Abstract
Background
Recently, the addition of dexmedetomidine to sedation regimens after cardiac surgery had been reported and there is a possibility that dexmedetomidine can cause vasoconstriction. Vasopressin has been used as a prophylactic treatment for refractory vasodilatory shock during coronary artery bypass graft (CABG). Also, vasopressin may play an important role in initiating spasms at the graft artery. Here we evaluate the direct effect of dexmedetomidine on isolated human gastroepiploic arteries and the synergistic effect of dexmedetomidine and vasopressin.
Methods
Discarded gastroepiploic arteries from elective subtotal gastrectomy (n = 10) were used in this study. We measured the level of contraction in isolated human gastroepiploic arteries induced by increasing concentrations of dexmedetomidine (10-10 to 10-6 M) with or without vasopressin (10-10, 10-9 M). Arterial contractions caused by increasing concentrations of vasopressin (10-10 to 10-7.5 M) with or without dexmedetomidine (10-9, 10-7 M) were also measured in the tissue samples.
Results
Supraclinical concentrations of dexmedetomidine elicited contractions at concentrations of 10-7 M and 10-6 M (P < 0.05 versus resting tension). The same concentrations of dexmedetomidine (10-7, 10-6 M) significantly enhanced vasopressin-induced contractions (P < 0.05 versus vasopressin-induced contraction). Vasopressin produced concentration-dependent contractions and vasopressin (10-10, 10-9.5, 10-9 M) also increased the intensity of dexmedetomidine (10-7 M) induced contractions.
Conclusions
There was a synergistic effect between supraclinical doses of dexmedetomidine and vasopressin on the degree of contraction in isolated human gastroepiploic arteries. However, a sedative dose of dexmedetomidine (clinical dose: 0.2-0.7 µg/kg/hr, plasma concentration: 0.36-1.25 ng/ml) did not enhance vasopressin induced-contraction in isolated human gastroepiploic arteries.
Persistent hypotension associated with low systemic vascular resistance and high cardiac output has been termed "vasoplegic syndrome" [1]. Treatment for vasoplegic syndrome involves inotropic support and the administration of high doses of vasopressors. Recent studies have reported that intravenous administration of vasopressin was beneficial in patients with persistent hypotension associated with sepsis or from cardiac surgeries involving cardiopulmonary bypass (CPB) [2-4]. Vasopressin is important in the maintenance of vascular tone after CPB, as a deficiency in vasopressin contributes to vasoplegic syndrome. Both high dose (0.1 to 1 IU/min) and low dose (0.03 IU/min) vasopressin are effective in the treatment of vasoplegic syndrome after cardiac surgeries involving CPB [3,4]. However, vasopressin can be a strong vasoconstrictor in the internal thoracic and radial arteries [5], and these vessels are often used for coronary artery bypass grafting (CABG) [6].
The use of dexmedetomidine (DMT) is associated with a reduction in the dosage of anesthetics and analgesic drugs used during the perioperative period, and it is also used in clinical practice for sedation in the ICU after cardiac operations. Activation of presynaptic α2-adrenoceptors on sympathetic nerves can lead to sympatholysis in the central nervous system. However, activation of vascular postsynaptic receptors causes both vasoconstriction (through activation of the α2-adrenoceptors on the vascular smooth muscle cells) and vasodilatation (through the activation of α2-adrenoceptors on the endothelial cells). Recently, it has been reported that systemically administered DMT caused vasoconstriction with doses lower than the clinically recommended dose [7].
Since vasopressin is one of the principal vasoconstrictors for human vessels and the mechanisms of its vasoconstrictive effect are likely different than the mechanisms for DMT-induced vasoconstriction, there is a possibility of a synergic effect with vasopressin and DMT in human arteries. Despite extensive use of both drugs to induce vasoconstriction, there is scant information available in their postoperative management for CABG. In this study, we hypothesized that DMT can enhance vasopressin-induced contraction in human gastroepiploic arteries (GEA). Moreover, we evaluated the effect of vasopressin on DMT-induced contraction in human GEA.
This study was approved by the Institutional Review Board of the University Hospital. After informed consent was obtained, discarded GEAs were obtained from patients who underwent subtotal gastrectomy (n = 10, 43-74 yr). GEA segments were collected and immediately delivered to the laboratory. The tissues were then placed in an oxygenated physiologic salt solution (PSS) that was maintained at 4℃. Fat and connective tissues were carefully removed, and vascular rings (2-3 mm length) were prepared. The rings were then suspended on wires in organ baths filled with PSS. Four segments in separate organ baths with PSS were run in parallel. The PSS had the following composition (mM/L): Na+, 137.4; HCO3-, 15.5; K+, 5.9; Ca2+, 2.6; H2PO4-, 1.2; Mg2+,1.2; Cl-, 134.4; and glucose, 11.5. All solutions were continuously aerated with a mixture of 95% oxygen and 5% carbon dioxide, the pH was adjusted to 7.3-7.4 and the temperature was kept at 37℃.
Two stainless steel hooks were introduced through the lumen of the vascular ring. One hook was anchored to the bottom of the organ bath, and the other was connected to a strain gauge to measure the level of isometric force. The vascular rings were stretched gradually to a resting tension of 2 g during a 90-120 min equilibration period to obtain optimal tension. After the equilibration period, 60 mM of KCl was added to the baths and the contractile force was recorded. For each vessel segment, the greatest contraction elicited by KCl was used as a reference point for the remaining contractions and test contractions were expressed as a percentage of the reference contraction. The vascular rings were then washed (at least 2 times) with PSS to restore the basal contraction levels (i.e. vascular rings had a second equilibration period).
The following drugs were used: DMT (Orion Pharma, Turku, Finland) and arginine vasopressin (Sigma-Aldrich Korea, Youngin, Kyunggi, Korea).
To establish a dose-response curve, increasing doses of DMT (10-10, 10-9, 10-8, 10-7 and 10-6 M) were added in a cumulative fashion to the organ baths at 3 min intervals to allow the vascular rings to respond to each concentration, and the contractile force was subsequently assessed after each addition.
Then, vasopressin (10-10 or 10-9 M) was also added to the organ baths. After 5 min, DMT was added cumulatively in increasing doses (10-10, 10-9, 10-8, 10-7 and 10-6 M) to the organ baths at 3 min intervals and the contractile force was assessed as above.
To establish a dose response for vasopressin (10-10, 10-9.5, 10-9, 10-8.5, 10-8, and 10-7.5 M), it was added cumulatively to the organ baths at 3 min intervals and the contractile response was assessed after each addition.
Next, DMT (10-9 or 10-7 M) was added to the organ baths. After 5 min, increasing concentrations of vasopressin were then added cumulatively (10-10, 10-9.5, 10-9, 10-8.5, 10-8, and 10-7.5 M) to the organ baths at 3 min intervals, and the contraction force was assessed after each addition.
Contractile responses were expressed as percentages of the maximum contraction in isotonic 60 mM KCl. When similar experiments were performed on more than one segment from the same patient, a mean for each patient was calculated before statistical analysis.
Results were expressed as the mean ± SD. The data were analyzed by analysis of variance (ANOVA), followed by a Scheffe post-hoc test to detect individual differences and by an unpaired t test to compare two independent groups. Differences were considered significant when P < 0.05.
When DMT (10-10, 10-9, 10-8, 10-7 and 10-6 M) was added cumulatively to the organ bath, the drug induced significant contractions at the higher concentrations (10-7 M: 5.6 ± 4.2%, 10-6 M: 9.3 ± 5.7% of the maximal contraction produced by 60 mM of KCl; P < 0.05 versus resting tension, Fig. 1). High concentrations of DMT significantly enhanced contractions induced by 10-10 M of vasopressin (10-7 M: 19.3 ± 4.9%, 10-6 M: 27.6 ± 5.8% of the maximum contraction produced by 60 mM of KCl; P < 0.05 versus 10-10 M vasopressin-induced contraction, Fig. 2).
High concentrations of DMT similarly enhanced the contractions induced by 10-9 M of vasopressin (10-7 M: 80.2 ± 9.7%, 10-6 M: 86.6 ± 11.2% of the maximum contraction produced by 60 mM of KCl; P < 0.05 versus 10-9 M vasopressin-induced contraction, Fig. 3). When vasopressin (10-10 to 10-7.5 M) was added cumulatively to the vessel segments in the organ bath, the drug induced concentration-dependent contractions (Fig. 4).
Vasopressin did not significantly enhance contractions elicited by 10-9 M concentrations of DMT (Fig. 5). However, lower concentrations of vasopressin (10-10, 10-9.5, and 10-9 M) did significantly enhance contractions induced by 10-7 M DMT (10-10 M: 19.4 ± 3.8%, 10-9.5 M: 23.4 ± 4.3%, 10-9 M: 88.9 ± 14.1% of the maximum contraction produced by 60 mM of KCl; P < 0.05 versus 10-7 M DMT-induced contraction, Fig. 6).
In the present study, we explored the effects of DMT on vasopressin-induced contractions on human GEA. In our study, DMT alone caused significant vasoconstriction at the higher concentrations (10-7 and 10-6 M). When high levels of DMT were added to vessels already exposed vasopressin, there was a significant enhancement of vasopressin-induced contractions, indicating a a synergistic effect between high levels of DMT and vasopressin on vasoconstriction in human GEA.
DMT is the most selective α2-adrenoceptor agonist available for clinical use. Rapid injection of DMT can cause vasoconstriction and result in transient hypertension through the activation of α2-adrenoceptors on vascular smooth muscle cells [8,9]. The stimulation of α1-adrenoceptors by higher concentrations of DMT may also contribute to vasoconstriction [10,11]. This suggests that, when there is no sympathetic inhibition from the central nervous system (in other words, when there is no effects of α2-adrenoceptor agonists in the central nervous system), the peripheral vascular effect (i.e. vasoconstriction) is unmasked. Talke et al. [7] reported that systemic administration of DMT led to peripheral vasoconstriction with minimal contribution from the sympatholytic effects of the drug. Yildiz et al. [12] suggested that DMT induced concentration-dependent contractions in isolated human internal mammary arteries by activating α2-adrenoceptors at lower concentrations and α1-adrenoceptors at higher concentrations. However, only large doses of DMT (10-7 and 10-6 M) in vitro produced significant contractions alone in human GEA in our experiment. Our results suggest that clinical doses (< 10-7 M, 0.2-0.7 µg/kg/hr) of DMT could not induce smooth muscle contraction in human GEA and these findings are contrary to previously published reports [12].
When DMT was added to vessels with vasopressin already present, there was a significant enhancement of vasopressin-induced contraction, but only with high doses of DMT (10-7 and 10-6 M). Previous reports have suggested that α2-adenoreceptor-induced contractions are dependent on Ca2+ influx through the L-type voltage-dependent calcium channels [13]. In our experiment, DMT may have directly affected the vascular smooth muscles at higher concentrations (> 10-7 M) and this is similar to previously reported results. Nielsen et al. suggested that compared to responses mediated by stimulation of postjunctional α1-adrenoceptors, stimulation of postjunctional α2-adrenoceptors relies heavily on calcium influx. Stimulation of postjunctional α2-adrenoceptors is, however, also coupled to intracellular release of calcium in isolated human subcutaneous resistance arteries.
When vasopressin (10-10 to 10-7.5 M) was added cumulatively to the vessel segments in the organ bath, it produced concentration-dependent contractions. DMT at 10-7 M further enhanced vasopressin-induced contractions with either physiologic or clinical doses of vasopressin (10-10, 10-9.5, 10-9 M). However, 10-9 M of DMT did not enhance vasopressin-induced contractions even at supraclinical concentrations of vasopressin (> 10-9 M). Henderson and Byron [14] reported that vasopressin-induced contraction has two concentration-dependent signaling pathways. Protein kinase C activation and Ca2+ entry through the voltage-dependent L-type calcium channels are required for picomolar vasopressin-induced contractions. Moreover, nanomolar concentrations of vasopressin can acutely activate the phospholipase C and inositol 1,4,5-triphosphate-dependent Ca2+ release pathways. In our study, vasopressin-induced contractions at picomolar levels was enhanced by adding 10-7 M of DMT. Thus, this indicated that DMT only directly affected the vascular smooth muscles at higher concentrations (> 10-7 M) and that it produced a marked enhancement of the vasopressin-induced contractions through voltage-dependent L-type calcium channels. The vasoconstrictive activity of vasopressin also requires the Ca2+ signaling pathway. The mechanism of vasopressin is related to the stimulation of V1-receptors on vascular smooth muscle cells and increased intracellular Ca2+ leads to arteriolar vasoconstriction. The degree of vasoconstriction is dependent on the diameter and location of the artery. Vasopressin-induced contraction at nanomolar doses involved a different signaling pathway and the contraction effect was more potent than the effect seen with 10-7 M of DMT.
Our study has several limitations. First, several reports have already shown the inhibitory effect of DMT on phenylephrine-induced contraction in GEA and in internal mammary arteries. This effect was related to the presynaptic α2-antagonist effect of DMT or the activation of α2-adrenoreceptors on endothelial cells, both of which can trigger the release of endothelium-derived nitric oxide. In our study, we used isolated human GEA and they were denervated, i.e. without a presynaptic α2-antagonist effect of DMT. The presynaptic α2-antagonist effect of DMT is important. Therefore, it is hard to directly apply our results to the clinical setting. Second, although all arterial grafts develop vasospasms, it occurs more frequently in GEA [15] and this led us to select isolated GEA for our experiments. However, there is a possibility that the results may be different in other vessels. Generally, vasospasm of the graft artery is related to the endothelial function of the graft artery. The nitric oxide and the endothelium-derived hyperpolarizing factor released from the endothelium of the graft artery may inhibit the contraction of the graft arteries. In this study with GEA, vasopressin-induced contractions overcame the effect of endothelium-derived relaxing factor. Finally, one should be careful to extrapolate the results from this study to clinical applications. There may be many factors that can cause graft vessel spasm in the clinic. These include patient and technical variables during the operation as well as the hormonal environment. Moreover, in our experiments, there was no influence from the central nervous system, as only the α2-adrenoceptor effect were present in vascular smooth muscle cells.
In conclusion, though DMT did enhance vasopressin-induced contraction, it did not directly lead to contraction of isolated human GEA at clinical doses (0.2-0.7 µg/kg/hr). At supraclinical doses (> 10-7 M ), DMT significantly affected the vascular smooth muscle tone and enhanced vasopressin-induced contraction. However, one should be cautious to use DMT for sedation of patients who underwent CABG and to use vasopressin in patients because our study was performed with denervated GEA in isolated preparations.
References
1. Gomes WJ, Carvalho AC, Palma JH, Teles CA, Branco JN, Silas MG, et al. Vasoplegic syndrome after open heart surgery. J Cardiovasc Surg (Torino). 1998; 39:619–623.
2. Dünser MW, Mayr AJ, Ulmer H, Ritsch N, Knotzer H, Pajk W, et al. The effects of vasopressin on systemic hemodynamics in catecholamine-resistant septic and postcardiotomy shock: a retrospective analysis. Anesth Analg. 2001; 93:7–13. PMID: 11429329.


3. Masetti P, Murphy SF, Kouchoukos NT. Vasopressin therapy for vasoplegic syndrome following cardiopulmonary bypass. J Card Surg. 2002; 17:485–489. PMID: 12643457.


4. Morales DL, Garrido MJ, Madigan JD, Helman DN, Faber J, Williams MR, et al. A double-blind randomized trial: prophylactic vasopressin reduces hypotension after cardiopulmonary bypass. Ann Thorac Surg. 2003; 75:926–930. PMID: 12645718.


5. Wei W, Floten HS, He GW. Interaction between vasodilators and vasopressin in internal mammary artery and clinical significance. Ann Thorac Surg. 2002; 73:516–522. PMID: 11845867.


6. Wei W, Yang CQ, Furnary A, He GW. Greater vasopressin-induced vasoconstriction and inferior effects of nitrovasodilators and milrinone in the radial artery than in the internal thoracic artery. J Thorac Cardiovasc Surg. 2005; 129:33–40. PMID: 15632822.


7. Talke P, Lobo E, Brown R. Systemically administered alpha2-agonist-induced peripheral vasoconstriction in humans. Anesthesiology. 2003; 99:65–70. PMID: 12826844.
8. Ebert TJ, Hall JE, Barney JA, Uhrich TD, Colinco MD. The effects of increasing plasma concentrations of dexmedetomidine in humans. Anesthesiology. 2000; 93:382–394. PMID: 10910487.


9. Link RE, Desai K, Hein L, Stevens ME, Chruscinski A, Bernstein D, et al. Cardiovascular regulation in mice lacking alpha2-adrenergic receptor subtypes b and c. Science. 1996; 273:803–805. PMID: 8670422.
10. Hamasaki J, Tsuneyoshi I, Katai R, Hidaka T, Boyle WA, Kanmura Y. Dual alpha(2)-adrenergic agonist and alpha(1)-adrenergic antagonist actions of dexmedetomidine on human isolated endothelium-denuded gastroepiploic arteries. Anesth Analg. 2002; 94:1434–1440. PMID: 12032002.
11. Coughlan MG, Lee JG, Bosnjak ZJ, Schmeling WT, Kampine JP, Warltier DC. Direct coronary and cerebral vascular responses to dexmedetomidine. Significance of endogenous nitric oxide synthesis. Anesthesiology. 1992; 77:998–1006. PMID: 1359812.
12. Yildiz O, Ulusoy HB, Seyrek M, Gul H, Yildirim V. Dexmedetomidine produces dual alpha2-adrenergic agonist and alpha1-adrenergic antagonist actions on human isolated internal mammary artery. J Cardiothorac Vasc Anesth. 2007; 21:696–700. PMID: 17905276.
13. Nielsen H, Mortensen FV, Pilegaard HK, Hasenkam JM, Mulvany MJ. Calcium utilization coupled to stimulation of postjunctional alpha-1 and alpha-2 adrenoceptors in isolated human resistance arteries. J Pharmacol Exp Ther. 1992; 260:637–643. PMID: 1346639.
14. Henderson KK, Byron KL. Vasopressin-induced vasoconstriction: two concentration-dependent signaling pathways. J Appl Physiol. 2007; 102:1402–1409. PMID: 17204577.


15. He GW, Yang CQ. Comparison among arterial grafts and coronary artery. An attempt at functional classification. J Thorac Cardiovasc Surg. 1995; 109:707–715. PMID: 7715218.


Fig. 1
Effects of increasing concentrations of dexmedetomidine on human gastroepiploic arterial contraction. Data are expressed as percent (%) contraction of dexmedetomidine to a contraction induced from addition of 60 mM KCl.
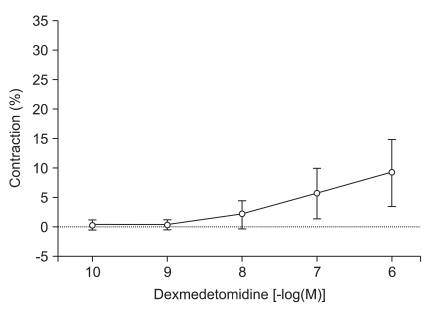
Fig. 2
Dexmedetomidine induced contraction of human gastroepiploic artery with and without vasopressin (10-10 M). Data are expressed as percent (%) contraction of dexmedetomidine to a contraction induced from addition of 60 mM KCl. A10: contraction from 10-10 M of arginine vasopressin treatment, DA10: contraction from increasing doses of dexmedetomidine after vasopressin (10-10 M) treatment, *P < 0.05 when compared with that of 10-10 M of arginine vasopressin treatment.
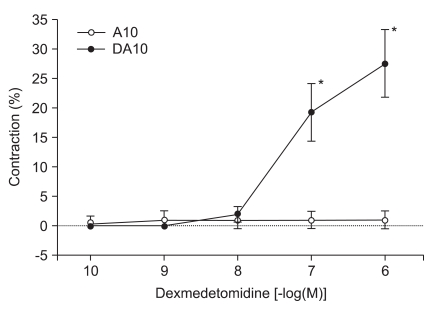
Fig. 3
Dexmedetomidine-induced contractions in human gastroepiploic arteries with and without vasopressin (10-9 M). Data are expressed as percent (%) contraction of dexmedetomidine to a contraction induced from addition of 60 mM KCl. A9: contraction from 10-9 M of arginine vasopressin treatment, DA9: contraction from increasing doses of dexmedetomidine after vasopressin (10-9 M) treatment, *P < 0.05 when compared with that of 10-9 M of arginine vasopressin treatment.
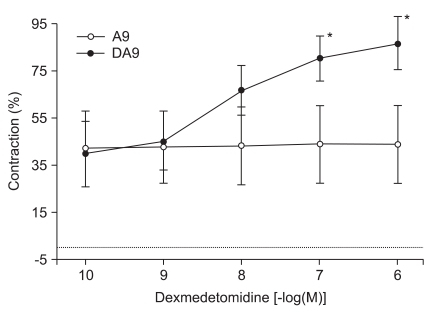
Fig. 4
Increasing concentrations of vasopressin-induced contractions in human gastroepiploic arteries. Data are expressed as percent (%) contraction of vasopressin to a contraction induced from addition of 60 mM KCl.
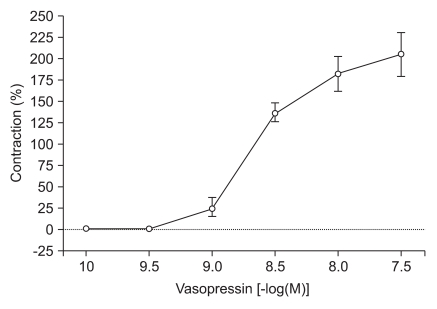
Fig. 5
Vasopressin induced contraction with and without dexmedetomidine (10-9 M) in human gastroepiploic arteries. Data are expressed as percent (%) contraction of dexmedetomidine to a contraction induced from addition of 60 mM KCl. A: contraction from arginine vasopressin treatment, AD9: contraction from increasing doses of vasopressin after dexmedetomidine (10-9 M) treatment, D9: contraction from 10-9 M of dexmedetomidine treatment.
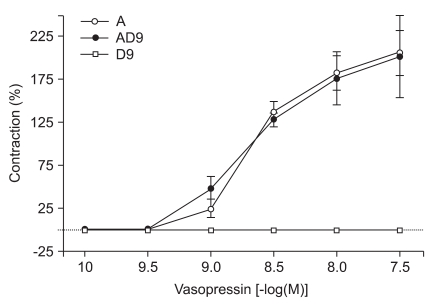
Fig. 6
Vasopressin induced contraction with and without dexmedetomidine (10-7 M) in the human gastroepiploic artery. Data are expressed as percent (%) contraction of vasopressin to a contraction induced from addition of 60 mM KCl. A: contraction from arginine vasopressin treatment, AD7: contraction from increasing doses of vasopressin after dexmedetomidine (10-7 M) treatment, D7: contraction from 10-7 M of dexmedetomidine treatment, *P < 0.05 when compared with that of arginine vasopressin without dexmedetomidine treatment.
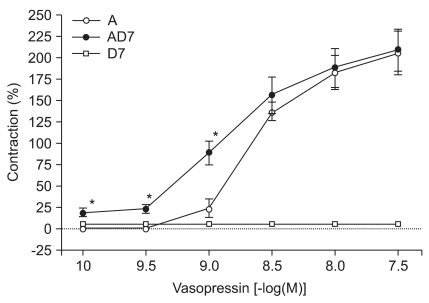