Abstract
Objectives
Our research group has previously demonstrated that hearing loss might be a risk factor for synaptic loss within the hippocampus and impairment of cognition using an animal model of Alzheimer disease. In this study, after inducing hearing loss in a rat model of Alzheimer disease, the associations of various microRNAs (miRNAs) with cognitive impairment were investigated.
Methods
Rats were divided randomly into two experimental groups: the control group, which underwent sham surgery and subthreshold amyloid-β infusion and the deaf group, which underwent bilateral cochlear ablation and subthreshold amyloid-β infusion. All rats completed several cognitive function assessments 11 weeks after surgery, including the object-in-place task (OPT), the novel object recognition task (NOR), the object location task (OLT), and the Y-maze test. After the rats completed these tests, hippocampus tissue samples were assessed using miRNA microarrays. Candidate miRNAs were selected based on the results and then validated with quantitative reverse transcription-polymerase chain reaction (qRT-PCR) analyses.
Results
The deaf group showed considerably lower scores on the OPT, OLT, and Y-maze test than the control group. The microarray analysis revealed that miR-29b-3p, -30e-5p, -153-3p, -376a-3p, -598-3p, -652-5p, and -873-3p were candidate miRNAs, and qRT-PCR showed significantly higher levels of miR-376a-3p and miR-598-3p in the deaf group.
As a result of progressive population aging in modern society, the impact of dementia on health, society, and the economy has become enormous [1]. Although numerous studies have attempted to identify treatments for Alzheimer disease, which is a major cause of dementia [2], no effective therapies have yet been developed [3]. Therefore, the early diagnosis and identification of Alzheimer disease, as well as the management of relevant risk factors, have become practical countermeasures.
A previous study by our research group using an animal model of Alzheimer disease demonstrated that hearing loss was a risk factor for synaptic loss within the hippocampus and impairment of cognition [4]. In this study, amyloid-β was administered to the brain at a level that induces no cognitive impairments, but makes the brain more susceptible to various risk factors [5]. Therefore, this animal model can be used to identify risk factors for Alzheimer disease and may ultimately contribute to the identification of the biological processes through which risk factors, such as hearing loss, contribute to the development of Alzheimer disease. To explore these previous findings in further detail, the present study aimed to identify microRNAs (miRNAs) related to cognitive impairment after hearing loss in a rat model of Alzheimer disease.
miRNAs degrade or suppress mRNAs, leading to posttranscriptional regulation of gene expression [6]. It is generally accepted that the expression of most genes is modulated by miRNAs and that miRNAs are involved in most biological processes [7-9]. Therefore, miRNAs may be valuable biomarkers for the early diagnosis of various diseases. In fact, studies have already reported that miRNAs can serve as early diagnostic biomarkers of various diseases [10-14]. However, to the best of our knowledge, the miRNAs involved in the association between hearing loss and cognitive impairment have yet to be investigated. The identification of miRNAs involved in cognitive impairment after loss of hearing may facilitate the early diagnosis of Alzheimer disease in people suffering from hearing loss.
The Institutional Animal Care and Use Committee of Chung-Ang University (No. 2016-00086) approved all study procedures and ensured that all animal care procedures were performed in accordance with the guidelines provided by this committee. This experiment included Wistar rats (200–250 g) from 7 weeks of age. Both auditory brainstem response (ABR) recordings and surgeries were conducted under anesthesia induced by intraperitoneal administration of xylazine (10 mg/kg; Rompun, Bayer Korea, Seoul, Korea)-mixed ketamine hydrochloride (100 mg/kg; Ketamine, Yuhan Co., Seoul, Korea).
This study included 18 rats that were divided randomly into two experimental groups: (1) control group with sham surgery and subthreshold amyloid-β infusion (n=9) and (2) deaf group which experienced bilateral cochlear ablation and subthreshold amyloid-β Infusion (n=9). ABR tests were performed in every animals prior to surgery to confirm that hearing was normal. The infusion of subthreshold amyloid-β was initiated at 9 weeks after surgery and continued for 2 weeks. All rats completed several cognitive function checks 11 weeks after surgery, including the object-in-place task (OPT), novel object recognition task (NOR), object location task (OLT), and Y-maze test. Following the tests for cognitive function, ABR recordings were conducted in all animals and then the hippocampus tissue samples were obtained; three samples were randomly selected from each of the control and deaf groups. miRNA microarrays were performed on these samples and candidate miRNAs were selected based on the results. To validate the candidate miRNAs, quantitative reverse transcription-polymerase chain reaction (qRT-PCR) analyses were performed on the remaining six samples from each group.
ABR recordings were performed as previously described [4]. In brief, to measure hearing levels, ABR studies are carried out before surgery and 1 week, 6 weeks and 11 weeks after surgery using High-frequency transducers (HFT9911–20–0035) and SmartEP software ver. 2.3 (Intelligent Hearing Systems, Glenvar Heights, FL, USA). We used tone pips at 8, 16, and 32 kHz as the sound stimulus (5-ms duration, cos shaping, 21 Hz) and reduced the intensity of the stimulus by 5 dB sound pressure level (SPL). The hearing threshold was defined as the lowest stimulus intensity that evoked an identifiable response determined by two researches who were blind to the test conditions.
As previously described, cochlear ablation was carried out on both sides [15]. In brief, the external auditory canal was opened after a retroauricular incision. The tympanic membrane and ossicles were removed, with the exception of the stapes. After identifying the cochlear bony wall, a small hole was made and we used a dental pick to ablate the contents of the cochlea. The empty space was stuffed with a small amount of soft tissue through the small hole on the cochlear bony wall. A retroauricular incision was done and sutured in the sham operation without exposing the auditory external canal.
As mentioned previously, behavioral tests for vestibular impairment were preformed 1 day and 1 week after surgery to rule out the consequences of vestibular dysfunction that may occur during cochlear ablation [16]. In short, the behavioral assessment of vestibular function composed of three components: head roll tilt, postural asymmetry, and nystagmus (Supplementary Table 1). The subject was dropped out from the study if any of these components were impaired.
The amyloid-β peptide solution was injected as previously described [4]. A mini-osmotic pump was filled with the amyloid-β peptide solution which was made by dissolving an amyloid-β1-42 peptide solution (AnaSpec Inc., San Jose, CA, USA) in 35% acetonitrile/0.1% trifluoroacetic acid. This solution has been proven to not induce cognitive impairment in a previous study [4]. Then, we connected the mini-osmotic pump (Osmotic Pump 2002; Alzet, Cupertino, CA, USA) to a brain infusion cannula (Brain Infusion Kit 2, Alzet), and implanted the brain infusion cannula into the right lateral ventricle of brain (anteroposterior, −0.3; lateral, 1.2; vertical, 4.5) in accordance with the Paxinos and Watson coordinates [17]. The solution of amyloid-β peptide was infused into the intracerebroventricular space for 2 weeks on a continuous basis (160 pmol/day).
The Y-maze test was performed as previously described [4]. Briefly, A rat was put on the tip of a Y-maze arm and allowed to travel around the maze for 7 minutes without any restrictions. The test was recorded and analyzed later. Successive entries into three arms are described as alternation based on overlapping triplets. The alternation percentage was assessed as follows: number of actual alternations/number of possible alternations (2 minus the total number of times rat goes into arm). None of the rats in the present study had ever previously experienced a Y-maze.
The OPT, OLT, and NOR were performed as previously described [4]. Briefly, each session included of phases of familiarization and test. In the test phase, the location or type of the stimulus object is varied from the familiarization phase; the conditions of the test are included in Supplementary Fig. 1. The experimenter was not in the room during the test, which was recorded and analyzed later. The rats encountered stimulus objects in the open field box for 5 minutes during the familiarization phase and were then confined to their home cage for a fixed period of time, i.e. 5 minutes for the OPT and OLT and 3 hours for the NOR. Then the rats were put in the box again in the test phase and permitted to investigate the stimulus objects. Exploration behavior was defined as contact the nose or paws of the item or directing the nose toward an object at a distance <2 cm and a discrimination ratio was determined as follows: (exploration time with the changed object–exploration time with the unchanged object)/(total exploration time with the changed and unchanged object). The data were excluded from the study when exploration time was less than 15 seconds during the familiarization phase or less than 10 seconds during the test phase.
BioCore Co., (Seoul, Korea) performed microarray analysis of the miRNAs as previously described [16]. Briefly, the RNA was extracted from the tissue sample using TRI REAGENT (Molecular Research Center, Cincinnati, OH, USA) according to the manufacturer’s instructions. The quality and quantity of the RNA were assayed with NanoDrop 1000 spectrophotometer (Thermo Scientific, Madison, WI, USA) and Agilent 2100 BioAnalyzer (Agilent Technologies, Santa Clara, CA, USA). The RNA was labeled with the FlashTag Biotin RNA Labeling Kit (Affymetrix Inc., Santa Clara, CA, USA) and hybridized to GeneChip miRNA 4.0 microarrays (Affymetrix Inc.). Hybridization was carried out with GeneChip Hybridization Oven 640 (Affymetrix Inc.) at 48°C for 16 hours. The arrays were cleaned as specified by the manufacturer’s instructions. GeneChip Scanner 3000 (Affymetrix Inc.) was used to scan all arrays and a raw analysis was performed with Transcriptome Analysis Console software (Affymetrix Inc.). The images of raw data generated by the scanner were translated into CEL files containing the measurement concentrations of each probe in the array. The CEL files were imported into the Gene Expression Workflow in GeneSpring GX ver. 14.9.1 (Agilent Technologies) and the default settings in the GeneSpring software were used to setting background correction, log2 transformation, and probe set summarization. A covariance dispersion matrix was used to conduct principal component analysis as the data quality control; gene-level differential expression was predicted between the two groups. Unpaired t-tests were independently performed to compare the individual gene expression data between the control and deaf groups.
Total RNA was isolated from hippocampal tissues using QIAzol Lysis Reagent (Qiagen, Hilden, Germany) according to the manufacturer’s manual. The concentrations of the samples were confirmed using the NanoDrop spectrophotometer (Thermo Scientific). cDNA was generated by the reverse transcription of RNA using the miScript II RT kit (Qiagen). qPCR was conducted using Bio-Rad CFX 96 real-time system (Bio-RAD, Hercules, CA, USA) with an miScript SYBR Green PCR kit (Qiagen). All PCR reactions were performed under standard PCR conditions. U6 was used as an endogenous control. Relative quantification (RQ) values were calculated using the comparative 2−ΔΔCt method.
We performed statistical analyses using IBM SPSS ver. 21.0 (IBM Corp., Armonk, NY, USA). ABR thresholds, scores on the cognitive tests and RQ values from the qRT-PCR results were analyzed with Mann-Whitney U-tests. P-values <0.05 were considered to indicate statistical significance.
The ABR thresholds at all frequencies were between 20 and 35 dB SPL in both groups prior to surgery; these levels were not significantly different between the groups (P>0.05). The ABR thresholds at all frequencies were between 20 and 35 dB SPL in the control group at 1 week, 6 weeks, and 11 weeks after surgery, whereas the ABR thresholds in the deaf group were higher than 80 dB SPL (Fig. 1). No animals showed vestibular deficits after surgery.
The results of the cognitive tests are presented in Fig. 2. The deaf group had somewhat lower scores than the control group on the OPT, OLT, and Y-maze test (P<0.05). There were no noteworthy differences in the results of the NOR test between the groups.
Candidate miRNAs were selected based on the microarray analysis (Supplementary Material 1). After excluding miRNAs that are not expressed in humans, those that satisfied the following criteria were selected: a fold change of >1.50 or <0.67 of the normalized intensity values between the control and deaf groups (P<0.10; Student t-test). Through this process, miR-29b-3p, -30e-5p, -153-3p, -376a-3p, -598-3p, -652-5p, and -873-3p were identified as candidate miRNAs.
To validate the candidate miRNAs, qRT-PCR analyses were performed. miRNAs that had significantly different RQ values between the control and deaf groups (P<0.05) were selected. The RQ values of miR-376a-3p and miR-598-3p significantly differed between the control and deaf groups, whereas those of miR-29b-3p, -30e-5p, and -153-3p did not show significant between-group differences (Fig. 3). The cycle threshold values of miR-652-5p and miR-873-3p were determined to be >35 even though the total RNA amount increased and several experiments were conducted.
Several epidemiological studies have suggested that hearing loss is a risk factor for dementia [18-21], which is a very important finding because hearing loss is common among the elderly. This relationship has two implications for the prevention and early diagnosis of dementia. First, it is possible that the occurrence of dementia can be reduced by hearing rehabilitation. Second, identifying biomarkers associated with the development of dementia in patients with hearing loss would enable the early diagnosis of dementia in those suffering from hearing loss. Our research group proposes that miRNAs may be such a biomarker. Since a single miRNA regulates the expression of multiple genes and most genes are under the control of miRNAs, it logically follows that most biological events are controlled by miRNAs [7-9]. Therefore, studies are actively investigating the role of miRNAs as early diagnostic markers for various diseases. Recently, extensive research has been conducted to assess the roles of miRNAs as diagnostic markers in various degenerative neurological diseases, including dementia [22].
Our research group used an animal model to identify miRNAs associated with cognitive impairment after hearing loss and demonstrated that hearing loss is a risk factor for synaptic loss within the hippocampus and impairment of cognition [4]. In this study, two groups were analyzed to identify miRNAs involved in cognitive impairment after hearing loss. One group was administered amyloid-β while maintaining normal hearing, while the other group was administered amyloid-β after hearing loss was induced. In accordance with our previous experimental results [4], the hearing loss group exhibited lower cognitive function related to the hippocampus than the normal hearing group. Additionally, in the present study, between-group comparisons of miRNA expression levels in the hippocampus revealed that miR-376a-3p and miR-598-3p levels were higher in the hearing loss group. Expression levels of miR-376a-3p and miR-598-3p in the central nervous system have been assessed in previous studies. For instance, it was found that miR-376a-3p levels increase in the hippocampus during prion disease [23], and an association between the aberrant expression of miR-376 and human gliomas has been proposed [24]. Additionally, miR-598-3p levels increase following traumatic brain injury [25]. Therefore, it is plausible that miR-376a-3p and miR-598-3p are related to cognitive impairment after hearing loss.
Several steps remain before the present results can be applied in clinical practice. First, miRNA expression was only measured in rat hippocampal tissues in the present study. To apply these findings in clinical practice, further studies should be carried out to determine whether miRNAs can be measured in the human cerebrospinal fluid and/or blood. Secondly, future studies should investigate which genes are regulated by miR-376a-3p and miR-598-3p. Nonetheless, the present results may be a starting point for the development of biomarkers for the early diagnosis of dementia. Additionally, future studies may develop miR-376a-3p and miR-598-3p as early diagnostic markers of cognitive function impairment in patients with hearing loss.
ACKNOWLEDGMENTS
This work was supported by a National Research Foundation of Korea (NRF) grant funded by the Korea government (Ministry of Science and ICT) (No. NRF-2016R1C1B2007131 to MC).
Supplementary Materials
Supplementary Fig. 1.
Diagrams of object-in-place task (OPT), object location task (OLT), and novel object recognition task (NOR). (A) Familiarization phase of the OPT. (B) Test phase of the OPT. (C) Familiarization phase of the OLT. (D) Test phase of the OLT. (E) Familiarization phase
of the NOR. (F) Test phase of the NOR.
REFERENCES
1. GBD 2016 Dementia Collaborators. Global, regional, and national burden of Alzheimer’s disease and other dementias, 1990-2016: a systematic analysis for the Global Burden of Disease Study 2016. Lancet Neurol. 2019; Jan. 18(1):88–106.
2. Ballard C, Gauthier S, Corbett A, Brayne C, Aarsland D, Jones E. Alzheimer’s disease. Lancet. 2011; Mar. 377(9770):1019–31.


3. Cao J, Hou J, Ping J, Cai D. Advances in developing novel therapeutic strategies for Alzheimer’s disease. Mol Neurodegener. 2018; Dec. 13(1):64.


4. Chang M, Kim HJ, Mook-Jung I, Oh SH. Hearing loss as a risk factor for cognitive impairment and loss of synapses in the hippocampus. Behav Brain Res. 2019; Oct. 372:112069.


5. Tran TT, Srivareerat M, Alkadhi KA. Chronic psychosocial stress triggers cognitive impairment in a novel at-risk model of Alzheimer’s disease. Neurobiol Dis. 2010; Mar. 37(3):756–63.


6. Hollins SL, Cairns MJ. MicroRNA: small RNA mediators of the brains genomic response to environmental stress. Prog Neurobiol. 2016; Aug. 143:61–81.


7. Campbell K, Booth SA. MicroRNA in neurodegenerative drug discovery: the way forward? Expert Opin Drug Discov. 2015; Jan. 10(1):9–16.


9. Gebert LF, Rebhan MA, Crivelli SE, Denzler R, Stoffel M, Hall J. Miravirsen (SPC3649) can inhibit the biogenesis of miR-122. Nucleic Acids Res. 2014; Jan. 42(1):609–21.


10. Huang Z, Chen W, Du Y, Guo Q, Mao Y, Zhou X, et al. Serum miR-16 as a potential biomarker for human cancer diagnosis: results from a large-scale population. J Cancer Res Clin Oncol. 2019; Mar. 145(3):787–96.


11. Ng PC, Chan KY, Yuen TP, Sit T, Lam HS, Leung KT, et al. Plasma miR-1290 is a novel and specific biomarker for early diagnosis of necrotizing enterocolitis-biomarker discovery with prospective cohort evaluation. J Pediatr. 2019; Feb. 205:83–90. e10.


12. Parizadeh SM, Jafarzadeh-Esfehani R, Ghandehari M, Goldani F, Parizadeh SM, Hassanian SM, et al. MicroRNAs as potential diagnostic and prognostic biomarkers in hepatocellular carcinoma. Curr Drug Targets. 2019; 20(11):1129–40.


13. Rezayi M, Farjami Z, Hosseini ZS, Ebrahimi N, Abouzari-Lotf E. MicroRNA-based biosensors for early detection of cancers. Curr Pharm Des. 2018; 24(39):4675–80.


14. Weis A, Marquart L, Calvopina DA, Genz B, Ramm GA, Skoien R. Serum microRNAs as biomarkers in hepatitis C: preliminary evidence of a microRNA panel for the diagnosis of hepatocellular carcinoma. Int J Mol Sci. 2019; Feb. 20(4):864.


15. Mun SK, Han KH, Baek JT, Ahn SW, Cho HS, Chang MY. Losartan prevents maladaptive auditory-somatosensory plasticity after hearing loss via transforming growth factor-β signaling suppression. Clin Exp Otorhinolaryngol. 2019; Feb. 12(1):33–9.


16. Chang MY, Park S, Choi JJ, Kim YK, Suh MW, Lee JH, et al. MicroRNAs 218a-5p, 219a-5p, and 221-3p regulate vestibular compensation. Sci Rep. 2017; Aug. 7(1):8701.


17. Paxinos G, Watson C. The rat brain in stereotaxic coordinates. Burlington (MA): Academic Press;2006.
18. Gallacher J, Ilubaera V, Ben-Shlomo Y, Bayer A, Fish M, Babisch W, et al. Auditory threshold, phonologic demand, and incident dementia. Neurology. 2012; Oct. 79(15):1583–90.


19. Kiely KM, Gopinath B, Mitchell P, Luszcz M, Anstey KJ. Cognitive, health, and sociodemographic predictors of longitudinal decline in hearing acuity among older adults. J Gerontol A Biol Sci Med Sci. 2012; Sep. 67(9):997–1003.


20. Lin FR, Metter EJ, O’Brien RJ, Resnick SM, Zonderman AB, Ferrucci L. Hearing loss and incident dementia. Arch Neurol. 2011; Feb. 68(2):214–20.


21. Lin FR, Yaffe K, Xia J, Xue QL, Harris TB, Purchase-Helzner E, et al. Hearing loss and cognitive decline in older adults. JAMA Intern Med. 2013; Feb. 173(4):293–9.


22. Brennan S, Keon M, Liu B, Su Z, Saksena NK. Panoramic visualization of circulating microRNAs across neurodegenerative diseases in humans. Mol Neurobiol. 2019; 56(11):7380–407.


23. Boese AS, Saba R, Campbell K, Majer A, Medina S, Burton L, et al. MicroRNA abundance is altered in synaptoneurosomes during prion disease. Mol Cell Neurosci. 2016; Mar. 71:13–24.


Fig. 1.
Auditory brainstem response (ABR) threshold results before cochlear ablation and 1, 6, and 11 weeks after surgery. (A) Control group (n=9). (B) Deaf group (n=9). The error bars indicate standard deviation. SPL, sound pressure level.
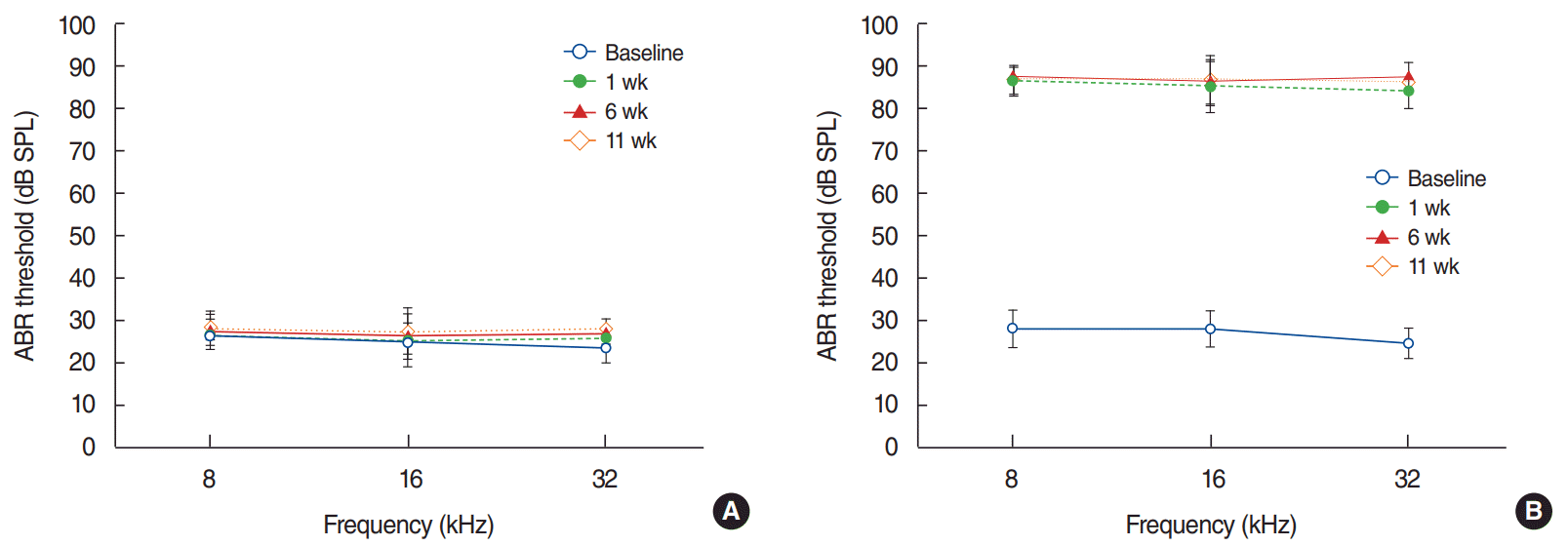
Fig. 2.
Results of cognitive tests. The deaf group (n=9) had somewhat lower scores than the control group (n=9) on the object-inplace task (OPT), object location task (OLT), and Y-maze test. NOR, novel object recognition task. The error bars indicate standard error. ***P<0.001 between the groups according to the Mann-Whitney test.
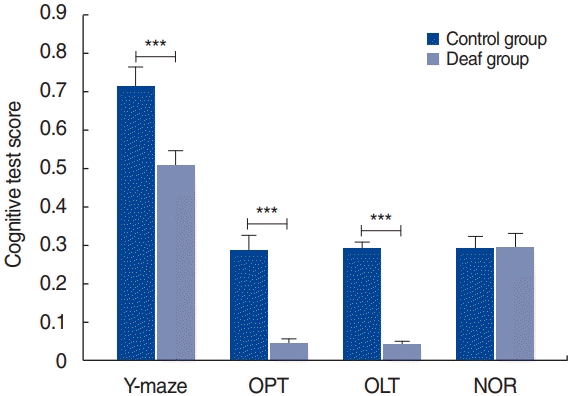
Fig. 3.
Quantitative reverse transcription-polymerase chain reaction of candidate microRNAs. The relative quantification (RQ) values of miR-376a-3p and miR-598-3p significantly differed between the control (n=6) and deaf (n=6) groups. The error bars indicate standard error. **P<0.01 between the groups according to the MannWhitney test.
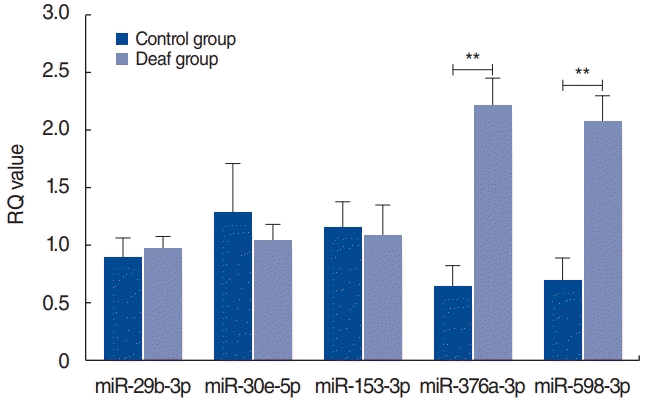