Abstract
Background
Methods
Results
Notes
Author Contributions
Kyungmi Kim (Data curation; Formal analysis; Investigation; Methodology; Software; Validation; Writing – original draft)
Wonyeong Jeong (Data curation; Formal analysis; Investigation; Methodology; Software; Validation; Writing – original draft)
In Gu Jun (Conceptualization; Data curation; Formal analysis; Investigation; Methodology; Software; Validation; Visualization)
Jong Yeon Park (Conceptualization; Data curation; Formal analysis; Investigation; Methodology; Project administration; Supervision; Validation)
References





























Fig. 1.
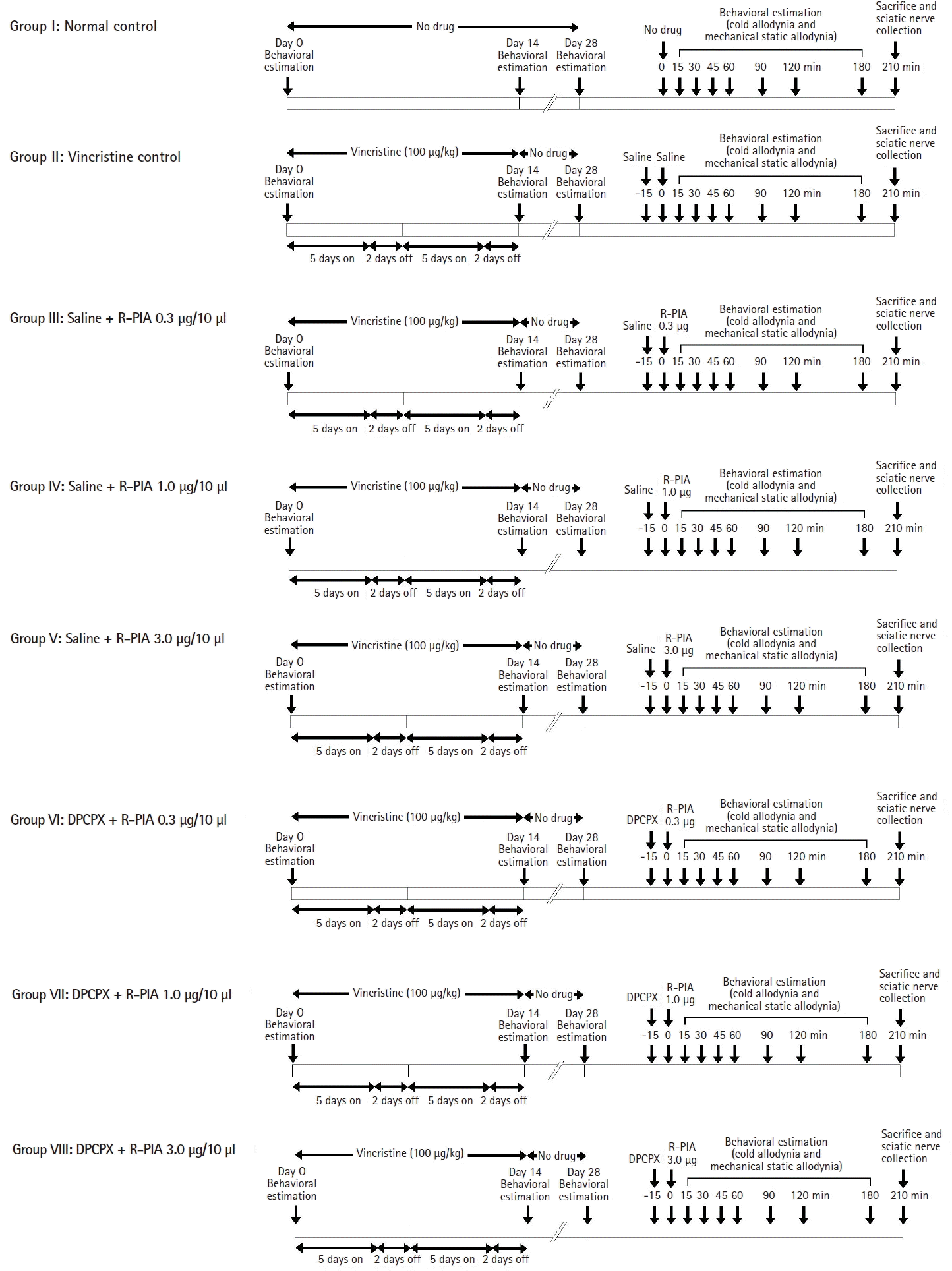
Fig. 2.
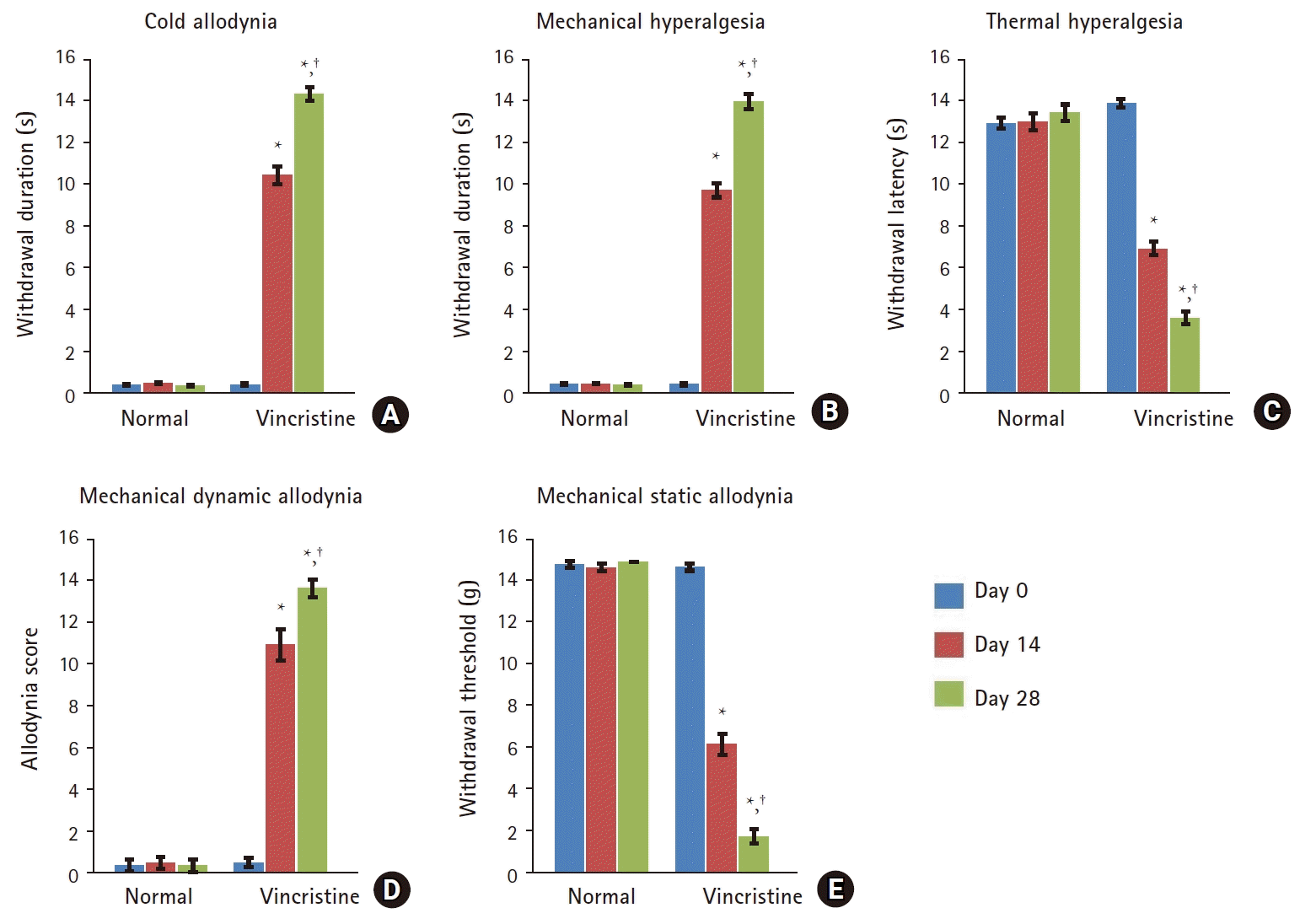
Fig. 3.
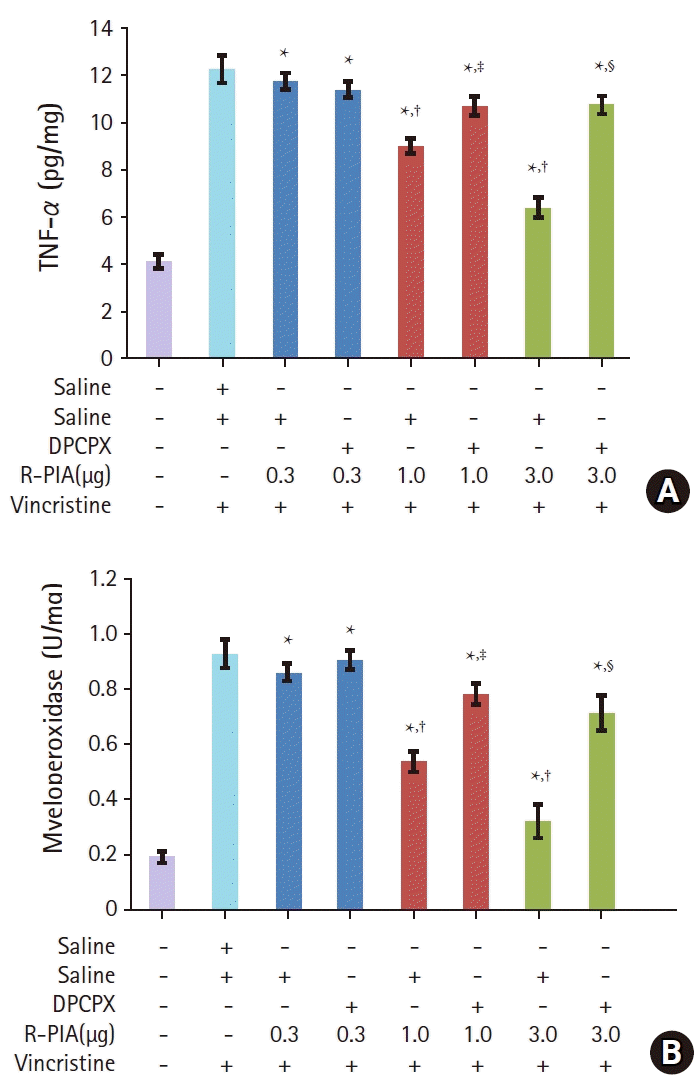
Table 1.
Time (min) | 0 | 15 | 30 | 45 | 60 | 90 | 120 | 180 |
---|---|---|---|---|---|---|---|---|
Normal | 0.5 ± 0.1 | 0.7 ± 0.1 | 0.6 ± 0.1 | 0.6 ± 0.1 | 0.5 ± 0.1 | 0.7 ± 0.1 | 0.6 ± 0.1 | 0.5 ± 0.1 |
V + S + S | 13.2 ± 0.4* | 13.4 ± 0.4* | 12.9 ± 0.4* | 12.9 ± 0.3* | 13.4 ± 0.4* | 12.9 ± 0.4* | 13.2 ± 0.4* | 13.0 ± 0.4* |
V + S + R-PIA 0.3 | 12.6 ± 0.3* | 11.7 ± 0.6* | 11.5 ± 0.4* | 11.8 ± 0.5* | 12.5 ± 0.3* | 11.6 ± 0.4* | 12.2 ± 0.4* | 12.7 ± 0.3* |
V + DPCPX + R-PIA 0.3 | 12.7 ± 0.4* | 12.6 ± 0.6* | 12.3 ± 0.5* | 12.1 ± 0.5* | 11.8 ± 0.4* | 12.4 ± 0.4* | 12.2 ± 0.4* | 12.7 ± 0.3* |
V + S + R-PIA 1.0 | 13.2 ± 0.4* | 4.2 ± 0.7*,† | 4.9 ± 0.9*,† | 6.8 ± 0.7*,† | 6.6 ± 0.4*,† | 7.7 ± 0.6*,† | 10.0 ± 0.3*,† | 11.0 ± 0.3*,† |
V + DPCPX + R-PIA 1.0 | 13.1 ± 0.3* | 11.4 ± 0.4*,‡ | 11.7 ± 0.4*,‡ | 12.2 ± 0.6*,‡ | 12.9 ± 0.2*,‡ | 12.4 ± 0.5*,‡ | 13.0 ± 0.2*,‡ | 13.3 ± 0.4* |
V + S + R-PIA 3.0 | 13.4 ± 0.4* | 0.7 ± 0.3† | 1.3 ± 0.6† | 2.0 ± 0.8† | 3.2 ± 0.8*,† | 3.7 ± 0.7*,† | 5.6 ± 0.6*,† | 6.7 ± 0.4*,† |
V + DPCPX + R-PIA 3.0 | 13.0 ± 0.4* | 11.1 ± 0.3*,§ | 10.6 ± 0.3*,§ | 11.2 ± 0.5*,§ | 11.2 ± 0.4*,§ | 11.7 ± 0.3*,§ | 12.7 ± 0.4*,§ | 12.9 ± 0.3*,§ |
Peripheral neuropathy was induced by the administration of vincristine (100 μg/kg, i.p.) for 10 days. On the 28th day, saline or DPCPX (10 μg/10 μl) was intrathecally administered 15 min before intrathecal R-PIA administration (1.0 μg/10 μl or 3.0 μg/10 μl). Cold allodynia was then assessed using the acetone drop test (seconds). Results are expressed as mean ± standard error of mean, n = 6 rats per group. One-way ANOVA followed by Tukey’s post hoc test.
Table 2.
Time (min) | 0 | 15 | 30 | 45 | 60 | 90 | 120 | 180 |
---|---|---|---|---|---|---|---|---|
Normal | 15.0 ± 0.0 | 14.9 ± 0.1 | 15.0 ± 0.0 | 14.9 ± 0.1 | 14.9 ± 0.1 | 15.0 ± 0.0 | 15.0 ± 0.0 | 14.9 ± 0.1 |
V + S + S | 1.8 ± 0.3* | 1.6 ± 0.4* | 2.2 ± 0.3* | 2.1 ± 0.4* | 1.6 ± 0.3* | 2.1 ± 0.4* | 1.8 ± 0.4* | 2.0 ± 0.4* |
V + S + R-PIA 0.3 | 2.4 ± 0.3* | 2.3 ± 0.5* | 2.5 ± 0.3* | 3.2 ± 0.3* | 3.6 ± 0.3* | 4.5 ± 0.3* | 2.8 ± 0.2* | 2.3 ± 0.2* |
V + DPCPX + R-PIA 0.3 | 2.3 ± 0.3* | 3.4 ± 0.5* | 2.7 ± 0.4* | 3.9 ± 0.4* | 4.2 ± 0.3* | 3.6 ± 0.3* | 2.8 ± 0.2* | 2.3 ± 0.2* |
V + S + R-PIA 1.0 | 1.8 ± 0.3* | 10.8 ± 0.8*,† | 10.1 ± 0.7*,† | 8.2 ± 0.5*,† | 8.4 ± 0.4*,† | 7.3 ± 0.4*,† | 5.0 ± 0.2*,† | 4.0 ± 0.2*,† |
V + DPCPX + R-PIA 1.0 | 1.9 ± 0.2* | 3.6 ± 0.4*,‡ | 3.4 ± 0.3*,‡ | 2.8 ± 0.5*,‡ | 2.1 ± 0.1*,‡ | 2.6 ± 0.4*,‡ | 2.0 ± 0.3*,‡ | 1.7 ± 0.2*,‡ |
V + S + R-PIA 3.0 | 1.6 ± 0.3* | 14.5 ± 0.3† | 13.9 ± 0.7† | 13.1 ± 0.8† | 11.9 ± 0.8*,† | 11.3 ± 0.7*,† | 9.4 ± 0.4*,† | 8.3 ± 0.4*,† |
V + DPCPX + R-PIA 3.0 | 2.0 ± 0.2* | 3.9 ± 0.3*,§ | 4.4 ± 0.3*,§ | 3.8 ± 0.4*,§ | 3.8 ± 0.3*,§ | 3.3 ± 0.3*,§ | 2.3 ± 0.2*,§ | 2.1 ± 0.2*,§ |
Peripheral neuropathy was induced by the administration of vincristine (100 μg/kg, i.p.) for 10 days. On the 28th day, saline or DPCPX (10 μg/10 μl) was intrathecally administered 15 min before intrathecal R-PIA administration (1.0 μg/10 μl or 3.0 μg/10 μl). Mechanical static allodynia was then assessed using the von Frey filament test (gram). Results are expressed as mean ± standard error of mean, n = 6 rats per group. One-way ANOVA followed by Tukey’s post hoc test.