Abstract
Multicellular spheroids (MCSs) from three dimensional culture, which is a complex architectural structure with dynamic cell to cell and cell to matrix interactions, mimic real tissues regarding structural and functional properties. MCSs have emerged as an effective tool for filling up the gap between the in vitro and in vivo experimental model and can replace the in vivo model. The viability and functional performance can be enhanced when cells are grown as multicellular spheroid (MCS). In this review paper, we discussed the basic mechanism of MCS formation, their biomedical applications, and recent advances in MCS culture and tissue engineering.
Moscona & Moscona(advances in multicellular spheroids formation-5)에 의해 처음 소개된 3차원 장기유사체는 세포간 그리고 세포와 기질간의 상호작용을 동반한 세포의 집합체로 생체의 구조와 기능을 모사하여 생체 내(in-vivo)실험과 유사한 환경을 구현함으로써 이미 다양한 기초 생물학과 임상 적용을 목표로 하는 다양한 연구에 활용되고 있다. 과거, 오가노이드란 단어는 1차 조직(primary tissue) 또는 그보다 하위 1차 세포(primary cell), 배아 줄기세포(embryonic stem cell) 및 유도만능줄기세포(induced pluripotent stem cell), 세포주(cell line), 그리고 여러 조직 유형들로 구성된 장기 외식편(explant)과 같은 전체 또는 분절된 기관에서 비롯된 모든 3차원 기관형 배양체(organotypic culture)를 포함하여 일컫는 용어였다. 그러나, 최근 많은 문헌들에서는 장기모사체를 줄기세포로부터 분화하여 체외(in-vitro)에서 다세포 인체유사조직으로 분화시켜 생산하는 오가노이드(Organoid)와 이미 분화된 인체장기의 세포를 분리하여 체외(in-vitro)에서 3차원 배양에 의해 구모양으로 만들어지는 스페로이드(spheroid)로 크게 구분하여 부르기도 한다[1].
오가노이드 또는 스페로이드와 같은 3차원 배양기법이 2차원 단층배양에 비해 생리학적으로 실제 생체 내 환경과 매우 유사하고, 생존능력(viability)과 기능성이 향상되며 생체 내 모델보다 주변미세환경 구성요소(niche component), 신호 전달 경로 및 유전체 조작을 통한 기능향상이 훨씬 더 간편하기 때문에 전통적인 2차원 단층배양과 생체 내 동물/인간 모델사이의 중요한 역할을 할 수 있다[2].
본 문헌에서는 최근 다양한 기초연구 분야 뿐만 아니라 임상적인 적용까지 시도되고 있는 최신 3차원 세포배양기법들을 분화된 1차 세포를 이용한 스페로이드 제작법에 중점을 두어 설명하고자 한다.
스페로이드를 형성하기 위한 세포간 유착 및 분화는 1) 인테그린(integrin)과 세포외기질(extracellular matrix)의 결합에 의한 불완전한 세포응집(cell aggregation), 2) 캐드헤린(cadherin)의 발현과 축적, 3) 캐드헤린 간(cadherin to cadherin)의 동종친화성 상호작용(homophilic interaction)에 의한 구체(spheroid)조직 형성의 3단계로 구분된다(Fig. 1)[3].
스페로이드는 생성초기에는 혈관성이 없는 조직(avascular tissue)으로 150~200 μm 이내의 제한된 범위 내에서 확산(diffusion)에 의해 필요한 물질과 산소를 외부로부터 공급받게 되며 내부의 대사노폐물을 배출한다.
따라서, 직경 500 μm를 초과하는 스페로이드는 흔히 내부의 괴사조직(necrotic core)과 외부의 생존조직(viable rim)이 띠 모양으로 층구조를 형성하고 있으며 생존조직은 다시 내부의 휴지기세포 구역(quiescent viable cell zone)과 증식세포구역(proliferating zone)으로 나뉜다(Fig. 2).
이렇게 생성된 스페로이드는 2차원 단층배양된 조직과 비교하여 더 생존력이 높고 다양한 유전자가 발현되며 이 유전자들은 모사하고자 하는 생체 조직에 고유한 유전자 전사인자를 포함하고 있어 실제 생체 조직과 더 유사한 기능을 갖게 된다고 보고된 바 있다[4].
특히, 이러한 고유한 조직 특성을 효과적이고 안정적으로 발현하는 장점은 종양 연구에 있어 매우 가치 있는 점이라 할 수 있겠다[5].
현적배양(haning-drop cultures)은 세포액 방울이 배양 접시 위에 위치한 배양판에 매달린 채로 방울 내부의 세포들이 중력에 의해 아래에 응집하여 조직을 형성하게 되는 원리이다(Figs. 3A and 4). 각 세포 방울의 용량은 15~30 μL 크기이며 각 세포액 방울 당 대략 300~3000개의 세포를 내부에 포함할 수 있다[3,12]. 4~7일 후 스페로이드 조직이 완성되면 세포방울이 아래쪽에 매달린 모래시계 모양의 마이크로채널(microchannel)의 위쪽 입구에 세포액을 주입하는 방식으로 완성된 스페로이드를 아래로 밀어내 내부에 유착방지처리 된 배양용기에 담아 배양하게 된다. 이 기법은 스페로이드의 크기, 내부의 세포수와 세포구성을 균일하게 조절하여 제작할 수 있는 장점이 있어 스페로이드 제작 중 발생할 수 있는 세포 및 분자단위의 현상에 대한 연구, 종양의 침습에 대한 연구, 이종의 세포간 상호작용 및 조직의 혈관화 과정에 대한 연구에 적용되고 있다[13-15].
가장 단순하면서 용이한 방법으로 세포가 표면에 유착되지 않게 내부처리 된 하단이 둥근 마이크로 웰 플레이트(microwell plate with non-adhesive surface) 내에 세포를 함유한 배양액을 담아 중력에 의해 세포들이 아래로 모이면서 둥근 조직을 형성하게 되는 원리로 초심자들도 쉽게 시행할 수 있는 용이한 방법이면서 균일한 크기와 세포조성을 조절할 수 있는 장점이 있다(Fig. 3B). 다만, 현적배양에 비해 크기의 균일성이 다소 떨어질 수 있으나 다른 방법들에 비해 비교적 균일한 크기의 스페로이드를 만들 수 있고, 방법 또한 현적배양에 비해 용이하여 가장 흔하게 이용되는 방법이라고 볼 수있다. 이 방법의 경우 현적배양과 같이 다양한 연구분야에 적용되고 있으며 스페로이드의를 세포액 내의 세포농도 및 마이크로 웰의 크기를 조절함으로써 다양한 크기로 제작가능하다[16,17].
내부에 유착방지처리 된 회전 플라스크(spinner flask)(Fig. 3D)와 롤러 보틀(roller bottle)(Fig. 3E)을 이용하여 대량으로 스페로이드를 제작할 수 있는 방법으로 정적인 배양법에 비해 동적으로 회전하는 배양용기 안에서 만들어지는 스페로이드는 상대적으로 그 모양에 있어 균일하며 동시에 대량의 스페로이드를 제작할 수 있고, 크기 또한 회전 속도와 세포액의 세포농도를 조절하여 다양하게 제작할 수 있는 장점이 있다. 반면 회전 플라스크와 같이 수평면으로 회전하는 배양 용기의 회전속도가 너무 빠르게 되면 전단력(shear force)이 강해져 세포의 생리학적 반응에 영향을 줄 수 있는 단점이있다[24,25]. 최근 미국의 National Aeronautics and Space Administration에서 이를 보완하기 위해 전단력을 낮추고, 대신 중력을 더해 구르는 운동으로 회전을 대신한 롤러 보틀을 개발하여 회전 플라스크를 대체하고 있다[26,27].
최근 기능화된 표면재료들을 이용한 스페로이드 제작법들이 소개되고 있다. 대표적인 예로 표면에 양전하를 띤 Primaria dishes(BD Biosciences, Franklin Lakes, NJ, USA)(Fig. 3F)로 세포를 배양하게 되면 배양 초기에는 세포들이 표면에 단층으로 퍼져서 배양되다가 48시간 후에는 actin filament를 매개로 한 조직 재구성 과정으로 세포들이 구축, 이동과 전이의 과정을 거쳐 2차원적인 단층구조에서 3차원 구체모양의 구조로 말려들게 된다. 유사한 재료로 galactosylated poly(vinylidene difluoride) 또는 polyethylene terephthalate film이 있다[28,29].
온도차를 이용한 방법 또한 소개된 바 있는데, thermosresponsive poly(N-isopropylacrylamide)로 처리된 표면의 배양용기에 세포를 배양시키면 용기 바닥으로부터 부유하는 단층구조의 세포층이 형성되고 배양온도를 낮추게 되면 세포층의 가장자리부터 말려드는 수축현상이 발생하여 구체모양의 조직을 형성하게 되는 원리이다(Fig. 3G). 상기 방법들로 만들어진 스페로이드는 원심분리를 통해 용기로부터 분리해낼 수 있다[30-32]. 기타 polyurethane foam으로 제작한 다공성 3-D 스캐폴드(porous 3-D scaffold)를 이용하여 세포들의 자가 응집을 물리적으로 지지하는 방법도 있다[33,34].
보고된 수 많은 연구에서 보여주듯 스페로이드를 포함한 생체모사체에 대한 연구는 생리-의학분야의 주된 연구 모델이 되어가고 있다. 더불어 공초점 레이져주사현미경(confocal laser scanning microscope), 다양한 조영제를 이용한 마이크로 자기공명현미경(micro-magnetic resonance microscopy), 양전자방출단층촬영(positron-emission tomography)및 광학단층촬영기법(optical sectioning technology)을 이용한 스페로이드 내부의 대사(metabolism), 세포의 생존과 괴사(apoptosis)와 같은 생리작용을 시각화하여 측정할 수 있는 영상기법들 또한 빠르게 발전하고 있다[40-42]. 이러한 기법들이 스페로이드의 기능을 효율적으로 향상시키는 다양한 기법들과 결합되어 조직의 생성, 변화과정과 나아가 유전자 발현을 실시간으로 시각화할 수 있는 연구로 발전해 나갈 것이며 궁극적으로는 인공장기 또는 임상적으로 적용가능한 이식체 개발에 중요한 역할을 할 것으로 예상된다.
REFERENCES
1. Fatehullah A, Tan SH, Barker N. Organoids as an in vitro model of human development and disease. Nat Cell Biol. 2016; 18(3):246–54.


2. Cui X, Hartanto Y, Zhang H. Advances in multicellular spheroids formation. J R Soc Interface. 2017; 14(127):20160877.


3. Lin RZ, Chou LF, Chien CC, Chang HY. Dynamic analysis of hepatoma spheroid formation: Roles of E-cadherin and beta1-integrin. Cell Tissue Res. 2006; 324(3):411–22.
4. Narayanan RA, Rink A, Beattie CW, Hu WS. Differential gene expression analysis during porcine hepatocyte spheroid formation. Mamm Genome. 2002; 13(9):515–23.


5. De Witt Hamer PC, Van Tilborg AA, Eijk PP, Sminia P, Troost D, Van Noorden CJF, et al. The genomic profile of human malignant glioma is altered early in primary cell culture and preserved in spheroids. Oncogene. 2008; 27(14):2091–6.


6. Kunz-Schughart LA, Freyer JP, Hofstaedter F, Ebner R. The use of 3-D cultures for high-throughput screening: The multicellular spheroid model. J Biomol Screen. 2004; 9(4):273–85.


7. Weaver VM, Lelièvre S, Lakins JN, Chrenek MA, Jones JCR, Giancotti F, et al. beta4 integrin-dependent formation of polarized three-dimensional architecture confers resistance to apoptosis in normal and malignant mammary epithelium. Cancer Cell. 2002; 2(3):205–16.
8. Debnath J, Mills KR, Collins NL, Reginato MJ, Muthuswamy SK, Brugge JS. The role of apoptosis in creating and maintaining luminal space within normal and oncogene-expressing mammary acini. Cell. 2002; 111(1):29–40.


9. Korff T, Kimmina S, Martiny-Baron G, Augustin HG. Blood vessel maturation in a 3-dimensional spheroidal coculture model: Direct contact with smooth muscle cells regulates endothelial cell quiescence and abrogates VEGF responsiveness. FASEB J. 2001; 15(2):447–57.


10. Kelm JM, Ehler E, Nielsen LK, Schlatter S, Perriard JC, Fussenegger M. Design of artificial myocardial microtissues. Tissue Eng. 2004; 10(1-2):201–14.


11. Mironov V, Boland T, Trusk T, Forgacs G, Markwald RR. Organ printing: Computer-aided jet-based 3D tissue engineering. Trends Biotechnol. 2003; 21(4):157–61.


12. Kelm JM, Timmins NE, Brown CJ, Fussenegger M, Nielsen LK. Method for generation of homogeneous multicellular tumor spheroids applicable to a wide variety of cell types. Biotechnol Bioeng. 2003; 83(2):173–80.


13. Kelm JM, Fussenegger M. Microscale tissue engineering using gravity-enforced cell assembly. Trends Biotechnol. 2004; 22(4):195–202.


14. de Ridder L, Cornelissen M, de Ridder D. Autologous spheroid culture: A screening tool for human brain tumour invasion. Crit Rev Oncol Hematol. 2000; 36(2-3):107–22.
15. Wartenberg M, Dönmez F, Ling FC, Acker H, Hescheler J, Sauer H. Tumor-induced angiogenesis studied in confrontation cultures of multicellular tumor spheroids and embryoid bodies grown from pluripotent embryonic stem cells. FASEB J. 2001; 15(6):995–1005.


16. Yuhas JM, Li AP, Martinez AO, Ladman AJ. A simplified method for production and growth of multicellular tumor spheroids. Cancer Res. 1977; 37(10):3639–43.
17. Hamilton GA, Westmorel C, George AE. Effects of medium composition on the morphology and function of rat hepatocytes cultured as spheroids and monolayers. In Vitro Cell Dev Biol Anim. 2001; 37(10):656–67.


18. Wu LY, Di Carlo D, Lee LP. Microfluidic self-assembly of tumor spheroids for anticancer drug discovery. Biomed Microdevices. 2008; 10(2):197–202.


19. Napolitano AP, Chai P, Dean DM, Morgan JR. Dynamics of the self-assembly of complex cellular aggregates on micromolded nonadhesive hydrogels. Tissue Eng. 2007; 13(8):2087–94.


20. Dean DM, Napolitano AP, Youssef J, Morgan JR. Rods, tori, and honeycombs: The directed self-assembly of microtissues with prescribed microscale geometries. FASEB J. 2007; 21(14):4005–12.


21. Karp JM, Yeh J, Eng G, Fukuda J, Blumling J, Suh KY, et al. Controlling size, shape and homogeneity of embryoid bodies using poly(ethylene glycol) microwells. Lab Chip. 2007; 7(6):786–94.


22. Khademhosseini A, Eng G, Yeh J, Fukuda J, Blumling J 3rd, Langer R, et al. Micromolding of photocrosslinkable hyaluronic acid for cell encapsulation and entrapment. J Biomed Mater Res A. 2006; 79(3):522–32.


23. Fukuda J, Khademhosseini A, Yeo Y, Yang X, Yeh J, Eng G, et al. Micromolding of photocrosslinkable chitosan hydrogel for spheroid microarray and co-cultures. Biomaterials. 2006; 27(30):5259–67.
24. Nyberg SL, Hardin J, Amiot B, Argikar UA, Remmel RP, Rinaldo P. Rapid, large-scale formation of porcine hepatocyte spheroids in a novel spheroid reservoir bioartificial liver. Liver Transpl. 2005; 11(8):901–10.


25. Song H, David O, Clejan S, Giordano CL, Pappas-Lebeau H, Xu L, et al. Spatial composition of prostate cancer spheroids in mixed and static cultures. Tissue Eng. 2004; 10(7-8):1266–76.


26. Ingram M, Techy GB, Saroufeem R, Yazan O, Narayan KS, Goodwin TJ, et al. Three-dimensional growth patterns of various human tumor cell lines in simulated microgravity of a NASA bioreactor. In Vitro Cell Dev Biol Anim. 1997; 33(6):459–66.


28. Du Y, Han R, Ng S, Ni J, Sun W, Wohland T, et al. Identification and characterization of a novel prespheroid 3-dimensional hepatocyte monolayer on galactosylated substratum. Tissue Eng. 2007; 13(7):1455–68.


29. Lu HF, Lim WS, Wang J, Tang ZQ, Zhang PC, Leong KW, et al. Galactosylated PVDF membrane promotes hepatocyte attachment and functional maintenance. Biomaterials. 2003; 24(27):4893–903.


30. Takezawa T, Mori Y, Yoshizato K. Cell culture on a thermo-responsive polymer surface. Biotechnology (NY). 1990; 8(9):854–6.


31. Takezawa T, Mori Y, Yonaha T, Yoshizato K. Characterization of morphology and cellular metabolism during the spheroid formation by fibroblasts. Exp Cell Res. 1993; 208(2):430–41.


32. Ueno K, Miyashita A, Endoh E, Takezawa T, Yamazaki M, Mori Y, et al. Formation of multicellular spheroids composed of rat hepatocytes. Res Commun Chem Pathol Pharmacol. 1992; 77(1):107–20.
33. Elkayam T, Amitay-Shaprut S, Dvir-Ginzberg M, Harel T, Cohen S. Enhancing the drug metabolism activities of C3A--a human hepatocyte cell line--by tissue engineering within alginate scaffolds. Tissue Eng. 2006; 12(5):1357–68.


34. Lin KH, Maeda S, Saito T. Long-term maintenance of liver-specific functions in three-dimensional culture of adult rat hepatocytes with a porous gelatin sponge support. Biotechnol Appl Biochem. 1995; 21(1):19–27.
35. Akiyama M, Nonomura H, Kamil SH, Ignotz RA. Periosteal cell pellet culture system: A new technique for bone engineering. Cell Transplant. 2006; 15(6):521–32.


36. Sebastian A, Buckle AM, Markx GH. Tissue engineering with electric fields: Immobilization of mammalian cells in multilayer aggregates using dielectrophoresis. Biotechnol Bioeng. 2007; 98(3):694–700.


37. Ino K, Ito A, Honda H. Cell patterning using magnetite nanoparticles and magnetic force. Biotechnol Bioeng. 2007; 97(5):1309–17.


38. Torisawa YS, Takagi A, Nashimoto Y, Yasukawa T, Shiku H, Matsue T. A multicellular spheroid array to realize spheroid formation, culture, and viability assay on a chip. Biomaterials. 2007; 28(3):559–66.
39. Mohr JC, de Pablo JJ, Palecek SP. 3-D microwell culture of human embryonic stem cells. Biomaterials. 2006; 27(36):6032–42.


40. Louie AY, Hüber MM, Ahrens ET, Rothbächer U, Moats R, Jacobs RE, et al. In vivo visualization of gene expression using magnetic resonance imaging. Nat Biotechnol. 2000; 18(3):321–25.


41. Santini MT, Romano R, Rainaldi G, Indovina P, Ferrante A, Motta A, et al. Temporal dynamics of 1H-NMR-visible metabolites during radiation-induced apoptosis in MG-63 human osteosarcoma spheroids. Radiat Res. 2006; 166(5):734–45.
Fig. 1.
Inverted bright field microscopic images of spheroids from single cell culture on non-adhesive surface in chronological sequence.
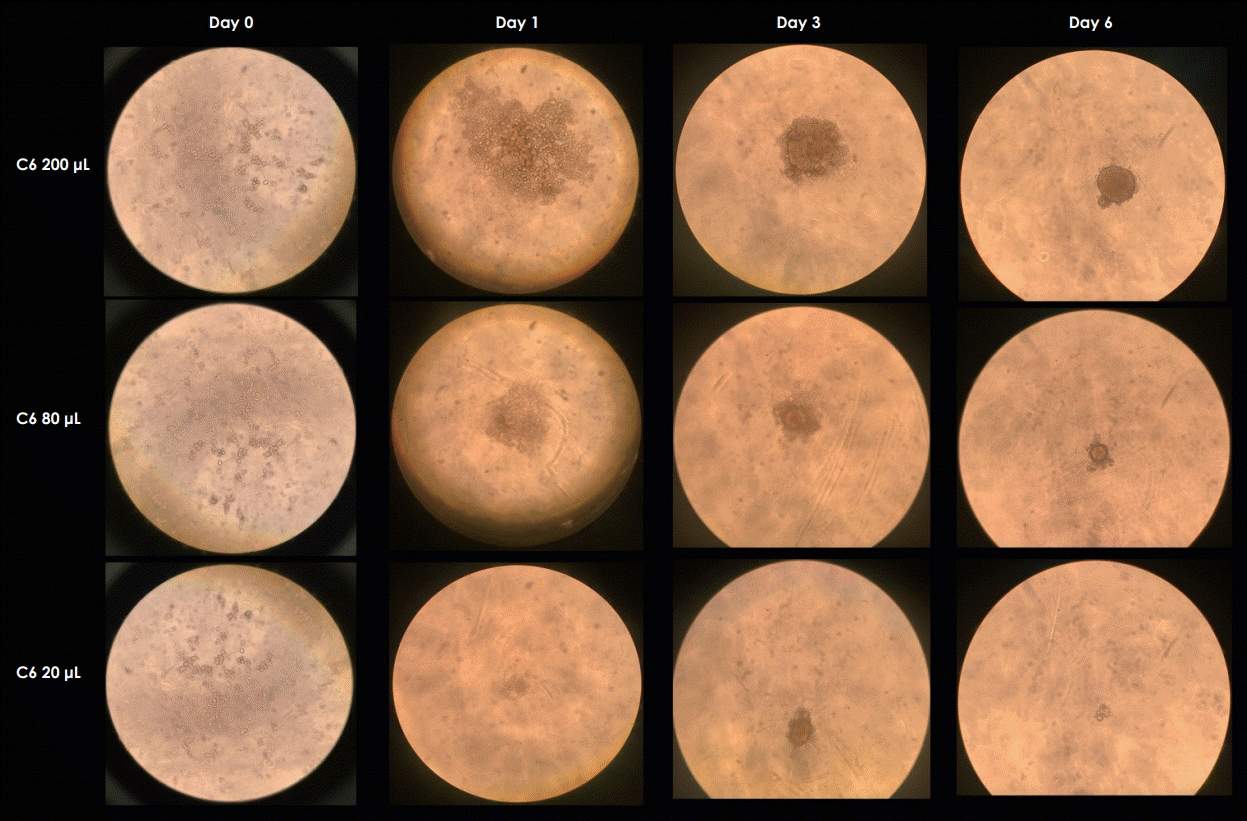
Fig. 2.
Micro-geography inside a spheroid. Spheroids show spherical structures comprise proliferating, quiescent and dead cells.
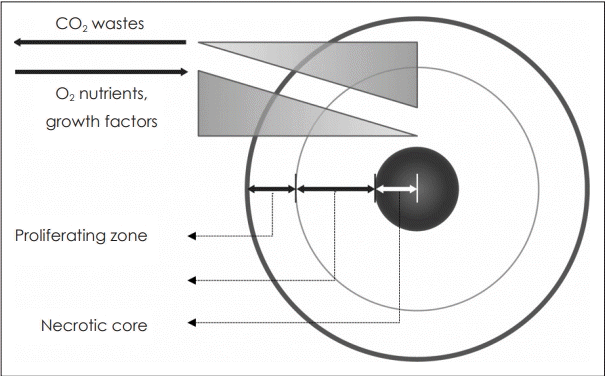
Fig. 3.
Methods for spheroid fabrication. Hanging-drop culture (A). Single cell culture on non-adhesive surface (B). Micromolding techniques (C). Spinner flask culture (D). Rotary cell culture systems (E). Hepatocyte self-assembly on Primaria dishes (F). The use of PNIPAAm based cell sheets (G). Electric, magnetic or acoustic force cell aggregation enhancement (H). PNIPAAm: poly(N-isopropylacrylamide).
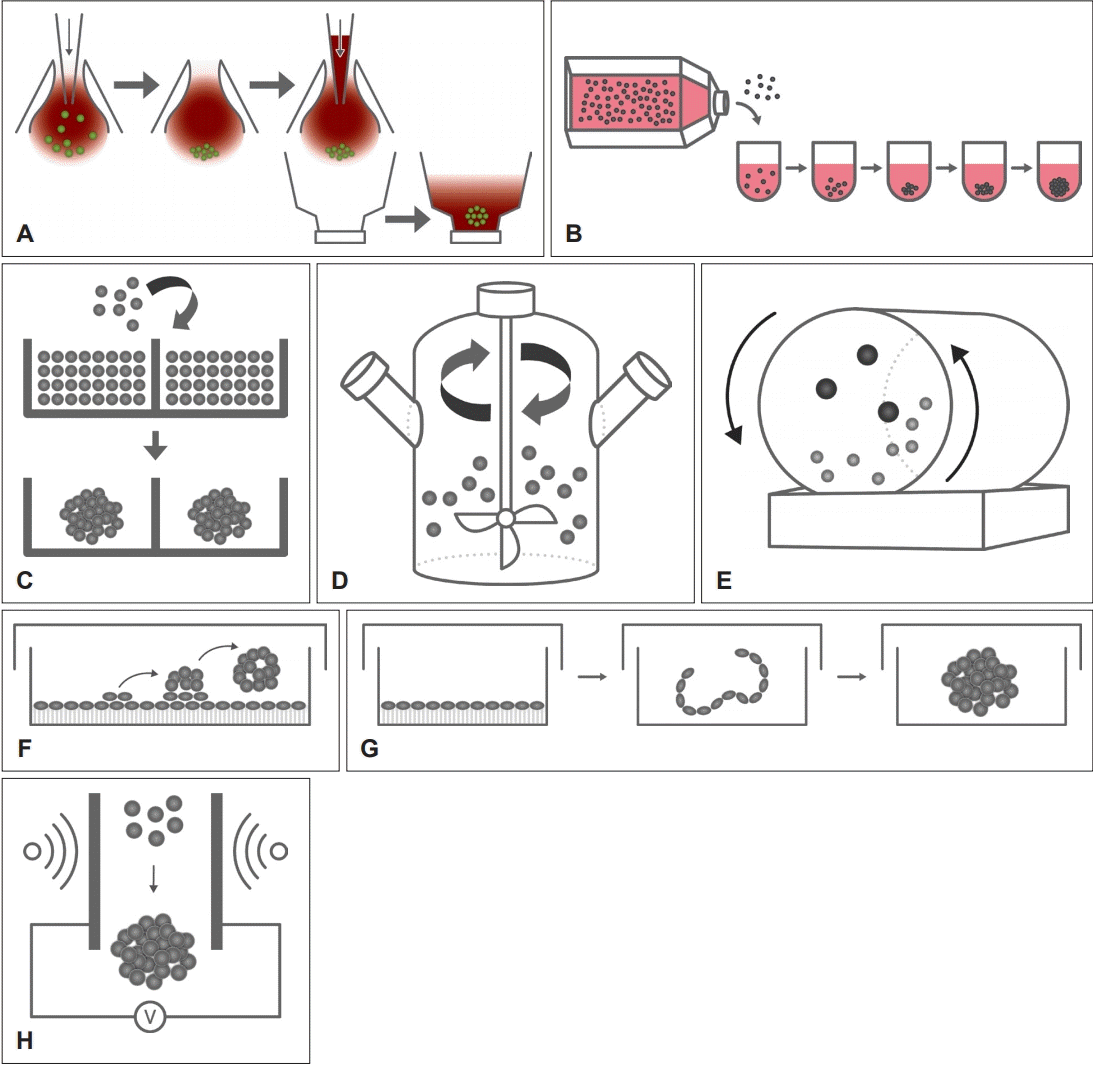
Fig. 4.
Confocal laser scanning microscopic images of spheroids from different cell concentration at 7 days after cell seeding into hanging-drop culture system. Note the necrotic central core in each spheroid.
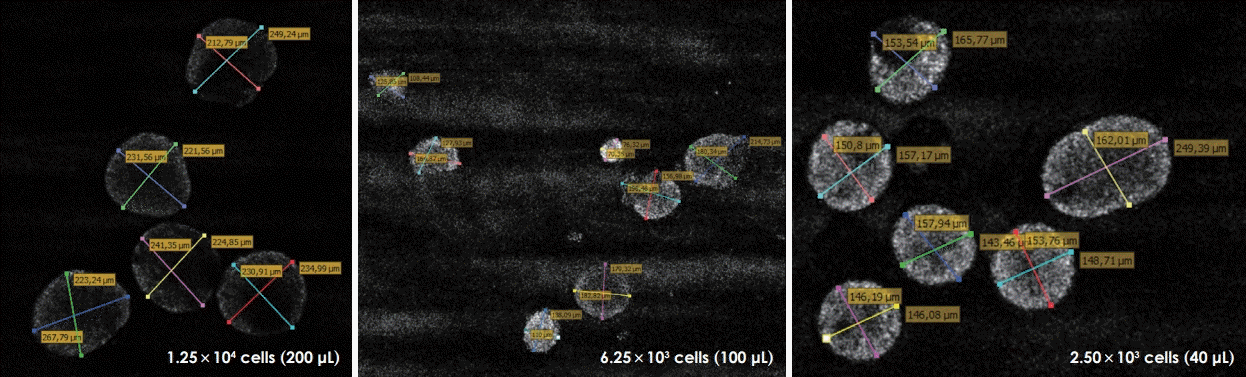
Table 1.
Spheroid fabrication methods