Abstract
Background
Ischemia reperfusion (IR) injury is a complex phenomenon that leads to organ dysfunction and causes primary liver failure following liver transplantation. We investigated whether an intravenous administration of magnesium before reperfusion can prevent or reduce IR injury.
Methods
Fifty-nine living donor liver transplant recipients were randomly assigned to an MG group (n = 31) or an NS group (n = 28). Each group was also divided in two groups based on the preoperative magnesium levels (normal: ≥ 0.70 mmol/L, low: < 0.70 mmol/L). The MG groups received 25 mg/kg of MgSO4 mixed in 100 ml normal saline intravenously before reperfusion and the NS groups received an equal volume of normal saline. The levels of lactate, pH, arterial oxygen tension, and base excess were measured to assess reperfusion injury at five specific times, which were 10 min after the beginning of anhepatic phase, and 10, 30, 60 and 120 min after reperfusion. To evaluate postoperative organ function, the serum aspartate aminotransferase (AST), alanine aminotransferase (ALT), total bilirubin and creatinine levels were measured at preoperative day 1, postoperative day 1 and 5.
With advances in critical care management, immunosuppression and surgical techniques, liver transplantation has become one of the most effective treatments for acute or chronic liver failure [1]. In Korea, living donor liver transplantation (LDLT) has been more frequently performed than orthotopic liver transplantation because of the critical shortage of cadaveric donor organs [2]. During liver transplantation, ischemia reperfusion (IR) injury occurs after hepatic vascular clamping and reperfusion of the grafted liver. IR injury is a phenomenon that hypoxic cellular damage is aggravated following the restoration of oxygen delivery and it may be a major cause of primary liver failure after liver transplantation [3-5].
The process of IR injury after reperfusion of the grafted liver has been divided into two phases [4,5]. The early phase (< 2 hours after reperfusion) involves abnormal increases in intracellular calcium concentration, and generation of reactive oxygen species by Kupffer cells, which results in subsequent hepatocyte injury and death. The late phase of liver injury (> 6 hours after reperfusion) is an inflammatory disorder mediated by recruited neutrophils. This cellular response to ischemia and reperfusion can lead to a systemic inflammatory response and subsequent cellular damage. Thus, there have been many efforts to reduce or prevent IR injury, including surgical interventions, pharmacologic agents and gene therapy, but few treatments are currently available.
IR injury also occurs in myocardial infarction, ischemic spinal cord injury and stroke. Recent experiments have shown protective effects of magnesium to reduce the reperfusion injury of these conditions [6-9]. The intravenous administration of magnesium during coronary occlusion has caused a significant reduction in myocardial infarct size [6]. This is because magnesium provide cellular protection during ischemia and reperfusion by stabilizing the cellular transmembrane potential, suppressing excessive calcium influx and reducing cellular energy demand [10]. Magnesium administration also enhances electrophysiological and neurobehavioral recovery and reduces brain infarction after cerebral ischemia-reperfusion [8]. Kaplan et al. [9] reported that the use of magnesium may have protective effects on reperfusion injuries of the spinal cord in rabbits.
Despite these advances, little is known about the effects of magnesium on hepatic IR injury during liver transplantation. The primary aim of this study was to evaluate the effects of intravenous magnesium infusion before reperfusion of the grafted liver by employing blood lactate levels and some clinical parameters, since the intraoperative changes in the blood lactate levels after hepatic allograft reperfusion served as an accurate predictor of the initial graft function in LDLT [11]. The secondary objective was to examine the postoperative organ function to detect delayed effects of magnesium.
The study was approved by the Institutional Review Board and written informed consent was obtained from all the patients. We studied adult patients who were scheduled for elective LDLT using the right hepatic lobe. The exclusion criteria were pediatric patients, re-transplantation and patients with renal dysfunction or cardiovascular disease. Liver transplantation was always performed by the same team of surgeons and anesthesiologists using the same surgical techniques.
Demographic data, Child-Turcotte-Pugh (CTP) classification [12], United Network for Organ Sharing (UNOS) status [13], Model for End-stage Liver Disease (MELD) score [14] and the diagnosis were obtained from the medical records. Patients were randomized to receive magnesium solution (MG group, n = 31) or normal saline (NS group, n = 30). Each group was also divided in two groups as the normal and the low group based on the preoperative magnesium levels (normal group: ≥ 0.70 mmol/L, low group: < 0.70 mmol/L).
Allocation concealment was ensured using sequentially numbered sealed opaque envelopes. Immediately after arrival of the patient in the operating room, an anesthesiologist not involved in the study opened the envelopes and prepared the study solution outside the operating room.
Thirty minutes after the beginning of the anhepatic phase, the patients received a magnesium solution (25 mg/kg of MgSO4 mixed in 100 ml of normal saline) or an equivalent volume of normal saline over 20 min. Other anesthesiologist, who was blinded to the group assignment, performed intravenous administration of the study solution and assessed study outcomes.
Administration of fluid, vasopressors and inotropes were guided by hemodynamic monitoring and clinical parameters (central venous pressure: 8 to 10 mmHg, systolic blood pressure > 90 mmHg, heart rate < 100 beat/min, pulmonary artery wedge pressure: 10 to 14 mmHg, urine output: 0.5 to 1 ml/kg/hr). Packed red blood cells were transfused to keep the hematocrit at 30%. Administration of fresh frozen plasma and platelets were based on clinical findings by inspection of the surgical field and the results of thromboelastography. Platelets were given if platelet count decreased to < 50 × 109/L. Calcium chloride and sodium bicarbonate were used to correct low ionized Ca2+ levels (Ca2+ < 0.9 mmol/L) and metabolic acidosis (pH < 7.2 and base excess < -10 mmol/L), respectively.
All patients who remained intubated after surgery were transferred directly to the intensive care unit (ICU) for postoperative care. Lengths of stay in the ICU were recorded for all patients.
The plasma total magnesium concentrations were measured four times: preoperatively (one day before surgery: the baseline), 10 minutes after the beginning of the anhepatic phase, 10 minutes after reperfusion and postoperative day 1 (POD 1).
Arterial blood samples were analyzed after anesthesia induction, 10 minutes after the beginning of the anhepatic phase and 10, 30, 60 and 120 minutes after reperfusion using a blood gas analyzer (ABL800 FLEX, Radiometer, Copenhagen, Denmark). The measured variables included arterial pH, arterial oxygen tension (PaO2), base excess, lactate and glucose as indicators of ischemic injury and perfusion markers to evaluate the effects of magnesium on reperfusion injury. The mean arterial pressure (MAP) was recorded at the same time. Blood lactate levels were measured using ABL800 FLEX that employs a technique based on spectrophotometry analysis adopting a lactate dehydrogenase (LDH) method. The precision of this method of lactate measurement has been previously validated [15-17].
For estimating postoperative organ dysfunction, serum concentrations of aspartate aminotransferase (AST), alanine aminotransferase (ALT), total bilirubin and creatinine were measured preoperatively, as well as on POD 1 and POD 5.
The sample size estimation was based on the mean value of the blood lactate levels from our preliminary study. Twenty five patients per group were required to show a 50% difference in blood lactate levels, with a two sided α of 0.05 and a β of 0.2 (power = 80%). All the analyses were performed using SAS software version 9.1 (SAS Institute, Cary, NC, USA) and the results were expressed as means ± standard deviations. Continuous variables were compared by repeated measures analysis of variance (ANOVA). Where post hoc testing was applied, a Bonferroni adjustment was used and P value was corrected. Simple comparisons of age, weight, and height were made by using one-way ANOVA. Categorical variables were compared by using the Chi-square test or Fisher's exact test. P values of < 0.05 were considered statistically significant.
Sixty-one patients were enrolled in this study and two patients in the NS groups were excluded because of postoperative bleeding and re-operation. Distributions of patients and clinical profiles are shown in Table 1. The demographic characteristics, CTP classification, UNOS status and MELD score did not differ among the groups. Hepatitis B virus (HBV) or hepatitis C virus (HCV) related liver cirrhosis and hepatocellular carcinoma were the most common liver diseases in all groups. Intraoperative transfusion requirement and the length of ICU stay were not different among the groups.
The magnesium concentrations increased significantly after magnesium administration in the magnesium-treated groups (Normal-MG and Low-MG group) compared to the NS groups (Normal-NS and Low-NS group, Fig. 1). At POD 1, the magnesium concentrations decreased but the levels were within the normal range in magnesium-treated groups. However, the magnesium levels in the NS groups constantly decreased and the levels fell below the normal range at POD 1 (Fig. 1).
The blood lactate levels were similar in all groups at baseline, but significantly reduced at 10, 30, 60 and 120 min after reperfusion in the magnesium-treated groups. However there was a continuous elevation of blood lactate levels in the NS groups. In addition, significantly higher blood lactate levels were observed in Low-NS group than magnesium-treated groups (Fig. 2).
After reperfusion, the pH values significantly decreased compared to the anhepatic phase. However, there were no differences in pH, base excess, PaO2 and MAP among the groups (Fig. 3).
At POD 1, the serum AST, ALT, total bilirubin and creatinine levels significantly increased compared to the preoperative values in all groups. These parameters decreased at POD 5, but there was no difference among the groups (Fig. 4).
The purpose of this study was to evaluate whether administration of magnesium has protective effects against reperfusion injury in liver transplantation. During ischemia and reperfusion of liver, ATP depletion and endothelial injury cause an increase in anaerobic metabolism that leads to lactate accumulation [18]. Our results showed that the blood lactate levels after reperfusion were significantly lower in the MG groups compared to the NS groups.
The blood lactate level is often used clinically as a marker of anaerobic metabolism and may represent inadequate tissue perfusion. Elevated blood lactate levels significantly correlated with the severity of hypoperfusion, hypoxia and severe ischemic damage of the liver [19]. During ischemia and reperfusion, injury to the endothelial cell resulted in inhibition of pyruvate dehydrogenase and consequently caused anaerobic glycosis, glycogenolysis, and lactate accumulation [20,21]. There are two major mechanisms for hyperlactatemia in liver transplantation: the shift to anaerobic glycolysis due to tissue hypoperfusion and the decrease of lactate clearance by insufficient liver function [22].
In previous studies [11,18], hyperlactatemia was shown to possess prognostic significance in liver transplantation. The lactate levels rose during the preanhepatic and anhepatic phases and peaked at 10 min after reperfusion. Following reperfusion, a constant decrease in the lactate level was recorded in the group with a good immediate graft function [18]. Some investigators have suggested the intraoperative changes in the blood lactate levels after hepatic allograft reperfusion served as an accurate predictor of the initial graft function in LDLT [11]. Therefore, we measured blood lactate levels before and after reperfusion of the grafted liver to evaluate the effects of magnesium treatment on IR injury.
Magnesium is an important cofactor for many enzymatic reactions. It is essential for the production and functioning of ATP, therefore preserves energy-dependent cellular activity, particularly during ischemic episodes. Magnesium also blocks calcium entry into the cell by acting on voltage receptor and receptor-operated membrane channels [10]. Since calcium overload has an important role in the pathogenesis of reperfusion injury [5], magnesium may provide cellular protection during ischemia.
Patients with end-stage liver disease usually show hypomagnesemia before liver transplantation either due to decreased intake and gastrointestinal absorption of magnesium, or to increased excretion of magnesium caused by the treatments of diuretics for ascites [23-25]. A significant decrease of the plasma magnesium concentration occurs during both dissection and anhepatic phase in orthotopic liver transplantation [24]. In this study, magnesium levels constantly decreased during liver transplantation in NS groups. Since magnesium is important for the maintenance of cardiovascular homeostasis and neurological stability, any acute changes in the magnesium levels must be detected and treated promptly [25].
However, the over-normalization of the magnesium levels must be cautiously avoided. Hypermagnesemia can cause peripheral vasodilation, leading to worsening of hypotension during the reperfusion phase in liver transplantation. In addition, magnesium is a powerful cerebral vasodilator that may be deleterious to patients with hepatic encephalopathy and increased intracranial pressure [24]. Thus, magnesium supplementation should be titrated with plasma magnesium levels to avoid hypermagnesemia and the associated risk of unwanted side effects. Magnesium is usually given in amount of 2 g intravenously over 30 min to correct hypomagnesemia [10]. In the second Leicester Intravenous Magnesium Intervention Trial (LIMIT-2) [7], patients with acute myocardial infarction randomly received magnesium 2 g or placebo over 5 min, before thrombolytic therapy, followed by 16 g as infusion over the next 24 h. Serum magnesium concentrations were approximately doubled for 24 h and returned to normal by 48 h. In a previous study [26], when 1.5 g of magnesium sulfate mixed in 100 ml of normal saline was infused, the magnesium concentration increased within the normal range during LDLT. From these results, we selected the dose (25 mg/kg) of magnesium sulfate because the administration of 1.5 to 2 g of magnesium to patients of 60 to 70 kg corresponds to 25 mg/kg. Our results showed that this dose of magnesium was adequate to maintain the normal range of the magnesium levels without inducing hypermagnesemia.
Magnesium deficiency is associated with enhanced lipid peroxidation and production of reactive oxygen species during ischemia [27-29]. In another study [30], magnesium supplementation markedly inhibited lactate production and concomitant lipid peroxidation after cerebral ischemia. In this study, we observed higher blood lactate levels after reperfusion in the NS group with preoperative hypomagnesemia than those with normal preoperative magnesium levels. In magnesium-treated groups, blood lactate levels were significantly lower at 10, 30, 60, and 120 min after reperfusion than in the NS group with preoperative hypomagnesemia. In addition, the MAP values, which are probably the most useful parameter to assess organ perfusion, were adequately maintained in all groups for 2 h after reperfusion. Based on these results, the differences in lactate levels were probably not due to tissue perfusion, but rather, correlated with magnesium administration.
The pH values significantly decreased after reperfusion, possibly due to the acid load entering the systemic circulation from the ischemic liver, despite attempts to compensate for this. The fall in pH at reperfusion was associated with a concomitant rise in blood lactate levels. However, there were no differences in the values of pH and base excess among the groups for 120 min after reperfusion. Thus, it is difficult to conclude that the magnesium employed in the present study has protective effects on these parameters associated with reperfusion injury.
We also compared postoperative AST, ALT, total bilirubin and serum creatinine levels to detect the delayed effects of magnesium on organ injury. These parameters significantly increased at POD 1 and decreased at POD 5, but there was no difference among the groups.
In cerebral IR injury, magnesium treatment significantly suppressed the increase of lactate concentrations, and improved EEG changes when compared with the untreated group [30]. The neuroprotective effects of magnesium may be explained as follows: magnesium is a well-known N-methyl-D-aspartate (NMDA) receptor blocker and may prevent intracellular accumulation of calcium ion. Magnesium may decrease endothelial and neuronal reperfusion injuries by directly inhibiting lipid peroxidation and by preventing glutathione depletion, regulate ATP concentrations and regenerate ATP after ischemia and reperfusion [31]. In the present study, magnesium treatment for hepatic IR injury attenuated the increase of lactate levels, but this result was not correlated with the protective effects of magnesium on postoperative organ function. This was most likely because such neuroprotective effects of magnesium may not be important in protecting hepatic IR injury.
This study had some limitations. First, the dose of magnesium (25 mg/kg) was relatively lower than in other studies. We anticipate that higher doses of magnesium would have protective effects on postoperative organ function. Second, we did not measure any cytokines or chemokines, such as tumor necrosis factor α, interleukin 1 and 6, which are the mediators of hepatic IR injury and give further information about IR injury. Third limitation is that the patients in this study were relatively healthy recipients because they were scheduled for elective LDLT. Also, every patient was controlled or supported with drugs and fluid administration to maintain stable hemodynamic and metabolic states during liver transplantation. These factors may contribute to observing no significant differences in postoperative organ function among the groups.
In conclusion, we determined that magnesium treatment is associated with reducing blood lactate levels after reperfusion of grafted liver. This result is consistent with those of previous studies [29,30] showing that magnesium administration inhibit lactate production after IR injury. However, there were no differences in the pH, base excess, PaO2, postoperative liver enzymes, and creatinine between the groups. Therefore, we recommend that further studies with optimized dose of magnesium are needed to investigate the precise and affirmative effects of magnesium on hepatic IR injury.
References
1. Busuttil RW, Tanaka K. The utility of marginal donors in liver transplantation. Liver Transpl. 2003; 9:651–663. PMID: 12827549.


2. Lee SG, Park KM, Hwang S, Lee YJ, Kim KH, Ahn CS, et al. Adult-to-adult living donor liver transplantation at the Asan Medical Center, Korea. Asian J Surg. 2002; 25:277–284. PMID: 12470999.


3. Teoh NC, Farrell GC. Hepatic ischemia reperfusion injury: pathogenic mechanisms and basis for hepatoprotection. J Gastroenterol Hepatol. 2003; 18:891–902. PMID: 12859717.


4. Fondevila C, Busuttil RW, Kupiec-Weglinski JW. Hepatic ischemia/reperfusion injury--a fresh look. Exp Mol Pathol. 2003; 74:86–93. PMID: 12710939.


5. Sakon M, Ariyoshi H, Umeshita K, Monden M. Ischemia-reperfusion injury of the liver with special reference to calcium-dependent mechanisms. Surg Today. 2002; 32:1–12. PMID: 11871810.


6. Christensen CW, Rieder MA, Silverstein EL, Gencheff NE. Magnesium sulfate reduces myocardial infarct size when administered before but not after coronary reperfusion in a canine model. Circulation. 1995; 92:2617–2621. PMID: 7586364.


7. Woods KL, Fletcher S, Roffe C, Haider Y. Intravenous magnesium sulphate in suspected acute myocardial infarction: results of the second Leicester Intravenous Magnesium Intervention Trial (LIMIT-2). Lancet. 1992; 339:1553–1558. PMID: 1351547.


8. Lee EJ, Lee MY, Chang GL, Chen LH, Hu YL, Chen TY, et al. Delayed treatment with magnesium: reduction of brain infarction and improvement of electrophysiological recovery following transient focal cerebral ischemia in rats. J Neurosurg. 2005; 102:1085–1093. PMID: 16028768.


9. Kaplan S, Ulus AT, Tütün U, Aksöyek A, Ozgencil E, Saritas Z, et al. Effect of Mg2SO4 usage on spinal cord ischemia-reperfusion injury: electron microscopic and functional evaluation. Eur Surg Res. 2004; 36:20–25. PMID: 14730219.
10. Fawcett WJ, Haxby EJ, Male DA. Magnesium: physiology and pharmacology. Br J Anaesth. 1999; 83:302–320. PMID: 10618948.
11. Nishimura A, Hakamada K, Narumi S, Totsuka E, Toyoki Y, Ishizawa Y, et al. Intraoperative blood lactate level as an early predictor of initial graft function in human living donor liver transplantation. Transplant Proc. 2004; 36:2246–2248. PMID: 15561207.


12. Pugh RN, Murray-Lyon IM, Dawson JL, Pietroni MC, Williams R. Transection of the oesophagus for bleeding oesophageal varices. Br J Surg. 1973; 60:646–649. PMID: 4541913.


13. Policy 3.6, Allocation of livers. United Network for Organ sharing. Available from http://www.unos.org.
14. Freeman RB Jr, Wiesner RH, Harper A, McDiarmid SV, Lake J, Edwards E, et al. The new liver allocation system: Moving toward evidence-based transplantation policy. Liver Transpl. 2002; 8:851–858. PMID: 12200791.


15. Grenache DG, Parker C. Integrated and automatic mixing of whole blood: An evaluation of a novel blood gas analyzer. Clin Chim Acta. 2007; 375:153–157. PMID: 16905126.


16. Karon BS, Scott R, Burritt MF, Santrach PJ. Comparison of lactate values between point-of-care and central laboratory analyzers. Am J Clin Pathol. 2007; 128:168–171. PMID: 17580286.


17. Suen WW, Ridley B, Blakney G, Higgins TN. Comparison of lactate, bilirubin and hemoglobin F concentrations obtained by the ABL 700 series blood gas analyzers with laboratory methods. Clin Biochem. 2003; 36:103–107. PMID: 12633758.


18. Theodoraki K, Arkadopoulis N, Fragulidis G, Voros D, Karapanos K, Markatou M, et al. Transhepatic lactate gradient in relation to liver ischemia/reperfusion injury during major hepatectomies. Liver Transpl. 2006; 12:1825–1831. PMID: 17031827.


19. De Gasperi A, Mazza E, Corti A, Zoppi F, Prosperi M, Fantini G, et al. Lactate blood levels in the perioperative period of orthotopic liver transplantation. Int J Clin Lab Res. 1997; 27:123–128. PMID: 9266283.


20. Kreisberg RA. Lactate homeostasis and lactate acidosis. Ann Intern Med. 1980; 92:227–237. PMID: 6766289.
21. Katayama Y, Fukuchi T, Mc Kee A, Terashi A. Effect of hyperglycemia on pyruvate dehydrogenase activity and energy metabolites during ischemia and reperfusion in gerbil brain. Brain Res. 1998; 788:302–304. PMID: 9555066.


22. Krenn CG, De Wolf AM. Current approach to intraoperative monitoring in liver transplantation. Curr Opin Organ Transplant. 2008; 13:285–290. PMID: 18685319.


23. Diaz J, Acosta F, Parrilla P, Sansano T, Tornel PL, Robles R, et al. Serum ionized magnesium monitoring during orthotopic liver transplantation. Transplantation. 1996; 61:835–837. PMID: 8607193.


24. Bennett MW, Webster NR, Sadek SA. Alterations in plasma magnesium concentrations during liver transplantation. Transplantation. 1993; 56:859–861. PMID: 8212207.


25. Ranasinghe DN, Mallett SV. Hypomagnesaemia, cardiac arrhythmias and orthotopic liver transplantation. Anaesthesia. 1994; 49:403–405. PMID: 8209980.


26. Choi JH, Lee J, Park CM. Magnesium therapy improves thromboelastographic findings before liver transplantation: a preliminary study. Can J Anaesth. 2005; 52:156–159. PMID: 15684255.


27. Kramer JH, Misik V, Weglicki WB. Magnesium-deficiency potentiates free radical production associated with postischemic injury to rat hearts: vitamin E affords protection. Free Radic Biol Med. 1994; 16:713–723. PMID: 8070674.


28. Dickens BF, Weglicki WB, Li YS, Mak IT. Magnesium deficiency in vitro enhances free radical-induced intracellular oxidation and cytotoxicity in endothelial cells. FEBS Lett. 1992; 311:187–191. PMID: 1397313.


29. Günther T, Vormann J, Höllriegl V. Effects of magnesium and iron on lipid peroxidation in cultured hepatocytes. Mol Cell Biochem. 1995; 144:141–145. PMID: 7623785.


30. Bariskaner H, Ustun ME, Ak A, Yosunkaya A, Ulusoy HB, Gurbilek M. Effects of magnesium sulfate on tissue lactate and malondialdehyde levels after cerebral ischemia. Pharmacology. 2003; 68:162–168. PMID: 12784088.


31. Lang-Lazdunski L, Heurteaux C, Dupont H, Widmann C, Lazdunski M. Prevention of ischemic spinal cord injury: comparative effects of magnesium sulfate and riluzole. J Vasc Surg. 2000; 32:179–189. PMID: 10876221.


Fig. 1
Changes in magnesium concentration during living donor liver transplantation. The magnesium concentrations significantly increased after magnesium administration in Normal-MG and Low-MG group. Preop: preoperative 1 day, Anhepatic + 10 min: 10 minute after the beginning of anhepatic phase, Reperfusion + 10 min: 10 minute after reperfusion, POD 1: postoperative 1 day. The normal range of serum magnesium concentration is 0.70 to 1.10 mmol/L. Normal-NS: NS group with preoperative magnesium levels ≥ 0.70 mmol/L, Normal-MG: magnesium-treated group with preoperative magnesium levels ≥ 0.70 mmol/L, Low-NS: NS group with preoperative magnesium levels < 0.70 mmol/L, Low-MG: magnesium-treated group with preoperative magnesium levels < 0.70 mmol/L. Data are the mean ± SD. *P < 0.05 compared with Normal-NS group, †P < 0.05 compared with Low-NS group. ‡P < 0.05 compared with Preop by Bonferroni correction.
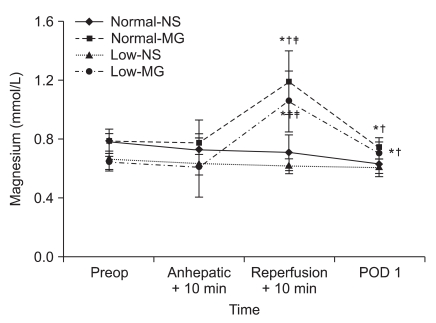
Fig. 2
Changes in blood lactate levels before and after reperfusion in living donor liver transplantation. Significantly higher blood lactate levels were observed in the Low-NS group compared to magnesium-treated groups after reperfusion. Normal-NS: NS group with preoperative magnesium levels ≥ 0.70 mmol/L, Normal-MG: magnesium-treated group with preoperative magnesium levels ≥ 0.70 mmol/L, Low-NS: NS group with preoperative magnesium levels < 0.70 mmol/L, Low-MG: magnesium-treated group with preoperative magnesium levels < 0.70 mmol/L. Data are the mean ± SD. *P < 0.05 compared with Normal-MG group, †P < 0.05 compared with Low-MG group.
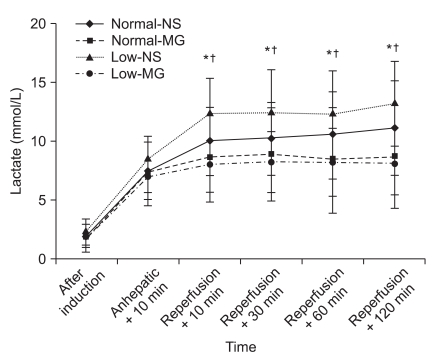
Fig. 3
Changes of physiological parameters before and after reperfusion in living donor liver transplantation. (A) pH, (B) PaO2, (C) MAP, (D) Base excess. After reperfusion, pH values were significantly decreased compared to anhepatic + 10 min. No significant difference was observed between the groups. Data are the mean ± SD. Anhepatic + 10 min: 10 min after the beginning of the anhepatic phase, Reperfusion + 10, 30, 60 and 120 min: 10, 30, 60 and 120 min after reperfusion, MAP: mean arterial pressure. Normal-NS: NS group with preoperative magnesium levels ≥ 0.70 mmol/L, Normal-MG: magnesium-treated group with preoperative magnesium levels ≥ 0.70 mmol/L, Low-NS: NS group with preoperative magnesium levels < 0.70 mmol/L, Low-MG: magnesium-treated group with preoperative magnesium levels < 0.70 mmol/L. *P < 0.05 compared with Anhepatic + 10 min by Bonferroni correction.
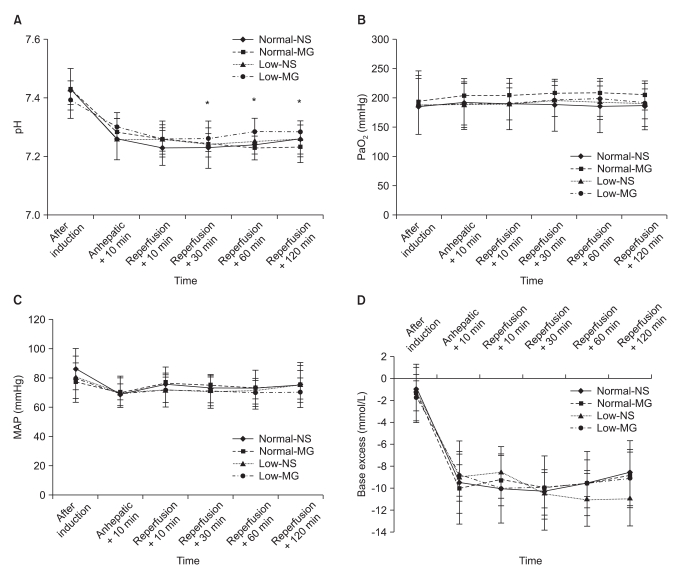
Fig. 4
Changes of serum AST, ALT, total bilirubin and creatinine levels before and after living donor liver transplantation. All parameters were observed to significantly increase at POD 1 and decrease at POD 5, but there was no difference between the groups. Normal-NS: NS group with preoperative magnesium levels ≥ 0.70 mmol/L, Normal-MG: magnesium-treated group with preoperative magnesium levels ≥ 0.70 mmol/L, Low-NS: NS group with preoperative magnesium levels < 0.70 mmol/L, Low-MG: magnesium-treated group with preoperative magnesium levels < 0.70 mmol/L. AST: aspartate aminotransferase, ALT: alanine aminotransferase. Preop: preoperative value (one day before surgery), POD 1: postoperative 1 day, POD 5: postoperative 5 day. *P < 0.05 when compared with Preop by Bonferroni correction.
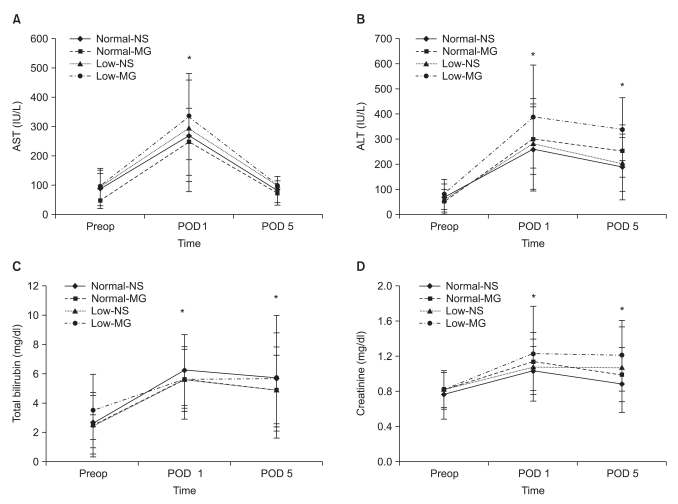
Table 1
Demographic Data and Clinical Profiles Including Transfusion Amounts in Living Donor Liver Transplantation
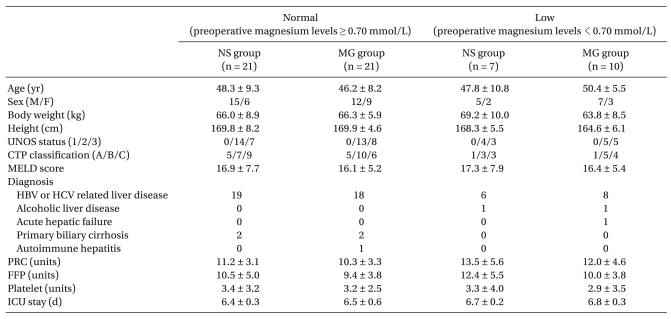
Values are expressed as mean ± SD or number of patients. UNOS status: United Network for Organ Sharing status, CTP classification: Child-Turcotte-Pugh classification, MELD: Model for End-stage Liver Disease, HBV: Hepatitis B virus, HCV: Hepatitis C virus, PRC: packed red cell, FFP: fresh frozen plasma, ICU stay: length of stay in intensive care unit. No significant differences among the groups.