Abstract
Background
Cholesterol plays an important role in the action of opioid analgesics, but its association with postoperative pain has not been clarified. Our study examined the association of pre- and postoperative total serum cholesterol (TSC), and change between the pre- and postoperative TSC levels with postoperative pain outcomes in patients with non-small cell lung cancer (NSCLC) who underwent video-assisted thoracoscopic surgery (VATS) lobectomy.
Methods
We retrospectively reviewed medical records of patients with NSCLC who underwent VATS lobectomy at the Seoul National University Bundang Hospital in South Korea. We sought to determine the association between preoperative TSC, TSC on postoperative day (POD) 0–1, and pre- and postoperative changes in TSC by comparing numeric rating scale (NRS) scores on POD 0, 1, and 2 and total morphine equivalent consumption on POD 0–2. Multivariate linear regression analyses were used, and P < 0.05 was considered statistically significant.
Results
A total of 1,720 patients with NSCLC who underwent VATS lobectomy were included in the analysis. The change in TSC, preoperative TSC, and postoperative TSC showed no associations with morphine equivalent consumption on POD 0–2 (P > 0.05). In addition, the changes in TSC, preoperative TSC, and postoperative TSC were not associated with postoperative NRS pain score on POD 0, 1, and 2 (P > 0.05).
Lung cancer is one of the most common types of cancer worldwide, with non-small cell lung cancer (NSCLC) accounting for 80% of all lung cancer cases [1]. Since surgical management is the only potentially curative option for NSCLC, the majority of patients undergo resection through thoracotomy [2]. Patients who undergo thoracotomy often complain of severe postoperative pain, which sometimes progresses to chronic pain [3]. Although the recent popularity of video-assisted thoracoscopic surgery (VATS) using minimally invasive surgical techniques has led to improved pain outcomes compared to those associated with open thoracotomy, the management of postoperative pain is still an important issue in thoracic surgery.
Opioids are the analgesics of choice for pain control after thoracic surgery, and recent studies have reported that cholesterol is one crucial factor that influences the mechanisms of opioid action [4,5]. This is because μ-opioid receptors (OPRM1 or MOR) are located on the cell membrane in cholesterol-rich lipid raft microdomains that form alongside G protein-coupled receptors (GPCR) [6,7], the effects of morphine are also mediated by receptors encoded by MOR-1 [8]. The impact of cholesterol on the signaling of μ-opioid receptors has been reported through in-vitro [9] and animal studies [5]. In 2016, the first study to investigate the association between opioid dose and cholesterol levels in humans reported that the dosage of opioids used for pain control had a negative correlation with total serum cholesterol (TSC) levels in patients with advanced lung cancer [10].
On the basis of these findings, we recently conducted a retrospective analysis, in which we found no significant effect of preoperative TSC on postoperative pain outcome in patients with gastric cancer who had undergone laparoscopic surgery [11]. However, our previous study had a limitation in that we did not evaluate the relationship between TSC levels over the postoperative period and postoperative pain outcome. Since pain outcome is measured over the postoperative period, we hypothesized that postoperative TSC would correlate with postoperative pain. Thus, in this study, we aimed to explore the effects of preoperative TSC, postoperative TSC, and changes between the pre- and postoperative TSC levels on pain outcome after VATS lobectomy.
This retrospective observational study was conducted with the approval of the Institutional Review Board of Seoul National University Bundang Hospital (SNUBH) in South Korea (approval number: B-1712/436-105). We reviewed the medical records of all patients over the age of 19 years who had been diagnosed with NSCLC and had undergone elective VATS lobectomy from January 1, 2011, to July 31, 2017. In addition, we included patients who underwent sublobar resection on other ipsilateral pulmonary lobes in the ‘lobectomy’ category but excluded those who underwent sublobar resection on the lung contralateral to the one on which lobectomy had been performed. The exclusion criteria included the following: requirement for additional operations within two days after lobectomy; intraoperative conversion of the procedure to open thoracotomy; conversion of the procedure to bilobectomy or pneumonectomy; missing or inaccurate data on pain outcomes or TSC; requirement for thoracic epidural analgesia; patients who participated in randomized trials that required the intraoperative infusion of magnesium sulfate [12]; and surgery involving organs outside the thoracic cavity.
Experienced thoracic surgeons routinely perform lung lobectomy operations using the VATS method [13]. Additionally, trained anesthetists perform thoracic anesthesia using standardized anesthetic techniques. In our study, in general, remifentanil infusion was used intraoperatively for balanced anesthesia, and fentanyl-based intravenous patient-controlled analgesia was administered postoperatively. Further, additional opioids were used for pain control upon patients’ request and according to the surgeons’ judgment. Non-steroidal anti-inflammatory drugs or acetaminophen were generally not administered for 2 to 3 days after the operation.
We collected the following data: sex, age, height (cm), weight (kg), American Society of Anesthesiologists classification, Charlson Comorbidity Index score, histologic type of NSCLC, postoperative intensive care unit admission, surgery time (min), duration of hospital stay (day), pre- and postoperative TSC (mg/dl) levels, changes in TSC levels, total opioid consumption on postoperative day (POD) 0–2, and pain scores.
Preoperative cholesterol level was defined as the value obtained from the results of laboratory tests conducted at least one month before surgery, while postoperative cholesterol was the value obtained from the results of laboratory tests conducted on POD 0–1. The change in TSC was calculated by subtracting the pre- from the post-operative TSC value. To calculate total opioid consumption, all opioids used on POD 0–2 were converted as per the standard conversion ratio into oral morphine equivalent (mg) and summed (Appendix 1) [14]. Pain scores were reported using the standard 0–10 numeric rating scale (NRS). Postoperative resting NRS scores were measured at least five times daily by registered nurses. Our study defines the NRS score on a specific day as the average of all resting NRS scores on the same day. All medical records were collected by medical record technicians blinded to the purpose of this study, and the main researchers were blinded to the data until statistical outcomes were obtained.
The primary outcome measure for this study was differences in postoperative pain outcomes after VATS lobectomy according to pre- and postoperative TSC levels. For the purposes of this study, we used the values of 160 mg/dl and 200 mg/dl—levels identified as clinically significant [15,16]—as the cut-off points of TSC, and divided patients into three groups on the basis of their TSC levels (< 160: low; 160–199: medium; ≥ 200: high). We investigated differences in postoperative pain outcomes among these groups. In addition, subgroup analysis was performed to investigate the differences in the primary outcome as a function of postoperative pain intensity, by splitting the patients in the POD 0–2 groups into < 4 and ≥ 4 subgroups on the basis of average NRS pain score.
To compare the three groups of patients (low, medium, and high TSC), we conducted one-way Analysis of Variance (ANOVA) s for continuous variables and chi-square tests for categorical variables. To investigate the significant factors associated with postoperative pain outcome after VATS lobectomy of the lung, we performed multivariate linear regression analyses for morphine equivalent consumption on POD 0–2, and NRS pain score on POD 0–2. The subgroup analyses for groups with NRS pain scores < 4 and ≥ 4 within the POD 0–2 groups were performed using the same multivariate linear regression method used for morphine equivalent consumption on POD 0–2. Additionally, mean values with 95% CI of morphine equivalent consumption on POD 0–2 were presented as box plots for the preoperative and postoperative TSC groups. We considered P < 0.05 statistically significant and used IBM SPSS version 23.0 software (IBM Corp., USA) for the analyses.
A total of 1,952 patients over the age of 19 years were diagnosed with NSCLC and underwent VATS lobectomy between January 1, 2011, and July 31, 2017. Among these patients, the following cases were excluded from the analysis: 1) 86 cases with missing data on pain outcomes or on TSC; 2) 92 cases with intraoperative conversion of the procedure to open thoracotomy, bilobectomy, or pneumonectomy; 3) 38 cases of surgery involving organs outside the thoracic cavity; 4) 12 cases in which epidural analgesia was performed; and 5) 4 patients who participated in clinical trials using intraoperative magnesium sulfate infusion [12]. Thus, a total of 1,720 patients were included in the analysis. Of these patients, 849 belonged to the low TSC group, 646 to the medium TSC group, and 225 to the high TSC group, in terms of postoperative TSC levels. Table 1 compares the characteristics of these three groups.
Figs. 1A and 1B show morphine equivalent consumption as box plots for the preoperative and postoperative TSC groups, respectively. There was no significant difference between the groups according to preoperative (P = 0.532) and postoperative (P = 0.419) TSC group. Table 2 shows the results of a multivariate linear regression analysis for morphine equivalent consumption on POD 0–2 after VATS lobectomy of the lung. The changes in TSC, preoperative TSC, and postoperative TSC showed no associations with morphine equivalent consumption on POD 0–2 (P > 0.05). Age (coefficient: −0.94, 95% CI [−1.70, −0.19], P = 0.015) and surgery time (coefficient: 0.52, 95% CI [0.37, 0.67], P < 0.001) were the only factors associated with morphine equivalent consumption on POD 0–2. Table 3 shows the results of the multivariate linear regression analyses for the NRS pain score on POD 0, 1, and 2. Changes in TSC, preoperative TSC, and postoperative TSC were not associated with postoperative NRS pain score on POD 0, 1, and 2 (P > 0.05). Finally, Table 4 shows the results of a multivariate linear regression analysis for morphine equivalent consumption on POD 0–2 after VATS lobectomy of the lung in POD 0–2 groups with average NRS pain scores < 4 and ≥ 4. There was no significant association between pre- and postoperative TSC groups and morphine equivalent consumption in POD 0–2 in either group (P > 0.05).
This study was performed to explore the effects of preoperative TSC, postoperative TSC, and changes in TSC on pain outcomes after VATS lobectomy. Our results indicated that neither preoperative TSC nor postoperative TSC was associated with postoperative pain outcomes on POD 0–2. These findings may be clinically meaningful since they were derived from multivariate linear regression analyses. It is also notable that these results match with our previous findings in a study on laparoscopic surgery performed on patients with gastric cancer [11].
The results of this study may be explained as follows. First, the concept of postoperative inflammatory reaction and cholesterol homeostasis should be considered. Physical burdens or stressful conditions such as surgery are generally known to reduce TSC levels [17–19]. The human body releases cytokines over the postoperative period as a reaction to stress. This, in turn, causes the upregulation of low-density lipoprotein cholesterol, which leads to a reduction in TSC via the increase in peripheral cholesterol uptake [20]. In this study, we hypothesized that patients with lower TSC levels would require a relatively higher opioid dosage to manage the same degree of pain; this hypothesis was based on the biochemical properties of cholesterol that affect the opioid signaling pathways [4]. Although this mechanism remains controversial, the impact of lipid composition on opioid signaling is becoming an important issue for the use of analgesics in clinical practice [21].
Although TSC levels were reduced postoperatively, our findings imply that cholesterol-related receptors may not have been significantly affected by cholesterol homeostasis, which is a postoperative physiologic reaction. In addition, a previous study found that in the central nervous system, cholesterol levels are controlled by a regulatory mechanism [22]. In summary, our findings provide indirect evidence that decreased postoperative TSC probably does not affect the opioid signaling pathway. The findings of this study are concordant with those of our previous study recently conducted at the same institution [11].
The findings of our current study are clinically meaningful regarding the variable characteristics of pain resulting from the variety of surgical procedures in our previous study [11]. The postoperative pain resulting from thoracic surgery is usually more severe than the pain of abdominal surgery and is influenced by neuropathic pain caused by thoracotomy [23]. Neuropathic pain is more difficult to control by analgesics such as opioids than somatic pain [24]. The need for a chest drain tube after VATS lobectomy could also be another cause of severe pain [25]. Therefore, the results of our study—that neither pre- nor postoperative TSC affected the pain outcome after VATS thoracic surgery—are clinically important considering the different pain characteristics in this study.
Another important factor for our study is the cut-off values of pre- and postoperative TSC level. We set the cut-off values as 160 and 200 mg/dl to divide the patients into three groups according to TSC level (low, medium and high). A TSC ≥ 200 is generally defined as hypercholesterolemia, which increases the risk of mortality from coronary heart disease or stroke [26]. In contrast, TSC < 160 is generally defined as hypocholesterolemia and is associated with indicators of bad prognosis in hospitalized patients [27]. Preoperative TSC levels of 160–199 appear to be a reference range that predicts a favorable long-term prognosis, so we set the cut-off values as 160 and 200 to divide the patients into three groups.
Our study has a few limitations that should be addressed. First, the inherent drawbacks of a retrospective design may have led to biases. Second, this was a single-center study, which might cast doubt on the generalizability of the findings. Third, although the main postoperative analgesic used was fentanyl, other types of opioids were also used where necessary, which compelled us to use opioid conversion formulas. Nonetheless, our study represents the first attempt to analyze the correlation of pre- and postoperative TSC and changes in TSC with pain outcomes after VATS lobectomy.
In conclusion, this study found that neither preoperative TSC nor postoperative TSC was associated with postoperative morphine equivalent consumption on POD 0–2 and NRS pain score on POD 0, 1 and 2. These findings suggest that perioperative TSC level is not a significant factor in severe postoperative pain outcomes.
References
2. Pearson FG. Non-small cell lung cancer: role of surgery for stages I-III. Chest. 1999; 116(6 Suppl):S500–3.
3. Bayman EO, Parekh KR, Keech J, Selte A, Brennan TJ. A prospective study of chronic pain after thoracic surgery. Anesthesiology. 2017; 126:938–51.


4. Zheng H, Pearsall EA, Hurst DP, Zhang Y, Chu J, Zhou Y, et al. Palmitoylation and membrane cholesterol stabilize μ-opioid receptor homodimerization and G protein coupling. BMC Cell Biol. 2012; 13:6.


5. Zheng H, Zou H, Liu X, Chu J, Zhou Y, Loh HH, et al. Cholesterol level influences opioid signaling in cell models and analgesia in mice and humans. J Lipid Res. 2012; 53:1153–62.


6. Patel HH, Murray F, Insel PA. G-protein-coupled receptor-signaling components in membrane raft and caveolae microdomains. Handb Exp Pharmacol. 2008; (186):167–84.


7. Qiu Y, Wang Y, Law PY, Chen HZ, Loh HH. Cholesterol regulates micro-opioid receptor-induced beta-arrestin 2 translocation to membrane lipid rafts. Mol Pharmacol. 2011; 80:210–8.
8. Klein G, Rossi GC, Waxman AR, Arout C, Juni A, Inturrisi CE, et al. The contribution of MOR-1 exons 1-4 to morphine and heroin analgesia and dependence. Neurosci Lett. 2009; 457:115–9.


9. Huang P, Xu W, Yoon SI, Chen C, Chong PL, Unterwald EM, et al. Agonist treatment did not affect association of mu opioid receptors with lipid rafts and cholesterol reduction had opposite effects on the receptor-mediated signaling in rat brain and CHO cells. Brain Res. 2007; 1184:46–56.


10. Huang Z, Liang L, Li L, Xu M, Li X, Sun H, et al. Opioid doses required for pain management in lung cancer patients with different cholesterol levels: negative correlation between opioid doses and cholesterol levels. Lipids Health Dis. 2016; 15:47.


11. Oh TK, Kim HH, Park DJ, Ahn SH, Do SH, Hwang JW, et al. Association of preoperative serum total cholesterol level with postoperative pain outcomes after laparoscopic surgery for gastriccancer. Pain Pract. 2018; 18:729–35.
12. Sohn HM, Jheon SH, Nam S, Do SH. Magnesium sulphate improves pulmonary function after video-assisted thoracoscopic surgery: a randomised double-blind placebo-controlled study. Eur J Anaesthesiol. 2017; 34:508–14.
13. Kim K, Kim HK, Park JS, Chang SW, Choi YS, Kim J, et al. Video-assisted thoracic surgery lobectomy: single institutional experience with 704 cases. Ann Thorac Surg. 2010; 89:S2118–22.


14. Derby SA. Opioid conversion guidelines for managing adult cancer pain. Am J Nurs. 1999; 99:62–5.


15. Neaton JD, Blackburn H, Jacobs D, Kuller L, Lee DJ, Sherwin R, et al. Serum cholesterol level and mortality findings for men screened in the multiple risk factor intervention trial. multiple risk factor intervention trial research group. Arch Intern Med. 1992; 152:1490–500.


16. Sempos CT, Cleeman JI, Carroll MD, Johnson CL, Bachorik PS, Gordon DJ, et al. Prevalence of high blood cholesterol among US adults. An update based on guidelines from the second report of the National Cholesterol Education Program Adult Treatment Panel. JAMA. 1993; 269:3009–14.


17. Elliott DC, Wiles CE 3rd. Low lipid concentrations in critical illness: hypocholesterolemia among trauma patients. Crit Care Med. 1997; 25:1437–9.
18. Gui D, Spada PL, De Gaetano A, Pacelli F. Hypocholesterolemia and risk of death in the critically ill surgical patient. Intensive Care Med. 1996; 22:790–4.


19. Higgins TL. Quantifying risk and assessing outcome in cardiac surgery. J Cardiothorac Vasc Anesth. 1998; 12:330–40.


20. Fraunberger P, Pilz G, Cremer P, Werdan K, Walli AK. Association of serum tumor necrosis factor levels with decrease of cholesterol during septic shock. Shock. 1998; 10:359–63.


21. Marino KA, Prada-Gracia D, Provasi D, Filizola M. Impact of lipid composition and receptor conformation on the spatio-temporal organization of μ-opioid receptors in a multi-component plasma membrane model. PLoS Comput Biol. 2016; 12:e1005240.


22. Dietschy JM, Turley SD. Control of cholesterol turnover in the mouse. J Biol Chem. 2002; 277:3801–4.


23. Dualé C, Guastella V, Morand D, Cardot JM, Aublet-Cuvelier B, Mulliez A, et al. Characteristics of the neuropathy induced by thoracotomy: a 4-month follow-up study with psychophysical examination. Clin J Pain. 2011; 27:471–80.
25. Luketich JD, Kiss M, Hershey J, Urso GK, Wilson J, Bookbinder M, et al. Chest tube insertion: a prospective evaluation of pain management. Clin J Pain. 1998; 14:152–4.


Fig. 1.
Box plots for morphine equivalent consumption (mg) according to preoperative (A) and postoperative (B) TSC level. TSC: total serum cholesterol, POD: postoperative day.
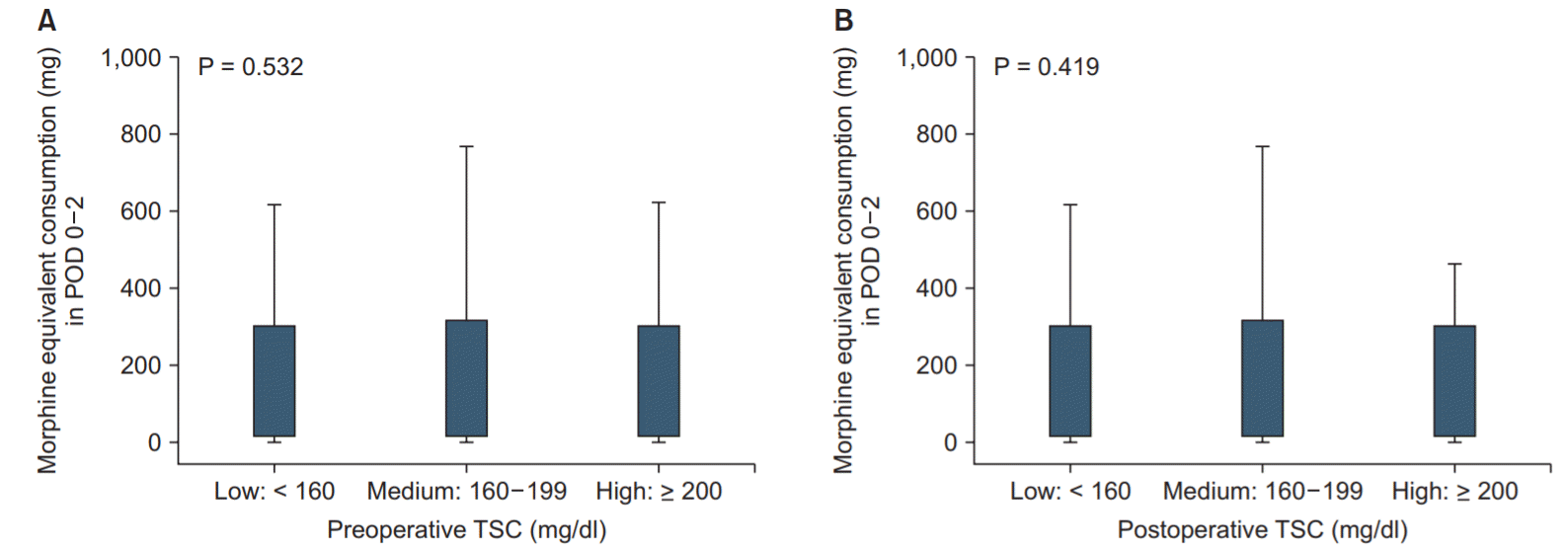
Table 1.
Demographic and Baseline Characteristics of Patients
Values are presented as mean ± SD or number (percentage). Cholesterol group: Low: < 160 mg/dl, Medium: 160–199 mg/dl, High: ≥ 200 mg/dl. TSC: total serum cholesterol, ASA: American Society of Anesthesiologists, Sqcc: squamous cell carcinoma, ICU: intensive care unit, ME: morphine equivalent, POD: postoperative day, NRS: numeric rating scale.
Table 2.
Multivariate Linear Regression Analysis for Morphine Equivalent Consumption (mg) in POD 0–2 after VATS Lobectomy of Lung
Variables | Coefficient (95% CI) | P value |
---|---|---|
Sex: F | −13.3 (−30.4, 4.0) | 0.132 |
Age | −0.9 (−1.7, −0.2) | 0.015 |
Body mass index | 1.5 (−1.2, 4.2) | 0.264 |
ASA class | ||
2 (vs 1) | 22.6 (−23.7, 68.9) | 0.339 |
3–4 (vs 1) | 15.0 (−5.9, 35.8) | 0.159 |
Charson comorbidity index (1 increase) | −0.4 (−9.3, 8.6) | 0.938 |
Duration of surgery (min) | 0.5 (0.4, 0.7) | < 0.001 |
Postoperative ICU admission (vs ward) | −17.5 (−49.5, 14.4) | 0.282 |
Change in TSC* (mg/dl) | 0.2 (−11.1, 6.5) | 0.144 |
Preoperative TSC (mg/dl) | ||
< 160 (vs: 160–199) | −7.3 (−29.5, 14.9) | 0.517 |
≥ 200 (vs: 160–199) | −10.7 (−32.1, 10.6) | 0.324 |
Postoperative TSC (mg/dl) | ||
< 160 (vs: 160–199) | −16.9 (−37.6, 3.8) | 0.110 |
≥ 200 (vs: 160–199) | −5.9 (−33.3, 21.6) | 0.676 |
Table 3.
Multivariate Linear Regression Analysis for Pain Score on POD 0, 1, and 2 after VATS Lobectomy of Lung
Variable | Coefficient (95% CI) | P value |
---|---|---|
NRS pain score on POD 0 | ||
Change in TSC* (mg/dl) | 0.0 (0.0, 0.0) | 0.125 |
Preoperative TSC (mg/dl) | ||
< 160 (vs: 160–199) | 0.1 (0.0, 0.2) | 0.254 |
≥ 200 (vs: 160–199) | 0.0 (−0.1, 0.0) | 0.183 |
Postoperative TSC (mg/dl) | ||
< 160 (vs: 160–199) | 0.0 (−0.2, 0.1) | 0.213 |
≥ 200 (vs: 160–199) | 0.0 (−0.2, 0.2) | 0.541 |
NRS pain score on POD 1 | ||
Change in TSC* (mg/dl) | 0.0 (0.0, 0.0) | 0.242 |
Preoperative TSC (mg/dl) | ||
< 160 (vs: 160–199) | 0.1 (−0.1, 0.2) | 0.284 |
≥ 200 (vs: 160–199) | −0.1 (−0.2, 0.1) | 0.233 |
Postoperative TSC (mg/dl) | ||
< 160 (vs: 160–199) | −0.1 (−0.2, 0.1) | 0.393 |
≥ 200 (vs: 160–199) | 0.0 (−0.2, 0.1) | 0.709 |
NRS pain score on POD 2 | ||
Change in TSC* (mg/dl) | 0.0 (0.0, 0.0) | 0.903 |
Preoperative TSC (mg/dl) | ||
< 160 (vs: 160–199) | 0.0 (−0.1, 0.1) | 0.560 |
≥ 200 (vs: 160–199) | 0.0 (−0.2, 0.7) | 0.486 |
Postoperative TSC (mg/dl) | ||
< 160 (vs: 160–199) | 0.0 (−0.1, 0.1) | 0.885 |
≥ 200 (vs: 160–199) | 0.0 (−0.2, 0.1) | 0.548 |
Table 4.
Multivariate Linear Regression Analysis for Morphine Equivalent Consumption (mg) on POD 0–2 after VATS Lobectomy of Lung in POD 0–2 Groups with Average NRS Pain Scores < 4 and ≥ 4
Variables |
< 4 of average NRS pain score in POD 0–2 (n = 1,459) |
≥ 4 of average NRS pain score in POD 0–2 (n = 261) |
||
---|---|---|---|---|
Coefficient (95% CI) | P value | Coefficient (95% CI) | P value | |
Sex: F | −11.2 (−31.2, 8.9) | 0.007 | −1.0 (−48.4, 46.4) | 0.967 |
Age (yr) | −0.8 (−1.7, 0.1) | 0.080 | −1.0 (−3.0, 1.1) | 0.349 |
Body mass index (kg/m2) | −0.8 (−3.8, 2.3) | 0.623 | 5.6 (−2.0, 13.2) | 0.148 |
ASA class | ||||
2 (vs 1) | 60.9 (6.1, 115.6) | 0.029 | −68.9 (−300.1, 62.2) | 0.302 |
3–4 (vs 1) | 30.0 (6.0, 54.0) | 0.014 | −21.5 (−79.8, 36.8) | 0.468 |
Duration of surgery (min) | 0.4 (0.3, 0.6) | < 0.001 | 0.9 (0.5, 1.3) | < 0.001 |
Postoperative ICU admission (vs ward) | 23.5 (−17.0, 64.1) | 0.255 | 23.1 (−168.4, 214.6) | 0.813 |
Change in TSC* (mg/dl) | 0.1 (−0.2, 0.4) | 0.527 | 1.2 (−0.2, 2.1) | 0.085 |
Preoperative TSC (mg/dl) | ||||
< 160 (vs: 160–199) | −18.0 (−43.1, 7.1) | 0.160 | 49.1 (−12.3, 110.4) | 0.117 |
≥ 200 (vs: 160–199) | 0.1 (−25.2, 25.4) | 0.993 | −29.4 (−95.1, 36.4) | 0.380 |
Postoperative TSC (mg/dl) | ||||
< 160 (vs: 160–199) | −13.9 (−37.9, 10.2) | 0.258 | −38.7 (−98.5, 21.1) | 0.203 |
≥ 200 (vs: 160–199) | 3.2 (−27.5, 33.9) | 0.839 | −18.0 (−102.0, 66.0) | 0.674 |