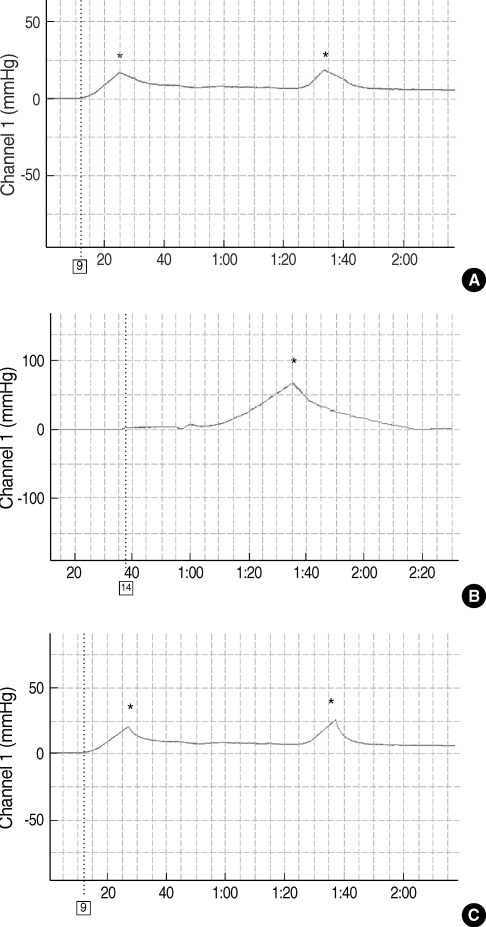
Fig. 3
Effects of nebulization with natural surfactant. The use of nebulized natural surfactant in the guinea pigs with otitis media with effusion produced passive opening pressures (POPs) that were significantly lower than the POPs resulting from the nebulization of phosphate buffered saline (PBS; *P<0.05). LPS: lipolysaccharide.

Fig. 4
The effects of nebulization with natural surfactant or phosphate buffered saline (PBS) on the subepithelial thickness of the bulla (H&E, ×300). (A1) An edematous subepithelial layer remains after nebulization with PBS. Scale bar, 30 µm. (A2) The subepithelial thickness of the bulla mucosa is significantly reduced after nebulization with natural surfactant. Scale bar, 50 µm. (A3) Appearance of the untreated control. Scale bar, 20 µm. (B) The surfactant nebulized group showed a significantly reduced subepithelial thickness as compared to that of the PBS nebulized group (*P<0.05). LPS: lipolysaccharide.
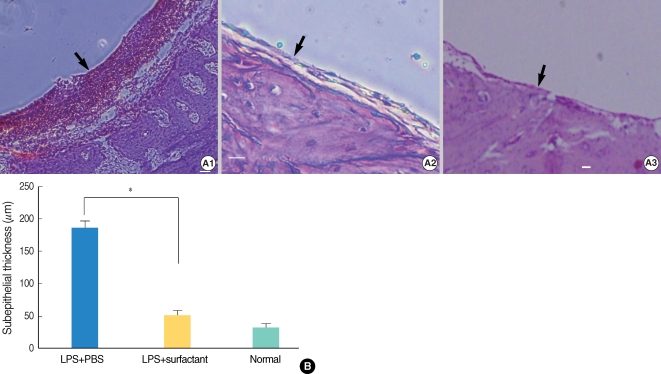