Abstract
Background
Methods
Results
Conclusions
ACKNOWLEDGMENTS
Notes
Author Contributions
Heyran Choi (Conceptualization; Data curation; Formal analysis; Funding acquisition; Investigation; Methodology; Project administration; Resources; Software; Supervision; Validation; Visualization; Writing – original draft; Writing – review & editing)
Sun Young Park (Conceptualization; Data curation; Formal analysis; Funding acquisition; Investigation; Methodology; Project administration; Resources; Software; Supervision; Validation; Visualization; Writing – original draft; Writing – review & editing)
Yong Beom Kim (Conceptualization; Data curation; Formal analysis; Funding acquisition; Investigation; Methodology; Project administration; Resources; Software; Supervision; Validation; Visualization; Writing – original draft; Writing – review & editing)
Junyong In (Conceptualization; Data curation; Formal analysis; Funding acquisition; Investigation; Methodology; Project administration; Resources; Software; Supervision; Validation; Visualization; Writing – original draft; Writing – review & editing)
Hong Seuk Yang (Conceptualization; Data curation; Formal analysis; Funding acquisition; Investigation; Methodology; Project administration; Resources; Software; Supervision; Validation; Visualization; Writing – original draft; Writing – review & editing)
Jeong-seok Lee (Conceptualization; Data curation; Formal analysis; Funding acquisition; Investigation; Methodology; Project administration; Resources; Software; Supervision; Validation; Visualization; Writing – original draft; Writing – review & editing)
Sanghyun Kim (Conceptualization; Data curation; Investigation; Methodology; Project administration; Validation; Visualization; Writing – original draft; Writing – review & editing)
Suyeon Park (Conceptualization; Formal analysis; Investigation; Methodology; Project administration; Software; Supervision; Validation; Visualization; Writing – review & editing)
References


















Fig. 1.
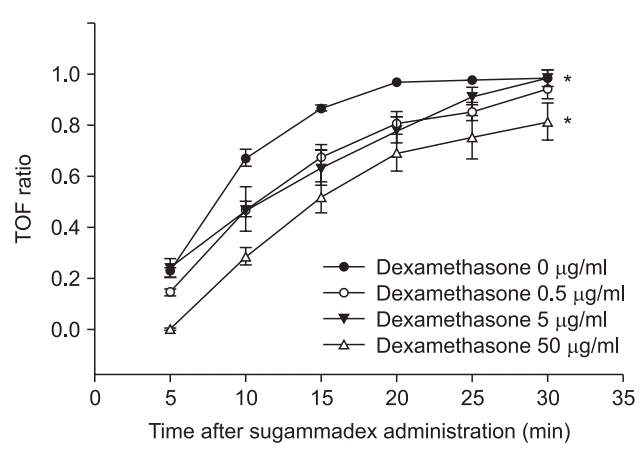
Fig. 2.
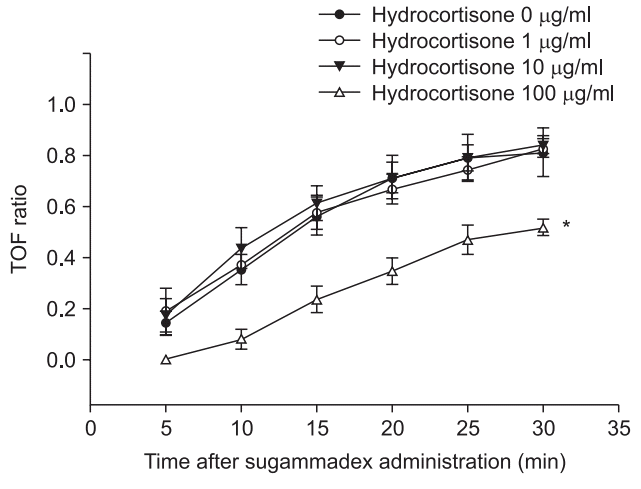
Fig. 3.
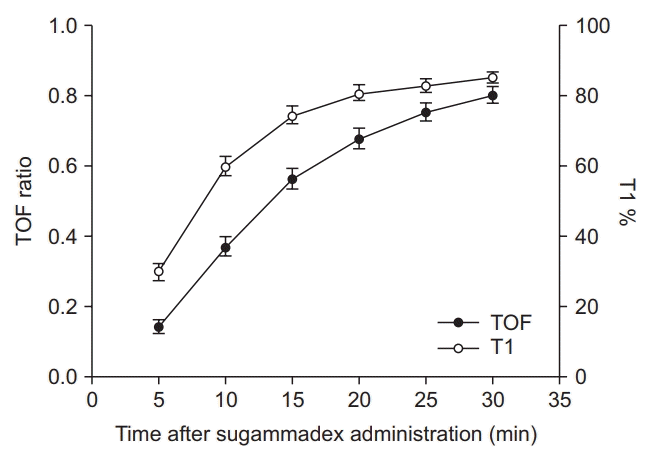
Table 1.
Table 2.
Concentration of drug | TOF ratio | T1 (%) | Recovery index (min) |
---|---|---|---|
Concentration of dexamethasone (µg/ml) | |||
0 (n = 10) | 0.98 (0.97–1.00) | 93.5 (84.8–94.3) | 7.25 (5.47–9.07) |
0.5 (n = 10) | 0.94 (0.79–0.98) | 89.6 (84.2–96.1) | 7.90 (6.70–10.30) |
5 (n = 11) | 0.97 (0.71–0.99) | 85.3 (72.4–93.0) | 7.40 (6.60–10.50) |
50 (n = 10) | 0.81 (0.53–0.87)† | 89.3 (75.3–91.8) | 10.45 (8.92–12.67) |
P | < 0.001* | 0.300 | 0.164 |
Concentration of hydrocortisone (µg/ml) | |||
0 (n = 8) | 0.93 (0.67–0.98) | 91.9 (85.0–99.7) | 6.25 (5.07–10.13) |
1 (n = 9) | 0.83 (0.71–0.94) | 87.0 (76.8–100.5) | 8.00 (5.00–12.00) |
10 (n = 12) | 0.86 (0.75–0.94) | 91.1 (83.8–96.0) | 7.43 (3.64–14.11) |
100 (n = 11) | 0.49 (0.41–0.59)†,‡,§ | 73.2 (68.4–77.7)†,‡,§ | 13.00 (10.24–16.66)† |
P | < 0.001* | < 0.001* | 0.045* |
Table 3.
Concentration × Time | Beta | SE |
95% Wald CI |
P value* | |
---|---|---|---|---|---|
Lower | Upper | ||||
Concentration of dexamethasone (mg/ml) | |||||
0 (n = 10) | Reference | ||||
0.5 (n = 10) | −0.003 | 0.002 | −0.008 | 0.001 | 0.165 |
5 (n = 11) | −0.004 | 0.001 | −0.008 | −0.001 | 0.022 |
50 (n = 10) | −0.008 | 0.003 | −0.015 | −0.002 | 0.015 |
oncentration of hydrocortisone (mg/ml) | |||||
0 (n = 8) | Reference | ||||
1 (n = 9) | −0.001 | 0.004 | −0.009 | 0.006 | 0.715 |
10 (n = 12) | 0.000 | 0.004 | −0.008 | 0.007 | 0.902 |
100 (n = 11) | −0.010 | 0.004 | −0.018 | −0.002 | 0.018 |