Abstract
Background
Lipid emulsions have been used to treat various drug toxicities and for total parenteral nutrition therapy. Their usefulness has also been confirmed in patients with local anesthetic-induced cardiac toxicity. The purpose of this study was to measure the hemodynamic and composition effects of lipid emulsions and to elucidate the mechanism associated with changes in intracellular calcium levels in myocardiocytes.
Methods
We measured hemodynamic effects using a digital analysis system after Intralipid® and Lipofundin® MCT/LCT were infused into hearts hanging in a Langendorff perfusion system. We measured the effects of the lipid emulsions on intracellular calcium levels in H9c2 cells by confocal microscopy.
Results
Infusion of Lipofundin® MCT/LCT 20% (1 ml/kg) resulted in a significant increase in left ventricular systolic pressure compared to that after infusing modified Krebs-Henseleit solution (1 ml/kg) (P = 0.003, 95% confidence interval [CI], 2.4–12.5). Lipofundin® MCT/LCT 20% had a more positive inotropic effect than that of Intralipid® 20% (P = 0.009, 95% CI, 1.4–11.6). Both lipid emulsion treatments increased intracellular calcium levels. Lipofundin® MCT/LCT (0.01%) increased intracellular calcium level more than that of 0.01% Intralipid® (P < 0.05, 95% CI, 0.0–1.9).
Lipid emulsions (LE) have been used as total parenteral nutrition (TPN) [12] and as therapeutic drugs for various drug toxicities, such as psychotropic drugs (haloperidol and tricyclic antidepressants), calcium channel blockers, beta-blockers, parasiticides, or herbs (non-local anesthetic drug toxicity) [34]. Systemic local anesthetic toxicity is a rare but potentially fatal complication that is intractable to conventional cardiopulmonary resuscitation [5]. Systemic local anesthetic toxicity also has a risk of progressing to "recurrent systemic local anesthetic toxicity after successful resuscitation" [6]. Intravenous infusion of LEs reverses intractable cardiac toxicity in an animal model [78], and LEs are effective for treating local anesthetic-induced cardiac toxicity [3591011]. Therefore, various studies associated with LEs have been actively conducted to understand the mechanism of lipid rescue and improve treatment regimens. Intralipid® 20%, which contains only 100% long-chain triglycerides, is commonly used to treat local anesthetic-induced systemic toxicity [910], whereas Lipofundin® MCT/LCT 20% which is composed of 50% long-chain triglycerides and 50% mediumchain triglycerides, is occasionally used to treat local anestheticinduced systemic toxicity [11]. However, both types of LE affect hemodynamics in an ex vivo model but the associated cellular mechanism remains unknown.
Therefore, we investigated the inotropic effect of two LEs (Intralipid® or Lipofundin® MCT/LCT) on a hanging heart model mounted in a Langendorff perfusion system to elucidate the mechanism associated with changes in intracellular calcium level in myocardial cells.
Male Sprague–Dawley rats (KOATECH, Pyeongtaek, Korea; weight, 250–350 g) were used for this study. All animals were maintained in accordance with the Guidelines for the Care and Use of Laboratory Animals published by the US National Institutes of Health in 1996. This protocol was approved by the Animal Research Committee of Gyeongsang National University. Animal preparation and surgery were performed as described previously [12]. Briefly, the animals received general anesthesia with an intramuscular injection of 15 mg/kg tiletamine/zolazepam (Zoletil50; Virbac Lab., Carros, France) and 9 mg/kg of xylazine (Rompun; Bayer, Seoul, Korea). If the tail moved (i.e. a sign of awakening) during the operation, an additional 5 mg/kg tiletamine/zolazepam and 3 mg/kg xylazine were injected to maintain anesthesia. Heparin (1000 IU/kg) was administered through the femoral vein after anesthesia was induced. A tracheostomy was performed, and the animals were mechanically ventilated with room air via a 16 G catheter. The chest cavity was opened, and the heart was excised rapidly. The heart was mounted quickly on a Langendorff perfusion system and perfused with modified Krebs-Henseleit solution (118 mM NaCl, 4.7 mM KCl, 1.2 mM MgSO4, 1.2 mM KH2PO4, 2.4 mM CaCl2, 25 mM NaHCO3, 11 mM glucose, and 0.03 mM EDTA), equilibrated to pH 7.4 with a mixture of 5% CO2/95% O2. The perfusion solution was maintained at 37℃ ± 0.2℃ using thermostatically controlled water circulating system (Water Bath NTT-2200; Tokyo Rikakikai Co. Ltd., Tokyo, Japan) and a water-jacketed organ bath. Perfusion pressure was maintained at 70 mmHg with a 95 cm high fluid column and an overflow pump. The left atrium was removed and a 2F Millar catheter (Model SPR-407; Millar Instruments Inc., Houston, TX, USA) was inserted into the left ventricle. A pressure transducer (Model ML 118; ADInstruments Pty. Ltd., Sydney, Australia) was connected to a digital analysis system to measure left ventricular hemodynamic function. Left ventricular systolic pressure (LVSP), maximum rate of intraventricular pressure increase (+dP/dtmax), maximum rate of intraventricular pressure decrease (−dP/dtmax), and heart rate (HR) were measured using a computer analysis program (ChartTM5 Pro; ADInstruments) as described previously [13]. The hanging hearts were perfused with modified Krebs-Henseleit solution for 10 min to stabilize prior to recording hemodynamic function (n = 10). Hearts were excluded if the average values for the last 5 min of the stabilization period failed to meet the following criteria; LVSP, 80−130 mmHg; and HR, 200−350 beats/min. Each experimental group was injected with the drug using an infusion pump (Auto Syringe AS50 Infusion Pump; Baxter, Singapore) through a three-way stopcock on the fluid column.
The hanging hearts were assigned randomly to three groups as follows: (1) control group (hearts in which 1 ml/kg modified Krebs-Henseleit solution was infused through the three-way stopcock using an infusion pump (n = 10); (2) IL1 group (hearts in which Intralipid® 20% (1 ml/kg) was infused through the three-way stopcock using an infusion pump (n = 10); and (3) MCT1 group (hearts in which Lipofundin® MCT/LCT 20% (1 ml/kg) was infused through the three-way stopcock using an infusion pump (n = 10). The sample size calculation was based on a preliminary study. The appropriate sample size was 8 using PASS 14 software (NCSS Inc., Kaysville, UT, USA; alpha = 0.05, power = 92%). Randomization was allocated by one author with a numbered container. Hemodynamic functions at baseline and the maximum response after the LE infusion were measured.
Changes in intracellular calcium were examined by measuring increased fluorescence of fluo-3/acetoxymethyl (AM) (Molecular Probes, Eugene, OR, USA)-loaded cells with a confocal microscope (FV1000; Olympus, Tokyo, Japan). Rat embryonic ventricular myocardial H9c2 cells were purchased from the American Type Culture collection (Manassas, VA, USA) and kept in complete growth medium (Dulbecco's modified Eagle's medium; Invitrogen, Carlsbad, CA, USA) supplemented with 100% fetal bovine serum (Invitrogen) at 37℃ in a humidified atmosphere of 5% CO2. Basal and intracellular changes in calcium were examined by measuring increased fluorescence of fluo-3/AM-loaded cells in the cytosol of H9c2 cells with a confocal microscope. H9c2 cells were attached to cover slips for 24 h at 37℃ before loading with 5 µM fluo-3/AM and incubating at 37℃ for 60 min. Cover slips were inserted into a chamber mounted on the stage of the confocal microscope and rinsed with a warm normal bath solution (140 mM NaCl, 5.0 mM KCl, 1.0 mM CaCl2, 1.0 mM MgCl2, and10 mM HEPES [pH 7.4]). Baseline measurements were taken on quiescent cells for 3−4 min prior to the application of LE. Fluorescent images were collected every 0.6 sec for fast signals and analyzed by Fluoview image processing software ver. 2.1b (Olympus). Twenty signal curves were taken from one view for each and averaged after each LE treatment (n = 4). The LE concentration used was 0.01%, which induced a maximal increase in intracellular calcium in the preliminary study.
All values are expressed as mean ± standard deviation. All analyses were performed using SPSS statistical software ver. 18.0 for Windows (SPSS Inc., Chicago, IL, USA). Experimental outcomes were analyzed using one-way analysis of variance and the Bonferroni post-hoc test. A P value < 0.05 was considered significant.
Hemodynamic functions were checked at baseline and during the maximum response after LE infusion. The ratio of LVSP in the MCT1 group (110.6% ± 6.4%) increased significantly compared to that in the control group (103.2% ± 2.1%, P = 0.003, 95% confidence interval [CI], 2.4–12.5) and the IL1 group (104.1% ± 3.7%, P = 0.009, 95% CI, 1.4–11.6) (Fig. 1). The ratio of +dP/dtmax (control, 98.5% ± 6.9%; IL1, 103.2% ± 7.2%; MCT1, 108.7% ± 15.7%), −dP/dtmax (control, 97.6% ± 10.0%; IL1, 103.9% ± 6.0%; MCT1, 102.1% ± 9.6%), and HR (control, 93.2% ± 7.8%; IL1, 96.6% ± 1.5%; MCT1, 94.0% ± 5.0%) were not significant between the groups.
LE-induced changes in intracellular calcium were detected by confocal microscopy. The change of intracellular calcium level was estimated by comparing the cellular fluorescence ratio with the basal fluorescence ratio using the fluo-3/AM total calcium imaging technique. Our results show that the LE treatments increased intracellular calcium level (Fig. 2). Lipofundin® MCT/LCT (0.01%) increased intracellular calcium levels more than that of Intralipid® (0.01%) (IL group: 3.03 ± 0.53; MCT group: 3.92 ± 0.60; P < 0.05; 95% CI, 0.0–1.9).
The major findings of our study are: 1) the Lipofundin® MCT/LCT infusion significantly increased LVSP in hanging hearts, 2) Lipofundin® MCT/LCT has had more of a positive inotropic effect than that of Intralipid®, and 3) the LE treatments increased the intracellular calcium levels in H9c2 cells. Lipofundin® MCT/LCT increased intracellular calcium levels more than that of Intralipid®.
Although there are some reports indicate that long-chain triglycerides has have virtually no effect on hemodynamics [14], it is a generally accepted that LE has a positive hemodynamic effect [1516]. However, a mixture of medium-chain triglycerides, long-chain triglycerides, and omega-3 polyunsaturated fatty acid emulsions increase mean aortic blood pressure [17]. We hypothesized that the hemodynamic effects of LEs may be dependent on their components. Thus, we investigated the effect of commercially available LEs (Intralipid® 20% and Lipofundin® MCT/LCT 20%) on hemodynamics in an ex vivo heart model. As results, Lipofundin® MCT/LCT had a more positive inotropic effect than that of Intralipid®. Therefore, careful observations of hemodynamic changes are needed during bolus infusion of LEs. In particular, Lipofundin® MCT/LCT should be used with caution as a total parenteral nutrition component in critically ill patients with compromised cardiovascular function.
The second aim of this study was to elucidate the mechanism responsible for the LE-induced increase in LVSP and intracellular calcium levels in myocardial cells. Long chain fatty acids directly activate calcium channels at some lipid sites near the channels or on the channel protein itself, as assessed by the standard whole cell voltage clamp technique in ventricular myocytes [18]. Therefore, the positive inotropic effect of Lipofundin® MCT/LCT shown on the hearts connected to the Langendorff perfusion system may be associated with cardiac myocyte calcium concentration. However, one study showed that 10% intralipid exerts a modest positive inotropic effect, although the intracellular calcium remains unchanged by Intralipid® [19]. Thus, we measured the change in calcium current after adding LEs to rat cardiac myoblastic H9c2 cells to clarify their effect on calcium current. Most of the signal transduction pathways that stimulate inotropy ultimately involve calcium either by increasing calcium influx, by increasing release of calcium from the sarcoplasmic reticulum, or by sensitizing troponin-C to calcium [20]. In this study, we found that LEs increased intracellular calcium level more than three times in H9c2 cells, and that Lipofundin® MCT/LCT increased intracellular calcium level more than that of Intralipid®, suggesting that Lipofundin® MCT/LCT has a more positive inotropic effect than that of Intralipid®.
LEs are used for various clinical purposes [12345] and have become an integral part of parenteral nutrition [12], effective treatment for local anesthetic-induced cardiovascular collapse [5], and treatment for lipophilic drug toxicity [34]. The mechanism of action of LEs for treating local anesthetic-induced cardiac toxicity is not completely understood. Moreover, it is unknown which LE is more effective for treating this condition. However, local anesthetic-induced systemic toxicity is caused by inhibiting inotropic and metabotropic cell signal systems and possibly mitochondrial metabolism [8]. The "lipid sink theory" [48] (reduced tissue binding by re-establishing equilibrium in the plasma lipid phase) and the "energetic-metabolic effect" [421] (reversal of inhibited mitochondrial fatty acid transport) are the most widely known theories for the mechanism of action of LEs for treating local anesthetic-induced systemic toxicity. In addition, a "positive inotropic effect" is proposed as another mechanism [9]. In this regard, Lipofundin® MCT/LCT seems to be a more effective LE for treating this condition than Intralipid®.
Our study had some limitations. First, the myoblastic H9c2 cell line was selected for this study. We used these cells because they are derived from rat heart. However, these cells retain many skeletal muscle properties [22]. Thus, primary rat heart culture would have been more reasonable for this research. However, it is technically challenging to obtain viable cultured myocytes. Second, we found that the LEs increase intracellular calcium levels. However, we did not verify the source of the increase or the channel involved. Thus, further research regarding the effect of calcium channel blockers on LE-induced increase in calcium is needed to elucidate the calcium source. Third, we showed previously that 3 ml/kg Intralipid® increases LVSP in an in vivo rat model [23]. However, as this large volume of LE could change the hemodynamics in an ex vivo heart model, we chose 1 ml/kg in this study [24]. We assume that this may have affected the limited increase in LVSP after the Intralipid® treatment despite the increased intracellular calcium level. In conclusion, LEs had positive inotropic effects in a perfused heart model. Particularly, Lipofundin® MCT/LCT had more of a positive inotropic effect than that of Intralipid®. The inotropic effect of LEs may be related to increased intracellular calcium levels in the heart.
Acknowledgments
Il-Woo Shin currently receiving a grant (NRF-2011-0021216) from Nation Research Foundation of Korea (NRF). This Work was supported by Development Fund Foundation, Gyeongsang National University, 2015. Other authors declare that they have no conflict of interests to disclose concerning the contents of the paper.
References
1. Warshawsky KY. Intravenous fat emulsions in clinical practice. Nutr Clin Pract. 1992; 7:187–196. PMID: 1294888.


2. Chambrier C, Bannier E, Lauverjat M, Drai J, Bryssine S, Boulétreau P. Replacement of long-chain triglyceride with medium-chain triglyceride/long-chain triglyceride lipid emulsion in patients receiving long-term parenteral nutrition: effects on essential fatty acid status and plasma vitamin K1 levels. JPEN J Parenter Enteral Nutr. 2004; 28:7–12. PMID: 14763787.


3. Cave G, Harvey M, Graudins A. Intravenous lipid emulsion as antidote: a summary of published human experience. Emerg Med Australas. 2011; 23:123–141. PMID: 21489160.
4. Rothschild L, Bern S, Oswald S, Weinberg G. Intravenous lipid emulsion in clinical toxicology. Scand J Trauma Resusc Emerg Med. 2010; 18:51. PMID: 20923546.


5. Dix SK, Rosner GF, Nayar M, Harris JJ, Guglin ME, Winterfield JR, et al. Intractable cardiac arrest due to lidocaine toxicity successfully resuscitated with lipid emulsion. Crit Care Med. 2011; 39:872–874. PMID: 21263316.


6. Marwick PC, Levin AI, Coetzee AR. Recurrence of cardiotoxicity after lipid rescue from bupivacaine-induced cardiac arrest. Anesth Analg. 2009; 108:1344–1346. PMID: 19299810.


7. Zausig YA, Zink W, Keil M, Sinner B, Barwing J, Wiese CH, et al. Lipid emulsion improves recovery from bupivacaine-induced cardiac arrest, but not from ropivacaine- or mepivacaine-induced cardiac arrest. Anesth Analg. 2009; 109:1323–1326. PMID: 19762764.


8. Weinberg G. Lipid rescue resuscitation from local anaesthetic cardiac toxicity. Toxicol Rev. 2006; 25:139–145. PMID: 17192120.


9. Ozcan MS, Weinberg G. Update on the use of lipid emulsions in local anesthetic systemic toxicity: a focus on differential efficacy and lipid emulsion as part of advanced cardiac life support. Int Anesthesiol Clin. 2011; 49:91–103. PMID: 21956080.
10. Warren JA, Thoma RB, Georgescu A, Shah SJ. Intravenous lipid infusion in the successful resuscitation of local anesthetic-induced cardiovascular collapse after supraclavicular brachial plexus block. Anesth Analg. 2008; 106:1578–1580. PMID: 18420881.


11. Charbonneau H, Marcou TA, Mazoit JX, Zetlaoui PJ, Benhamou D. Early use of lipid emulsion to treat incipient mepivacaine intoxication. Reg Anesth Pain Med. 2009; 34:277–278. PMID: 19436188.


12. Jang IS, Park MY, Shin IW, Sohn JT, Lee HK, Chung YK. Ethyl pyruvate has anti-inflammatory and delayed myocardial protective effects after regional ischemia/reperfusion injury. Yonsei Med J. 2010; 51:838–844. PMID: 20879048.


13. Shin IW, Jang IS, Lee SM, Park KE, Ok SH, Sohn JT, et al. Myocardial protective effect by ulinastatin via an anti-inflammatory response after regional ischemia/reperfusion injury in an in vivo rat heart model. Korean J Anesthesiol. 2011; 61:499–505. PMID: 22220228.


14. Mjos OD. Effect of free fatty acids on myocardial function and oxygen consumption in intact dogs. J Clin Invest. 1971; 50:1386–1389. PMID: 5090055.
15. Stojiljkovic MP, Zhang D, Lopes HF, Lee CG, Goodfriend TL, Egan BM. Hemodynamic effects of lipids in humans. Am J Physiol Regul Integr Comp Physiol. 2001; 280:R1674–R1679. PMID: 11353670.


16. Umpierrez GE, Smiley D, Robalino G, Peng L, Kitabchi AE, Khan B, et al. Intravenous intralipid-induced blood pressure elevation and endothelial dysfunction in obese African-Americans with type 2 diabetes. J Clin Endocrinol Metab. 2009; 94:609–614. PMID: 19001516.


17. Van de Velde M, Wouters PF, Rolf N, Van Aken H, Vandermeersch E. Comparative hemodynamic effects of three different parenterally administered lipid emulsions in conscious dogs. Crit Care Med. 1998; 26:132–137. PMID: 9428555.


18. Huang JM, Xian H, Bacaner M. Long-chain fatty acids activate calcium channels in ventricular myocytes. Proc Natl Acad Sci U S A. 1992; 89:6452–6456. PMID: 1321440.


19. Cook DJ, Housmans PR. Mechanism of the negative inotropic effect of propofol in isolated ferret ventricular myocardium. Anesthesiology. 1994; 80:859–871. PMID: 8024141.


20. George CH, Parthimos D, Silvester NC. A network-oriented perspective on cardiac calcium signaling. Am J Physiol Cell Physiol. 2012; 303:C897–C910. PMID: 22843795.


21. Stehr SN, Ziegeler JC, Pexa A, Oertel R, Deussen A, Koch T, et al. The effects of lipid infusion on myocardial function and bioenergetics in l-bupivacaine toxicity in the isolated rat heart. Anesth Analg. 2007; 104:186–192. PMID: 17179268.


22. Kimes BW, Brandt BL. Properties of a clonal muscle cell line from rat heart. Exp Cell Res. 1976; 98:367–381. PMID: 943302.


23. Shin IW, Hah YS, Kim C, Park J, Shin H, Park KE, et al. Systemic blockage of nitric oxide synthase by L-NAME increases left ventricular systolic pressure, which is not augmented further by Intralipid®. Int J Biol Sci. 2014; 10:367–376. PMID: 24719554.


24. Grieve DJ, Cave AC, Byrne JA, Layland J, Shah AM. Analysis of ex vivo left ventricular pressure-volume relations in the isolated murine ejecting heart. Exp Physiol. 2004; 89:573–582. PMID: 15184354.
Fig. 1
Effects of lipid emulsions (Lipofundin® MCT/LCT 20% and Intralipid® 20%) and modified Kreb-Henseleit solution on left ventricular systolic pressure (LVST), ± dP/dtmax, and heart rate (HR) in hanging heart in a Langendorff perfusion system (parameters are shown as a ratio before and after infusing the lipid emulsion). Control group: modified Krebs-Henseleit solution (1 ml/kg) was infused through the three-way stop cock using an infusion pump, IL1 group: Intralipid® 20% (1 ml/kg) was infused; and MCT1 group: Lipofundin® MCT/LCT 20% (1 ml/kg) was infused. Values are means ± standard deviations (n = 10/group). N indicates number of hearts. *P < 0.05 compared to control group. †P < 0.05 compared to IL1 group.
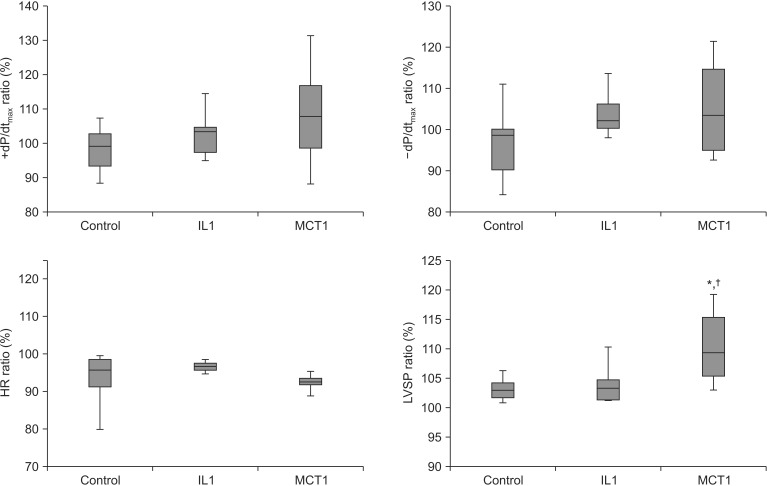
Fig. 2
Effect of lipid emulsions (0.01% Intralipid® and 0.01% Lipofundin® MCT/LCT) on changes in intracellular calcium levels as detected by confocal microscopy. Values are expressed as the ratio of the baseline value before administration of the lipid emulsion and are means ± standard deviations (n = 4/group). N indicates the number of independent experiments. *P < 0.001 compared with baseline. †P < 0.05 compared with 0.01% Intralipid®.
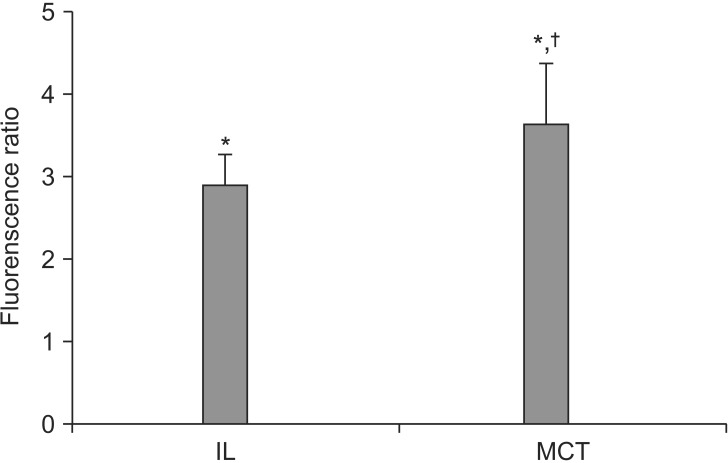