Abstract
Background
Minimal-flow anesthesia can meet the demands of a modern society that is more sensitive to environmental protection and economic burdens. This study compared the safety and efficacy of minimal-flow desflurane anesthesia with conventional high-flow desflurane anesthesia for prolonged laparoscopic surgery.
Methods
Forty-six male patients (ASA physical status II or III) undergoing laparoscopic urologic surgery for more than 6 hours were randomly divided into two groups: the high-flow (HF) group and the minimal-flow (MF) group. The HF group was continuously administered a fresh gas flow of 4 L/min. In the MF group, a fresh gas flow of 4 L/min was administered for the first 20 minutes and was thereafter lowered to 0.5 L/min. Inspiratory and expiratory desflurane concentrations, respiratory variables, and hemodynamic variables were continuously monitored during administration of anesthesia. Measurements of carboxyhemoglobin (COHb) concentration and arterial blood gas analysis were performed every 2 hours during anesthesia. Serum aspartate aminotransferase (AST), alanine aminotransferase (ALT), blood urea nitrogen (BUN) and creatinine were measured on the first and second day after the surgery.
Results
Demographic data and duration of anesthesia were not different between the two groups. Significant differences were not observed between the two groups in terms of hemodynamic variables, respiratory variables, and inspiratory and expiratory desflurane concentrations. Inspiratory O2 concentration was maintained lower in the MF group than in the HF group (43-53% vs. 53-59%; P < 0.05). Compared with the HF group, COHb concentrations was higher (P < 0.05), but not increased from the baseline value in the MF group. Serum AST, ALT, BUN, and creatinine were not significantly different between the two groups.
Low-flow anesthesia (fresh gas flow less than 1 L/min) can be used to heat and humidify inspired gases, provide cost benefit and reduce atmospheric pollution. However, many anesthesiologists still commonly use high-flow anesthesia because of a long history of practicing high-flow anesthesia and a fear of side effects from low-flow anesthesia. The reduction of fresh gas flow (FGF) to 500 ml/min (known as minimal-flow anesthesia) would provide more advantages of low-flow anesthesia.
Several authors have reported safe use of prolonged minimal-flow anesthesia for non-laparoscopic surgery [1-3]. Though the concentration of inspired oxygen is gradually decreased during minimal-flow anesthesia, adequate oxygenation is maintained especially on current anesthesia machine [4]. However, there have been no studies on the disparity of delivered and inspired oxygen concentration during prolonged minimal-flow anesthesia for laparoscopic surgery.
Desflurane contains a difluoromethoxy group (F2HCO-) and produces carbon monoxide (CO) when reacting with desiccated CO2 absorbents [5-7]. Various factors such as inhaled anesthetics, duration of anesthesia, amount of FGF, intra-abdominal CO2 insufflation, and smoke absorption from tissue combustion may influence the production of CO and carboxyhemoglobin (COHb) during laparoscopic surgery.
The purpose of this study was to determine the safety and efficacy of minimal-flow desflurane anesthesia for prolonged laparoscopic surgery. Therefore, the effects of minimal-flow desflurane anesthesia were compared with those of high-flow (FGF of 4.0 L/min) desflurane anesthesia by evaluating hemodynamic and respiratory parameters, COHb and postoperative hepatic and renal function in patients who underwent prolonged laparoscopic surgeries.
Fifty male patients (American Society of Anesthesiologists physical status II or III) who were undergoing robotic laparoscopic surgery of more than 6 hours duration participated in this study. Informed consent was obtained from each patient. Patients with abnormal hepatic, renal, or cardiopulmonary function; central nervous system disease; or a history of alcohol or recreational drug abuse or chronic drug use were excluded from the study.
The patients were premedicated with 0.004 mg/kg of glycopyrrolate and 20 mg of famotidine before induction of anesthesia. For every case, fresh soda lime (Spherasorb®, Intersurgical, England) was used with the same anesthesia machine (Primus Dräger-werk, Lübeck, Germany). Anesthesia was induced with fentanyl 1.0 µg/kg and propofol 2.0 mg/kg. Rocuronium 0.6 mg/kg was administered to facilitate tracheal intubation.
The minimal-flow (MF) group was first ventilated with a FGF of 4 L/min (FiO2 0.5 with a mixture of O2 and air) using desflurane (6.0-8.0 vol%). After 20 min, FGF was reduced to 0.5 L/min (FiO2 0.5 with a mixture of O2 and air) for the duration of the surgical procedure. The high-flow (HF) group was continuously ventilated with a FGF of 4 L/min (FiO2 0.5 with a mixture of O2 and air) using desflurane (6.0-8.0 vol%). The desflurane concentration was adjusted to maintain heart rate and blood pressure within ± 20% of baseline values and to achieve a Bispectral index (BIS) of 40-50. Vecuronium was used for muscle relaxation during the surgeries. When hypotension (systolic blood pressure < 90 mmHg) occurred, volume replacement was first conducted, followed by the administration of 10 mg of ephedrine or 100 µg of phenylephrine. Bradycardia (heart rate < 45 beats/min) was treated with 0.5 mg of atropine. Mechanical ventilation was initiated with a tidal volume of 8 ml/kg, and the ventilatory rate was adjusted to maintain end-tidal CO2 tension of 30-40 mmHg.
BIS, temperature, oxygen saturation, end-tidal CO2 tension, inspiratory O2 concentration, inspiratory and expiratory desflurane concentrations, tidal volume, and airway pressure were continuously monitored during anesthesia. Arterial blood gas analysis was performed and COHb concentration measured before anesthesia, every 2 hours during anesthesia, and after the patient recovered from anesthesia. The COHb concentration was measured with cooximeter (Rapidlab 1265®, Siemens, USA), with which the measurement and normal range of COHb concentration was 0.0-100.0% and 0.0-1.5%, respectively. At the end of the surgery, residual neuromuscular block was antagonized with pyridostigmine 0.25 mg/kg and glycopyrrolate 0.005 mg/kg, and desflurane was discontinued without tapering. The time to eye opening from the discontinuation of desflurane was recorded. Venous blood samples were analyzed for levels of aspartate aminotransferase (AST), alanine aminotransferase (ALT), blood urea nitrogen (BUN), and creatinine the day before surgery and on days 1 and 2 after surgery.
Statistical analyses were performed using SPSS software (Version 18.0, SPSS Inc., Chicago, IL, USA). All values are expressed as the mean ± SD. Differences between the groups were compared using an independent t-test. Changes between time points within each group were compared using repeated measures of ANOVA with post hoc comparison using Bonferroni's correction. P values less than 0.05 were considered statistically significant.
Fifty male patients were recruited into the study. However, 2 patients in each group were excluded because of an operation time of less than 4 hours; thus, 46 patients were available for complete evaluation. No significant differences were observed in the demographic, clinical, and anesthetic data between the two groups (Table 1).
The changes in heart rate, systolic blood pressure, and diastolic blood pressure during laparoscopic surgery are shown in Fig. 1. These hemodynamic parameters were maintained within ± 20% of baseline and were not significantly different between the groups during the study period.
The changes in desflurane concentrations are shown in Fig. 2. The inspiratory desflurane concentration was maintained at 5.0-6.5% in the HF group and at 5.3-6.0% in the MF group. The expiratory desflurane concentration was maintained at 4.7-6.0% in the HF group and at 4.8-5.7% in the MF group. Inspiratory and expiratory desflurane concentration did not differ significantly between the groups during the study period.
The changes in inspiratory oxygen concentrations are shown in Fig. 3. Inspiratory O2 concentration was maintained at 53-59% in the HF group and at 43-53% in the MF group. At 2, 4, and 6 hours after induction, PaO2 was significantly lower in the MF group than in the HF group (P < 0.05). In contrast, pH, PaCO2 and HCO3- were not different between the groups (Table 2).
The COHb concentration was significantly higher in the MF group than in the HF group (P < 0.05). However, the COHb concentration was not increased at 2, 4, or 6 hours after induction or in the recovery room in the MF group (Fig. 4). The COHb concentration did not reach values in excess of 1.5% in any patient in either group. The COHb concentration was not different between smoker and nonsmoker in each group.
The changes in postoperative hepatic and renal function tests are summarized in Table 3. At 1 day (POD1) and 2 days (POD2) after surgery, AST values showed significant increase over preoperative values in both groups (P < 0.05). However, significant differences were not observed in AST, ALT, BUN, and creatinine between the two groups throughout the study period.
This study was the first report concerning minimal-flow desflurane anesthesia during prolonged surgery, and intended to show the safety and efficacy of minimal-flow desflurane anesthesia during prolonged laparoscopic surgery.
Minimal-flow anesthesia has many advantages for inhalation anesthesia [8], including low cost and reduction in global warming and air pollution. Currently available volatile anesthetics such as sevoflurane, desflurane, and isoflurane undergo very little in vivo metabolism during clinical use [9,10]. These anesthetics are exhaled and scavenged by anesthesia machines with little or no additional degradation [11,12] and are usually vented out of the building as medical waste gases. Most of the organic anesthetic gases remain for a long time in the atmosphere where they have the potential to act as greenhouse gases. The published atmospheric lifetimes for sevoflurane and desflurane range between 1.4 and 21.4 years [13,14]. Ryan and Nielsen [15] found that desflurane has a greater potential impact on global warming than either sevoflurane or isoflurane. Furthermore, their calculations indicate that avoiding N2O and unnecessarily high FGF rates can reduce the environmental impact of volatile anesthetics. Thus, the lower possible FGF rate would be the better choice for the environment because it would minimize anesthetic use. Therefore, the design of this study included only desflurane with an FGF of 0.5 L/min, without N2O.
Various factors such as inhaled anesthetics, duration of anesthesia, amount of FGF, intra-abdominal CO2 insufflation, and smoke absorption from tissue combustion may influence the production of CO during laparoscopic surgery. Fang et al. [5] found that the difluoromethoxy group (F2HCO-) of anesthetics, including desflurane, isoflurane, and sevoflurane, produce CO by reacting with CO2 absorbents.
CO concentrations have been correlated with COHb levels [16]. Dry CO absorbents has produced high level of CO with high CO concentrations in swine during desfluane anesthesia [17]. The amount of CO produced could be estimated by the COHb concentration during inhalational anesthesia.
Soro et al. [18] assumed that significant increases in COHb are not produced during laparoscopic surgery, even under closed-system anesthesia without pulmonary CO elimination. This is most likely due to a low peritoneal absorption of CO. They concluded that CO intoxication is not produced if reasonable periods of electrocautery are used and the pneumoperitoneum gas is regularly renewed in adult patients. Baum et al. [19] reported that no patient has shown any evidence or clinical signs of CO intoxication. Furthermore, Strauss et al. [20] reported that there is only a 0.4% mean increase in COHb during 6 hours of closed-system anesthesia.
This study showed a lower PaO2 in the MF anesthesia group than in the HF anesthesia group. Although this value was slightly lower in minimal-flow anesthesia, this oxygen concentration level was not harmful to patients. As a result, it was a significant difference in PaO2 as well. However, this was within the safe range of oxygen pressure for patients.
Postoperative hepatic and renal function test results did not show a significant difference between the MF and HF groups, and results of both groups were within the normal range. Similarly, results of postoperative hepatic and renal function tests were not significantly different in a minimal-flow sevoflurane anesthesia study [3].
The subjects of this study were limited to male patients with normal hepatic and renal functions and a prolonged urologic surgery. The effect of minimal-flow anesthesia on hemodynamic and respiratory variables was examined under the following conditions: the generation of CO2 by laparoscopy, prolonged surgery, and desflurane anesthesia using soda lime as an absorbent. However, further studies are necessary in a variety of subjects undergoing diverse surgeries to confirm that this method can be freely and safely used for patients regardless of gender, type of surgery, or the duration of anesthesia.
In conclusion, in prolonged laparoscopic surgery, no significant differences were found in safety and efficacy between minimal-flow and high-flow desflurane anesthesia.
References
1. Lee JK, Um SY, Chung CJ, Chin YJ. Comparison of enflurane consumptions and costs in low-flow and high-flow anesthesia. Korean J Anesthesiol. 1999; 37:574–579.
2. Chung CJ, Ko DK, Lee HJ, Lee SI. Clinical evaluation of low flow enflurane anesthesia in infants. Korean J Anesthesiol. 2000; 39:523–527.
3. Choi SR, Cho WJ, Chin YJ, Chung CJ. The effects of prolonged minimal-flow sevoflurane anesthesia on postoperative hepatic and renal function. Korean J Anesthesiol. 2008; 54:501–506.


4. Virtue RW. Minimal flow nitrous anesthesia. Anesthesiology. 1974; 40:196–198. PMID: 4812720.
5. Fang ZX, Eger EI 2nd, Laster MJ, Chortkoff BS, Kandel L, Ionescu P. Carbon monoxide production from degradation of desflurane, enflurane, isoflurane, halothane, and sevoflurane by soda lime and Baralyme. Anesth Analg. 1995; 80:1187–1193. PMID: 7762850.
6. Baxter PJ, Kharasch ED. Rehydration of desiccated Baralyme prevents carbon monoxide formation from desflurane in an anesthesia machine. Anesthesiology. 1997; 86:1061–1065. PMID: 9158355.


7. Knolle E, Heinze G, Gilly H. Carbon monoxide formation in dry soda lime is prolonged at low gas flow. Anesth Analg. 2001; 93:488–493. PMID: 11473885.


8. Baxter AD. Low and minimal flow inhalational anaesthesia. Can J Anaesth. 1997; 44:643–652. PMID: 9187785.


9. Shiraishi Y, Ikeda K. Uptake and biotransformation of sevoflurane in humans: a comparative study of sevoflurane with halothane, enflurane, and isoflurane. J Clin Anesth. 1990; 2:381–386. PMID: 2271202.
10. Yasuda N, Lockhart SH, Eger EI 2nd, Weiskopf RB, Johnson BH, Freire BA, et al. Kinetics of desflurane, isoflurane, and halothane in humans. Anesthesiology. 1991; 74:489–498. PMID: 2001028.


11. Mchaourab A, Arain SR, Ebert TJ. Lack of degradation of sevoflurane by a new carbon dioxide absorbent in humans. Anesthesiology. 2001; 94:1007–1009. PMID: 11465591.


12. Liu J, Laster MJ, Eger EI 2nd, Taheri S. Absorption and degradation of sevoflurane and isoflurane in a conventional anesthetic circuit. Anesth Analg. 1991; 72:785–789. PMID: 2035861.


13. Langbein T, Sonntag H, Trapp D, Hoffmann A, Malms W, Röth EP, et al. Volatile anaesthetics and the atmosphere: atmospheric lifetimes and atmospheric effects of halothane, enflurane, isoflurane, desflurane and sevoflurane. Br J Anaesth. 1999; 82:66–73. PMID: 10325839.


14. Oyaro N, Sellevag SR, Nielsen CJ. Atmospheric chemistry of hydrofluoroethers: Reaction of a series of hydrofluoroethers with OH radicals and Cl atoms, atmospheric lifetimes, and global warming potentials. J Phys Chem A. 2005; 109:337–346. PMID: 16833352.


15. Ryan SM, Nielsen CJ. Global warming potential of inhaled anesthetics: application to clinical use. Anesth Analg. 2010; 111:92–98. PMID: 20519425.
16. Wald NJ, Idle M, Boreham J, Bailey A. Carbon monoxide in breath in relation to smoking and carboxyhaemoglobin levels. Thorax. 1981; 36:366–369. PMID: 7314006.


17. Frink EJ Jr, Nogami WM, Morgan SE, Salmon RC. High carboxyhemoglobin concentrations occur in swine during desflurane anesthesia in the presence of partially dried carbon dioxide absorbents. Anesthesiology. 1997; 87:308–316. PMID: 9286895.


18. Soro M, García-Pérez ML, Ferrandis R, Aguilar G, Belda EJ. Closed-system anaesthesia for laparoscopic surgery: is there a risk for carbon monoxide intoxication? Eur J Anaesthesiol. 2004; 21:483–488. PMID: 15248629.


19. Baum J, Sachs G, vd Driesch C, Stanke HG. Carbon monoxide generation in carbon dioxide absorbents. Anesth Analg. 1995; 81:144–146. PMID: 7598244.


20. Strauss JM, Bannasch W, Hausdorfer J, Bang S. The evolution of carboxyhemoglobin during long-term closed-circuit anesthesia. Anaesthesist. 1991; 40:324–327. PMID: 1883059.
Fig. 1
Changes in heart rate (HR), systolic blood pressure (sBP), and diastolic blood pressure (dBP) during laparoscopic surgery. Values are expressed as mean ± SD. pre-MF: pre-minimal-flow, pre-Pn: pre-pneumoperitoneum, Pn+5, 60, and 120: 5, 60, and 120 minutes after pneumoperitoneum, Pn-e: end of pneumoperitoneum, post-Pn: post pneumoperitoneum, End: before emergence.
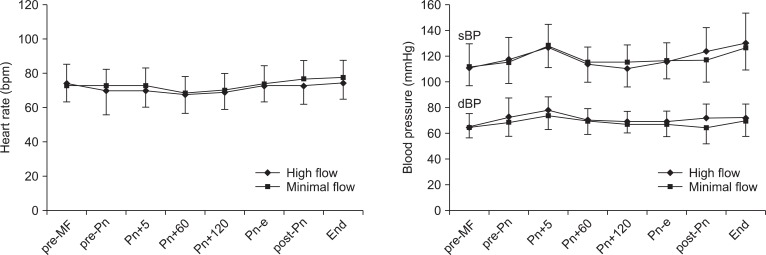
Fig. 2
Changes in inspiratory and expiratory desflurane concentrations. Values are expressed as mean ± SD. pre-MF: pre-minimal flow, pre-Pn: pre-pneumoperitoneum, Pn+5, 60, and 120: 5, 60, and 120 minutes after pneumoperitoneum, Pn-e: end of pneumoperitoneum, post-Pn: post pneumoperitoneum, End: before emergence.
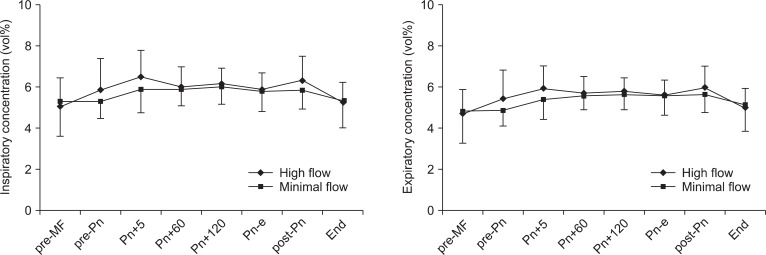
Fig. 3
Changes in inspiratory oxygen concentrations. Values are expressed as mean ± SD. pre-MF: pre-minimal flow, pre-Pn: pre-pneumoperitoneum, Pn+5, 60, and 120: 5, 60, and 120 minutes after pneumoperitoneum, Pn-e: end of pneumoperitoneum, post-Pn: post pneumoperitoneum, End: before emergence. *P < 0.05 compared with preop values.
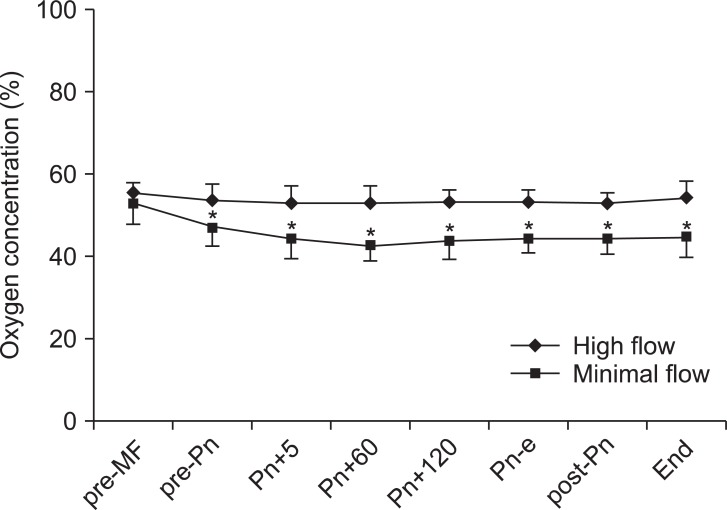
Fig. 4
Changes in COHb concentrations. Values are expressed as mean ± SD. COHb: carboxyhemoglobin. Tbaseline: before induction at room air, T2, T4, and T6: 2, 4, and 6 hours after induction, Trecovery: recovery room after extubation. *P < 0.05 compared with high flow values.
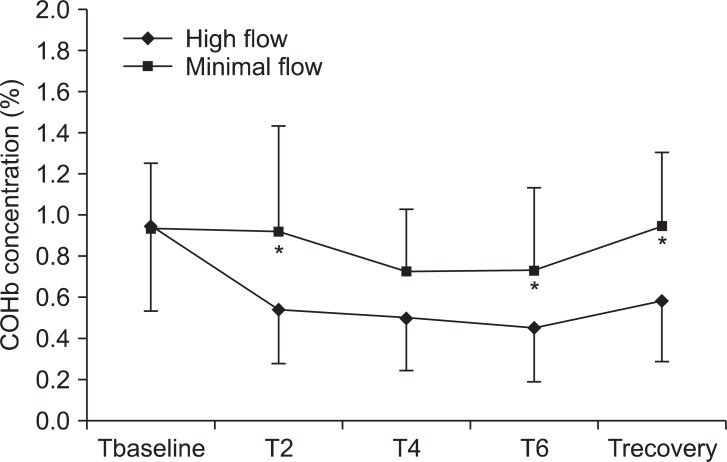