Abstract
Background
Emergence agitation after sevoflurane anesthesia in children can be prevented by midazolam. Alternative splicing of the GABAA receptor changes with age. Therefore, we hypothesized that alternative splicing of the γ2 subunit affects the GABA current when applying sevoflurane and midazolam.
Methods
We performed the whole-cell patch clamp technique on human embryonic kidney 293 cells that were transfected with α1β2γ2L or α1β2γ2S. The concentration-response relations were recorded for midazolam and sevoflurane, and the co-application responses were measured at concentrations of 1.5 nM, 15 nM and 300 nM of midazolam and 0.5%, 2.0% and 4.0% of sevoflurane. Each GABA current was compared with that produced by 5 µM of GABA.
Results
The concentration-response relationships for midazolam and sevoflurane were dose-dependent without any differences between the α1β2γ2L and α1β2γ2S subtypes. 1.5 nM and 15 nM of midazolam did not significantly enhance the current after treatment with 0.5% sevoflurane for both subtypes. The current after treatment with 2.0% sevoflurane was enhanced by 1.5 nM midazolam for the α1β2γ2S subtype, but not for the α1β2γ2L subtype. In the case of 2.0% sevoflurane with 15 nM of midazolam, and 4.0% sevoflurane with 300 nM of midazolam, the GABA currents were significantly enhanced for both subtypes.
Conclusions
These results show that the difference in the γ2 subunit cannot explain the emergence agitation after sevoflurane anesthesia in children in vitro. This suggests that co-application of sevoflurane and midazolam enhances the GABA current according to the alternative splicing of the γ2 subunit and the concentration of both drugs.
The GABAA receptor mediates rapid inhibitory action by using GABA, which is a major inhibitory neurotransmitter of the central nervous system. Activation of the GABAA receptor induces the opening of chloride channels and this increases the inward chloride current. The hyperpolarization of the membrane of neurons then decreases the post-synaptic action potential of the neuron [1].
As the GABAA receptor has specific binding sites for GABA, barbiturate, benzodiazepines and the anesthetic steroids, which induces sedation, hypnosis, amnesia or anesthesia through their binding, the GABAA receptor is considered to be a major target protein of anesthesia [2].
The GABAA receptor is a complex of 5 subunits of 19 different subunits (α, β, γ, δ, ε, π, θ, ρ, etc.) in mammals. Multiple combinations from the different subunits make several subtypes of GABAA receptor. Most GABAA receptors consist of two α subunits, two β subunits and a γ subunit. The complexity of GABAA receptors is still under investigation and there exits the possibility of new combinations of subunits. Each combination of different subunits has unique physiologic characteristics and the drug responses are different from each other [3].
The γ2 subunits of the GABAA receptor exist as a long type (γ2L) and a short type (γ2S) by alternative splicing of RNA. The γ2L subunits have another 8 amino acids (LLRMFSFK) in the intracellular loop and the phosphorylation by protein kinase C (PKC) occurs in this region (Ser343) [4].
It seems that the agents binding to the γ2 subunit may have different effects according to the subtype. The total expression of the γ2 subunit is similar for all ages, but γ2L/γ2S increases by the up-regulation of the γ2L subunit and γ2L becomes dominant in mature synapses according to age [5,6].
The distribution and pattern of GABAA receptors are various according to the region of the central nervous system and the type of neuron. This is also true for γ2L and γ2S [7,8]. The most common pattern of GABAA receptor is the α1β2γ2 type, which is 43% of all the GABAA receptors [9]. This implies that the diversity of the subtype variants and the distribution of GABAA receptors may affect the effect of anesthetics and induce different effects from subject to subject.
Midazolam is a popular sedative and sevoflurane is a most commonly used inhalational anesthetic. It is known that these drugs show their sedative, hypnotic or anesthetic actions by binding to GABAA receptors. The GABAA receptor has the loci for binding to benzodiazepine, barbiturate and steroids. Midazolam activates the GABAA receptor by augmenting the binding of GABA to the receptor and midazolam directly activates the GABAA receptor at a high concentration. The γ2 subunit is essential for binding benzodiazepine to the GABAA receptor [10].
Sevoflurane is a volatile anesthetic that was developed relatively recently, and it is now widely used and preferred for clinical anesthesia, and especially for pediatric anesthesia. Sevoflurane has been reported to potentiate GABA-induced currents at the GABAA receptor. Sevoflurane enables the rapid induction and quick recovery after inhalational anesthesia when using it because sevoflurane possesses several favorable properties, including low blood and tissue solubility, non-pungency and limited cardiorespiratory depression, which may make sevoflurane desirable for use in infants and children [11]. The quick recovery from sevoflurane is likely to be accompanied by emergence agitation, which often occurs after administering sevoflurane in children even when sufficient analgesia is provided [12,13].
One of the trials to reduce the unpleasant effects during emergence is to use midazolam as premedication. It has been reported that premedication with oral midazolam is effective for decreasing the occurrence of emergence agitation without delaying the discharge from the post-anesthesia care unit and so this is safe and suitable for outpatient surgery [14]. However, the mechanism is still not clear, and it is not known whether sevoflurane and midazolam have the interaction at the level of the GABAA receptor.
The mechanism of agitation was described in the model of Sachdev and Kruk as the result of decreased inhibitory signals to the globus pallidus interna/substantia nigra pars or the disinhibition of the thalamocortical neurons and brain stem neurons by the disturbance of neuronal circuits [15]. So, it is expected that the change of GABAA receptor activity may cause or reduce emergence agitation.
We postulated that alternative splicing of γ2 subunit is related with the occurrence of emergence agitation on the basis of the characteristics of midazolam, sevoflurane and the γ2 subunit; sevoflurane binds to GABAA receptor, a benzodiazepine like midazolam prevents emergence agitation and binds to the α and γ subunits of the GABAA receptor, and alternative splicing of the γ2 subunit is different according to age. Therefore, we performed the whole-cell patch clamp to the α1β2γ2L and α1β2γ2S GABAA receptors that are expressed on human embryonic kidney (HEK) 293 cells with using midazolam and/or sevoflurane.
HEK 293 cells are suitable for the transient expression of GABAA receptors and electrophysiological study [16]. Culturing of the HEK 293 cells and expressing GABAA cDNA in the HEK 293 cells were performed as previously described [17].
The HEK 293 cells (CRL-1573; American Type Culture Collection) were cultured on glass cover slips in a solution containing minimum essential medium (MEM) supplemented with 10% heated fetal bovine serum, glutamine (4 mM), penicillin G (100 U/ml) and streptomycin sulfate (100 µg/ml).
Human γ2S cDNA was made from human γ2L cDNA (JC Biotech, Seoul, Korea) by deletion mutagenesis and its sequence was confirmed by polymerase chain reaction.
The γ-aminobutyric acid type A receptor complementary DNAs (the human γ2L or γ2S cDNA, the rat α1 cDNAs and the rat β2 cDNAs: the rat α1 and β2 cDNAs were generous gifts from Dr. Werner Sieghart, Professor of Biochemistry and Molecular Pharmacology, and Head of the Department of Biochemistry and Molecular Biology, Center for Brain Research, Medical University Vienna, Vienna, Austria) were expressed in the human embryonic kidney (HEK) 293 cells along with the cDNA of green fluorescent protein as previously described. For the transient expression of GABAA receptors, the cells were transfected using the CaPO4 precipitation technique as previously described [18].
The GABAA receptor cDNAs and green fluorescent protein cDNA were precipitated for 60 min at room temperature in 65 µl of distilled water that contained 8 µl of 2.5 M CaCl2, 750 µl of 50 mM N, N-bis [2-hydroxyethyl]-2-aminoethanesulfonic acid and 5 µg of each cDNA. The mixture was added to the cells grown on the cover slips. The cDNA was in contact with the HEK cells for 24 h in an atmosphere containing 3% CO2 (37℃) before being removed and replaced with fresh culture medium in an atmosphere of 5% CO2 (37℃). The transfected cells were cultured on cover slips for 48-72 h after cDNA removal. The cover slips were transferred to a 35 mm culture dish 1 h before the whole-cell patch clamp.
A cover slip was mounted on the recording chamber and it was continuously perfused with extracellular solution (145 mM NaCl, 3 mM KCl, 1.5 mM CaCl2, 1 mM MgCl2, 6 mM D-glucose and 10 mM HEPES/NaOH adjusted to pH 7.4) at a rate of 10 ml/min.
The whole cell patch clamp recordings from the fluorescing HEK 293 cells (voltage clamped at -60 mV) were made using a EPC 10 USB amplifier (HEKA Electronik, Lambrecht, Germany) under a fluorescence microscope (Olympus IX71; Olympus Co. Ltd., Tokyo, Japan) at room temperature (22℃) as described previously.
The patch pipette was made by using a PC-10 puller (Narishige Co., Tokyo, Japan) and a glass capillary tube (outer diameter: 1.5 mm, Harvard Apparatus Ltd., Edenbridge, Kent, UK). The resistance of the patch pipette was 4-6 M when it was filled with intracellular solution (145 mM N-methyl-D-glucamine HCl, 5 mM HEPES/KOH and 0.1 mM CaCl2, pH 7.2).
The expression of GABA cDNA was confirmed by the development of intrinsic current, which was measured from the fluorescing HEK 293 cells, but not from the non-fluorescing cells by the application of GABA (10 µM) and inhibition of the intrinsic current by the application of bicuculline (10 µM), which is an antagonist of the GABAA receptor.
In addition to the continuous bath perfusion with extracellular solution, solutions including GABA, sevoflurane or midazolam were applied to the cells for 3 s at a rate of 10 ml/min using a manual valve controller. The solutions were rinsed away for at least 1 min to minimize the residual effect of other solutions.
Stock solutions of GABA and midazolam (Midacom®, Myungmoon Pharm., Korea) were diluted in the extracellular solutions shortly before use. The sevoflurane (Sevorane®, Abbott Korea, Korea) solutions were prepared by a sevoflurane vaporizer (Vapor 2000®, Draeger Medical, Germany). The extracellular medium was bubbled with a vaporized sevoflurane and oxygen mixture. The concentration of sevoflurane was measured by an anesthetic gas monitor (Vamos®, Draeger Medical, Germany). The agents without specific notification were purchased from Sigma (St. Louis, MO, USA).
The concentration-response curve of GABA was obtained by applying 6 different concentrations of GABA solutions in a row (0.1-1,000 µM) to the GABAA receptors for 3s after the cells were superfused with extracellular medium, followed by a return to extracellular medium for at least 1 min before any subsequent GABA application. As the currents for 5 µM of GABA were similar to 20% of the maximum response in both subtypes, 5 µM of GABA was used for comparison of each of the currents made by the midazolam and/or sevoflurane.
The current for 5 µM of GABA was measured as a control current for each cell before the application of sevoflurane and/or midazolam. The current for each drug was divided by the control current, and the ratio was used for comparison.
The concentration-response curves of midazolam and sevoflurane were obtained by the application of 4 different concentrations of midazolam (15-10,000 nM) or sevoflurane (0.5-8.0%) in a row for 3 s together with 5 µM of GABA for both subtypes and the 3 sets of concentrations of those were obtained for comparison. To determine the interaction of midazolam and sevoflurane, midazolam and sevoflurane were cross-applied together with 5 µM of GABA at the concentration of 1.5 nM (minimum response), 15 nM and 300 nM (maximum response) of midazolam and at the concentration of 0.5%, 2.0% (1 MAC) and 4% (2 MAC) of sevoflurane for both subtypes.
Origin 6.1 (MicroCal, Northampton, USA) and Microsoft Excel 2007 (Microsoft, Redmond, USA) were used for the statistical analysis. All the data is expressed as means ± standard errors. The measured currents of each agent were converted to the ratio of the measured current of each agent to the current measured by GABA 5 µM. Student's t-test was used for comparing the differences of each ratio. P values < 0.05 were considered statistically significant.
There were no current changes according to GABA (10 µM) or no fluorescences in the non-transfected cells (Fig. 1). Yet the transfected cells showed green fluorescences and inward current due to GABA (10 µM) for both subunits (Fig. 1). Application of bicuculline (10 µM) decreased the current by 10.3 ± 4.5% for the α1β2γ2L subtype (n = 13) and by 7.5 ± 3.2% for the α1β2γ2S subtype (n = 6) (Fig. 2). The responses to GABA were recovered by the superfusion of extracellular solution for 3 min. This means that α1β2γ2L cDNA or α1β2γ2S cDNA was successfully transfected to the HEK 293 cells.
The responses to GABA increased in a concentration-dependent manner for both the α1β2γ2L and α1β2γ2S subtypes. The EC50 of the α1β2γ2L subtype was 37.2 µM (n=53) (Fig. 3) and the EC50 of the α1β2γ2S subtype was 17.1 µM (n = 66) (Fig. 4). The maximum responses were 3.7 ± 0.4 nA for the α1β2γ2L subtype and 3.1 ± 0.6 nA for the α1β2γ2S subtype without a statistically significant difference. The EC20 was 4.5 µM and 4.1 µM for α1β2γ2L and α1β2γ2S, respectively.
Midazolam increased the current in response to GABA (5 µM) for both subtypes, and this was dependent on the increase of its concentration (Fig. 5 and 6). There were no differences of the EC50 of midazolam (249 nM for the α1β2γ2L subtype (n = 37) and 211 nM for the α1β2γ2S subtype (n = 34)) or for the maximum responses (303 ± 35% for the α1β2γ2L subtype and 329 ± 33% for the α1β2γ2S subtype).
The response to GABA (5 µM) was increased depending on the concentration of sevoflurane for both subtypes (Fig. 7 and 8). The EC50 of sevoflurane was 4.8% for the α1β2γ2L subtype (n = 20) and 5.2% for the α1β2γ2S subtype (n = 15), and the maximum response was 368 ± 87% and 412 ± 103% for both types, respectively. The difference was not significant. This means there were no differences according to the subtypes for midazolam and sevoflurane.
We measured the cross-responses of 1.5 nM and 15 nM of midazolam and 0.5% and 2.0% of sevoflurane. The response of 300 nM of midazolam to 4.0% of sevoflurane was also measured.
There was no effect of 1.5 nM of midazolam to 0.5% of sevoflurane for both the α1β2γ2L subtype (n = 4) and the α1β2γ2S subtype (n = 4). The ratio of the current to the control current was 136% and 135%, respectively (Fig. 9A). 15 nM of midazolam did not affect the response of 0.5% of sevoflurane either (n = 5 and 3). The ratio of the current to the control current was 194% and 200%, respectively (Fig. 9B). There was a significant difference for the co-application of 1.5 nM of midazolam and 2.0% of sevoflurane (Fig. 10A). Midazolam increased the response of sevoflurane for the α1β2γ2S subtype (n = 4), but not for the α1β2γ2L subtype (n = 6). The ratio was 227% to 154%. There was significant increases of the response to 2.0% of sevoflurane and 15 nM of midazolam for both subtypes without any differences between the α1β2γ2L subtype (n = 6, 225%) and the α1β2γ2S subtype (n = 3, 258%) (Fig. 10B).
The response of 300 nM of midazolam and 4.0% of sevoflurane showed significant increases of the GABA current in the cells with both the α1β2γ2L subtype (n = 4, 369%) and the α1β2γ2S subtype (n = 4, 355%) but there was no significant difference between the two subtypes (Fig. 11).
This means that 1.5 nM of midazolam inhibited the GABA current according to 2.0% of sevoflurane by affecting the phosphorylation of protein kinase C in the α1β2γ2L subtype.
The purpose of this study is to determine the difference of the GABA currents by the interaction of sevoflurane and midazolam at the GABAA receptor with the γ2L or γ2S subunit and whether the currents according to sevoflurane and/or midazolam are influenced by the difference of the γ2 subunit. The results showed that the GABA currents according to sevoflurane could be partially modulated by midazolam.
The induction of general anesthesia for children has greatly improved since sevoflurane was introduced. Sevoflurane enabled easy, smooth and rapid inhalational anesthesia for children because it can be inhaled at the high concentration with a non-pungent odor and there is less irritability of the airway even in an awake condition.
Emergence agitation is described that the patients are irrationally excited, agitated, restless, combative, frightened and non-cognizant, and the patients refuse parental care. Sometimes these patients have needed physical restraints [19]. This agitation is usually a self-limiting condition that subsides in an hour without any sequels or memory, but this makes the care of the patient difficult in the post-anesthesia recovery room. The physical over activity can be harmful to the patients and additional medical intervention with sedatives can prolong the stay in the post-anesthesia care unit [20]. The mechanism of this phenomenon has not been clearly explained, but there have been several theories and one of them is the change of activity of the GABAA channels in the central nervous system [15]. Clinical trials have been performed to alleviate this activity such as premedication with sedatives and regional anesthesia. Among them, premedication with a benzodiazepine like midazolam has been reported to be safe and useful [14]. Midazolam has the effects of amnesia, unconsciousness and anxiolysis, it has a broad therapeutic range and it causes less depression of the cardiovascular system. Its properties have enabled midazolam to become the most popular sedative for anesthesia. Yet the suppression of respiration and cognitive function has limited its use [21].
The long-term maintenance of a whole cell patch clamp after midazolam pretreatment and before the application of sevoflurane application, as in clinical practice, was difficult, so that we applied both drugs to the cells at the same time. Therefore, we cannot exclude the possibility of molecular and pharmacological changes of the GABAA receptors during the pretreatment with midazolam when interpreting our results.
Both midazolam and sevoflurane activate GABAA receptors, that is, they augment the binding of GABA to the receptor and facilitate the opening of chloride channels [10,22,23]. The γ subunit is essential for the binding of benzodiazepine to the GABAA receptor. Benzodiazepine does not increase the membrane potentials activated by GABA in the GABAA receptor without the γ subunit [16,24]. The γ2 subunit plays an important role in postsynaptic anchoring and intracellular incorporation of the GABAA receptor [25]. The RNA of the γ2 subunit of the GABAA receptor is spliced alternatively into the long (γ2L) form and the short (γ2S) form, and each has different pharmacological properties. A mutation of the γ2 subunit of the GABAA receptor is closely associated with familial idiopathic epilepsy [26]. A marked reduction of the γ2S subunit and the associated relative increase of the γ2L subunit in the prefrontal cortex results in functionally less active GABAA receptors and this has severe consequence for the cortical integrative function, and it leads to schizophrenia [27]. In contrast, others have reported that the anxiety and sensitivity to benzodiazepine were higher in knockout mice that lacked the long splice than that in the control group [28].
There is a phosphorylation site in the γ2L subunit. Phosphorylation plays an important role in the control of ligand-gated ion channels. Phosphorylation by PKC decreases the inward currents activated by GABA and then this inhibits GABAA receptor function [4]. Both γ2L and γ2S have the same phosphorylation site at Ser327, but the γ2L subunit has an additional phosphorylation site at Ser343 in an alternative splicing area [29]. PKC decreases the activity of the GABAA receptor at both sites, Ser327 and Ser343, by phosphorylation, yet the suppression of the receptor is greater at Ser343 [30]. This is because the alternative splicing makes conformational changes in the intracellular loop, where Ser343 exists, between the 3rd and 4th transmembrane domains.
The difference in the alternative splicing and the additive effects of midazolam and sevoflurane in the γ2S form and γ2L form in our study show the possibility of the individual diversity in the responses to both drugs.
While recovery is occurring from sevoflurane anesthesia, the concentration of sevoflurane gradually decreases to zero. We postulated that the emergence agitation occurs when gradual recovery from the inhibition of the central nervous system by the GABA pathway during this process is interrupted in certain subjects and this interruption can be overcome by the administration of benzodiazepine to partially re-activate the GABA pathway, with the agitation being suppressed. We could not reveal that the γ2L dominant property of children was closely related with emergence agitation after sevoflurane anesthesia because we failed to show the difference of the responses to only sevoflurane in both subtypes. Yet it is expected that pretreatment with midazolam additionally activates the GABAA receptors and this minimizes the emergence agitation from sevoflurane anesthesia in the relatively γ2S-dominant subjects, but not in the relatively γ2L-dominant subjects.
In conclusion, the co-application of midazolam and sevoflurane showed different results according to the type of γ2 subunit in the GABAA receptors. Further studies are needed in order to reveal the differences of drug responses for each subject according to the γ2L/γ2S ratio and to individualize the drug dosages when these drugs are used for anesthesia and sedation.
References
1. Zimmerman SA, Jones MV, Harrison NL. Potentiation of gamma-aminobutyric acidA receptor Cl- current correlates with in vivo anesthetic potency. J Pharmacol Exp Ther. 1994; 270:987–991. PMID: 7932211.
2. Macdonald RL, Olsen RW. GABAA receptor channels. Annu Rev Neurosci. 1994; 17:569–602. PMID: 7516126.


3. Sieghart W, Sperk G. Subunit composition, distribution and function of GABA(A) receptor subtypes. Curr Top Med Chem. 2002; 2:795–816. PMID: 12171572.


4. Machu TK, Firestone JA, Browning MD. Ca2+/calmodulin-dependent protein kinase II and protein kinase C phosphorylate a synthetic peptide corresponding to a sequence that is specific for the gamma 2L subunit of the GABAA receptor. J Neurochem. 1993; 61:375–377. PMID: 8390566.
5. Gutiérrez A, Khan ZU, Ruano D, Miralles CP, Vitorica J, De Blas AL. Aging-related subunit expression changes of the GABAA receptor in the rat hippocampus. Neuroscience. 1996; 74:341–348. PMID: 8865187.


6. Wang JB, Burt DR. Differential expression of two forms of GABAA receptor gamma 2-subunit in mice. Brain Res Bull. 1991; 27:731–735. PMID: 1661635.
7. Wisden W, Laurie DJ, Monyer H, Seeburg PH. The distribution of 13 GABAA receptor subunit mRNAs in the rat brain. I. Telencephalon, diencephalon, mesencephalon. J Neurosci. 1992; 12:1040–1062. PMID: 1312131.


8. Laurie DJ, Seeburg PH, Wisden W. The distribution of 13 GABAA receptor subunit mRNAs in the rat brain. II. Olfactory bulb and cerebellum. J Neurosci. 1992; 12:1063–1076. PMID: 1312132.


9. McKernan RM, Whiting PJ. Which GABAA-receptor subtypes really occur in the brain? Trends Neurosci. 1996; 19:139–143. PMID: 8658597.


10. McKernan RM, Wafford K, Quirk K, Hadingham KL, Harley EA, Ragan CI, et al. The pharmacology of the benzodiazepine site of the GABA-A receptor is dependent on the type of gamma-subunit present. J Recept Signal Transduct Res. 1995; 15:173–183. PMID: 8903939.
11. Lerman J, Sikich N, Kleinman S, Yentis S. The pharmacology of sevoflurane in infants and children. Anesthesiology. 1994; 80:814–824. PMID: 8024136.


12. Cravero JP, Beach M, Dodge CP, Whalen K. Emergence characteristics of sevoflurane compared to halothane in pediatric patients undergoing bilateral pressure equalization tube insertion. J Clin Anesth. 2000; 12:397–401. PMID: 11025242.


13. Aono J, Ueda W, Mamiya K, Takimoto E, Manabe M. Greater incidence of delirium during recovery from sevoflurane anesthesia in preschool boys. Anesthesiology. 1997; 87:1298–1300. PMID: 9416712.


14. Ko YP, Huang CJ, Hung YC, Su NY, Tsai PS, Chen CC, et al. Premedication with low-dose oral midazolam reduces the incidence and severity of emergence agitation in pediatric patients following sevoflurane anesthesia. Acta Anaesthesiol Sin. 2001; 39:169–177. PMID: 11840583.
15. Lindenmayer JP. The pathophysiology of agitation. J Clin Psychiatry. 2000; 61(Suppl 14):5–10. PMID: 11154018.
16. Pritchett DB, Sontheimer H, Shivers BD, Ymer S, Kettenmann H, Schofield PR, et al. Importance of a novel GABAA receptor subunit for benzodiazepine pharmacology. Nature. 1989; 338:582–585. PMID: 2538761.


17. Sebel LE, Richardson JE, Singh SP, Bell SV, Jenkins A. Additive effects of sevoflurane and propofol on gamma-aminobutyric acid receptor function. Anesthesiology. 2006; 104:1176–1183. PMID: 16732088.
18. Chen C, Okayama H. High-efficiency transformation of mammalian cells by plasmid DNA. Mol Cell Biol. 1987; 7:2745–2752. PMID: 3670292.


19. Wells LT, Rasch DK. Emergence "delirium" after sevoflurane anesthesia: a paranoid delusion? Anesth Analg. 1999; 88:1308–1310. PMID: 10357335.
20. Welborn LG, Hannallah RS, Norden JM, Ruttimann UE, Callan CM. Comparison of emergence and recovery characteristics of sevoflurane, desflurane, and halothane in pediatric ambulatory patients. Anesth Analg. 1996; 83:917–920. PMID: 8895263.


21. Short TG, Plummer JL, Chui PT. Hypnotic and anaesthetic interactions between midazolam, propofol and alfentanil. Br J Anaesth. 1992; 69:162–167. PMID: 1389820.


22. Khan ZU, Gutiérrez A, Miralles CP, De Blas AL. The gamma subunits of the native GABAA/benzodiazepine receptors. Neurochem Res. 1996; 21:147–159. PMID: 9182240.
23. Rüsch D, Forman SA. Classic benzodiazepines modulate the open-close equilibrium in alpha1beta2gamma2L gamma-aminobutyric acid type A receptors. Anesthesiology. 2005; 102:783–792. PMID: 15791108.
24. Sigel E, Baur R, Trube G, Möhler H, Malherbe P. The effect of subunit composition of rat brain GABAA receptors on channel function. Neuron. 1990; 5:703–711. PMID: 1699569.


25. Kneussel M. Dynamic regulation of GABA(A) receptors at synaptic sites. Brain Res Brain Res Rev. 2002; 39:74–83. PMID: 12086709.


26. Baulac S, Huberfeld G, Gourfinkel-An I, Mitropoulou G, Beranger A, Prud'homme JF, et al. First genetic evidence of GABA(A) receptor dysfunction in epilepsy: a mutation in the gamma2-subunit gene. Nat Genet. 2001; 28:46–48. PMID: 11326274.
27. Huntsman MM, Tran BV, Potkin SG, Bunney WE Jr, Jones EG. Altered ratios of alternatively spliced long and short gamma2 subunit mRNAs of the gamma-amino butyrate type A receptor in prefrontal cortex of schizophrenics. Proc Natl Acad Sci U S A. 1998; 95:15066–15071. PMID: 9844016.
28. Quinlan JJ, Firestone LL, Homanics GE. Mice lacking the long splice variant of the gamma 2 subunit of the GABA(A) receptor are more sensitive to benzodiazepines. Pharmacol Biochem Behav. 2000; 66:371–374. PMID: 10880692.
29. Whiting P, McKernan RM, Iversen LL. Another mechanism for creating diversity in gamma-aminobutyrate type A receptors: RNA splicing directs expression of two forms of gamma 2 phosphorylation site. Proc Natl Acad Sci U S A. 1990; 87:9966–9970. PMID: 1702226.


30. Krishek BJ, Xie X, Blackstone C, Huganir RL, Moss SJ, Smart TG. Regulation of GABAA receptor function by protein kinase C phosphorylation. Neuron. 1994; 12:1081–1095. PMID: 8185945.


Fig. 1
The HEK 293 cells with α1β2γ2L and α1β2γ2S GABAA receptors. The HEK293 cell without fluorescence shows no inward current. The inward currents are reduced after the application of bicuculline (10 µM) and they reappear after washout of bicuculline.
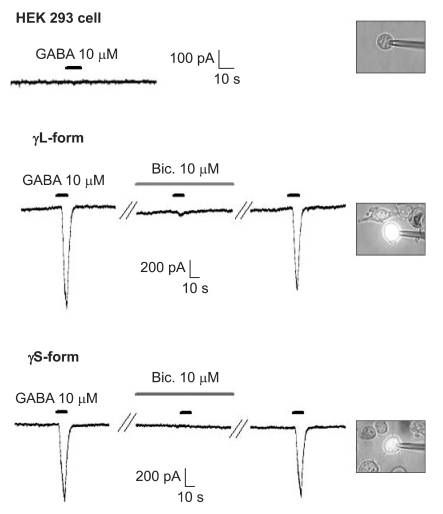
Fig. 2
Reduction of the GABAA receptor response after the application of bicuculline (10 µM). This reduction is reversed after washout of bicuculline. *P value < 0.05, when compared to the currents by GABA (10 µM).
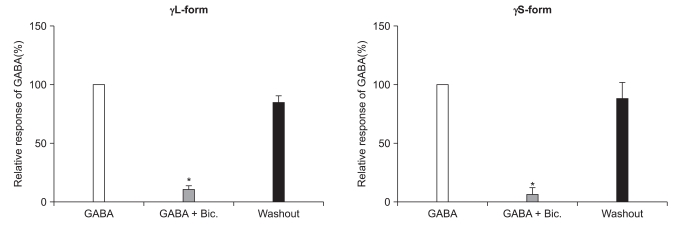
Fig. 3
Dose-response curve to GABA for α1β2γ2L GABAA receptor. The response is concentration-dependent. The data is expressed as means ± SEMs (6, 6, 13, 16, 8 and 4 cells were used at the concentrations of 1, 3, 5, 10, 50 and 200 µM, respectively).
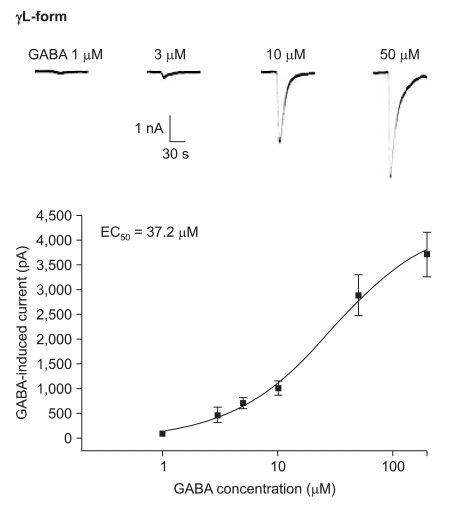
Fig. 4
Dose-response curve to GABA for α1β2γ2S GABAA receptor. The response is concentration-dependent. The data is expressed as means ± SEMs (8, 9, 18, 17, 11 and 3 cells were used at the concentration of 1, 3, 5, 10, 50 and 200 µM, respectively). *P value < 0.05, when compared with the γ2L form.
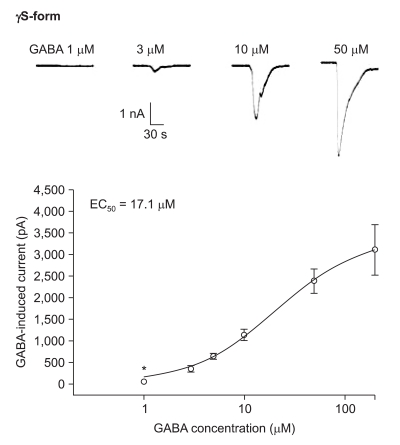
Fig. 5
Midazolam's potentiation of the GABA (5 µM)-evoked response for the α1β2γ2L GABAA receptor. The responses evoked by 5 µM GABA are potentiated by increasing concentrations of midazolam (15, 7, 8 and 7 cells were used at the concentration of 1.5, 15, 300 and 1,500 nM respectively).
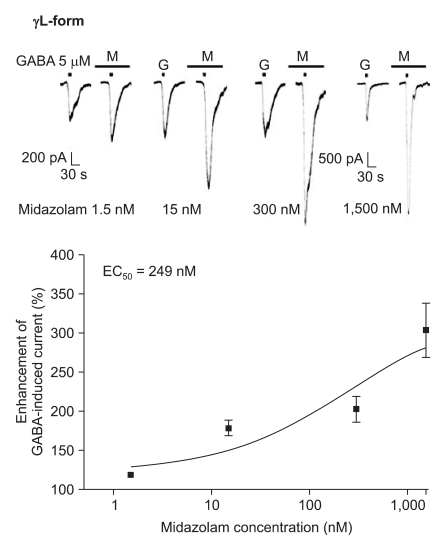
Fig. 6
Midazolam's potentiation of the GABA (5 µM)-evoked response for the α1β2γ2S GABAA receptor. The responses evoked by 5 µM GABA are potentiated by increasing concentrations of midazolam (13, 8, 6 and 7 cells were used at the concentration of 1.5, 15, 300 and 1,500 nM, respectively).
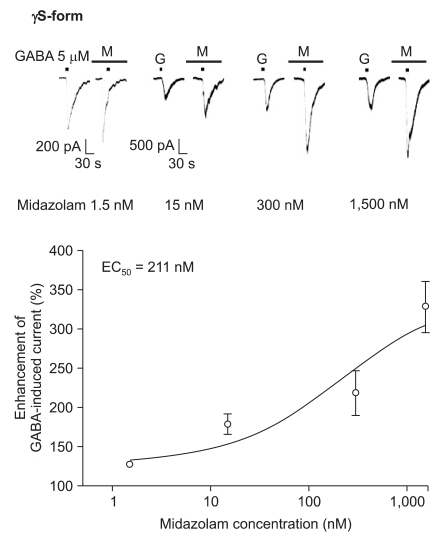
Fig. 7
Sevoflurane's potentiation of the GABA (5 µM)-evoked response for the α1β2γ2L GABAA receptor. The responses evoked by 5 µM GABA are potentiated by increasing concentrations of sevoflurane (4, 4, 4 and 8 cells were used at the concentration of 0.5, 2, 4 and 8%, respectively).
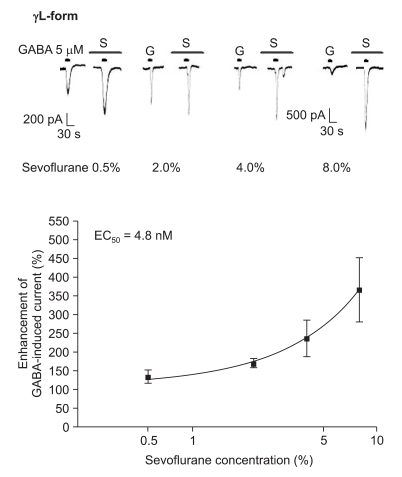
Fig. 8
Sevoflurane's potentiation of the GABA (5 µM)-evoked response for the α1β2γ2S GABAA receptor. The responses evoked by 5 µM GABA are potentiated by increasing concentrations of sevoflurane (3, 4, 2 and 6 cells were used at the concentration of 0.5, 2, 4 and 8%, respectively).
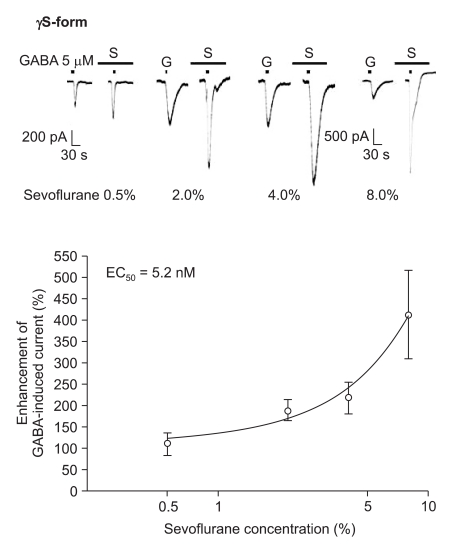
Fig. 9
(A) Sevoflurane (0.5%) enhancement of the currents evoked by co-application of midazolam (1.5 nM) and GABA (5 µM) for the α1β2γ22L and α1β2γ2S GABAA receptors. MDZ: midazolam, Sevo: sevoflurane. The data is expressed as means ± SEMs (4 cells for γ2L and 4 cells for γ2S were used.). (B) Sevoflurane (0.5%) enhancement of the currents evoked by the co-application of midazolam (15 nM) and GABA (5 µM) at the α1β2γ2L and α1β2γ2S GABAA receptors. MDZ: midazolam, Sevo: sevoflurane. The data is expressed as means ± SEMs (4 cells for γ2L and 3 cells for γ2S were used).
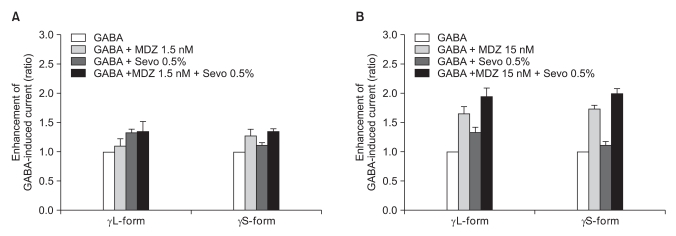
Fig. 10
(A) Sevoflurane (2.0%) enhancement of the currents evoked by the co-application of midazolam (1.5 nM) and GABA (5 µM) at the α1β2γ2L and α1β2γ2S GABAA receptors. MDZ: midazolam, Sevo: sevoflurane. The data is expressed as means ± SEMs (6 cells for γ2L and 4 cells for γ2S were used). (B) Sevoflurane (2.0%) enhancement of the currents evoked by the co-application of midazolam (15 nM) and GABA (5 µM) at the α1β2γ2L and α1β2γ2S GABAA receptors. MDZ: midazolam, Sevo: sevoflurane. The data is expressed as means ± SEMs (6 cells for γ2L and 3 cells for γ2S were used). *P value < 0.05, when compared with γL-form.
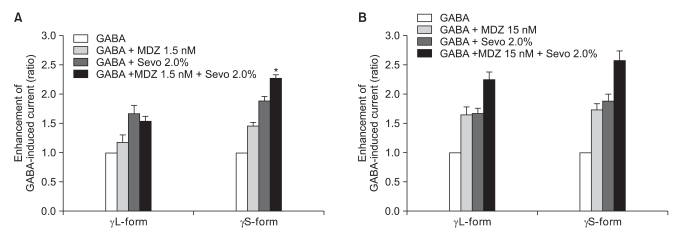