Abstract
Infections with multidrug-resistant organisms among patients in intensive care units (ICUs) are associated with high mortality. Among multidrug-resistant organisms, carbapenem-resistant Enterobacterales (CRE) harbor important pathogens for healthcare-associated infections, including pneumonia, bacteremia, and urinary tract infections. Risk factors for CRE colonization include underlying comorbid conditions, prior antibiotics exposure, prior use of healthcare facilities, device use, and longer ICU stay. The mortality rate due to invasive CRE infection is 22%–49%, and CRE colonization is associated with an approximately 10-fold increased risk of CRE infection. Infection control measures include hand hygiene, contact precautions, minimizing the use of devices, and environmental control. Additionally, implementing active surveillance of CRE carriage should be considered in ICU settings.
Patients in intensive care units (ICUs) are susceptible to nosocomial infections due to underlying diseases, device use, and prior antibiotics use. Nosocomial infections are associated with morbidity, mortality, and increased costs [1,2]. An international study conducted in 1,265 ICUs from 75 countries showed that 51% (7,087/13,796) of patients were considered having infection according to the definitions of the International Sepsis Forum, and 71% were receiving antibiotics. Additionally, the infection rate of ICU patients was related to disease severity, and infection was independently associated with a greater risk of hospital mortality (adjusted odds ratio, 1.51; 95% confidence interval, 1.36–1.68; P<0.001) [2].
ICU patients are at a high risk of colonization and infection caused by multidrug-resistant organisms (MDROs). Patients with extended ICU stays have higher rates of infection by resistant organisms [2]. MDROs, including methicillin-resistant Staphylococcus aureus, vancomycin-resistant enterococci (VRE), multi-drug resistant Acinetobacter baumannii (MRAB), and Enterobacterales that produce extended-spectrum β-lactamases (ESBL), are associated with ICU-related nosocomial infections [3]. Of these MDROs, Enterobacterales, an order of Gramnegative bacteria, harbor common pathogens for healthcare-associated infections, including pneumonia, bacteremia, and urinary tract infections. Recently, among MDROs, carbapenem-resistant Enterobacterales (CRE) have emerged as a threat for ICU patients [4] because of limited treatment options and high mortality rates [5]; therefore, CRE outbreaks, especially in the ICU, have become a critical issue. Several agents including ceftazidime-avibactam, ceftolozane-tazobactam, meropenem-vaborbactam, and imipenem-cilastatin-relebactam have been approved for clinical use [5], but these agents are not available in Korea. This study presents a review of the epidemiology, risk factors, clinical outcomes, and infection control of CRE in the ICU setting.
The Centers for Disease Control and Prevention (CDC) defines CRE as: (1) Enterobacterales that test resistant to at least one of the carbapenem antibiotics (i.e., minimum inhibitory concentrations of ≥ 4 µg/mL for doripenem, meropenem, and imipenem, and ≥ 2 µg/mL for ertapenem) or (2) Enterobacterales that produce a carbapenemase [6]. For Enterobacterales (Proteus species, Morganella spp., Providencia spp.) that may have intrinsic reduced susceptibilities to imipenem other carbapenem antibiotics should be used to test carbapenem resistance [6].
The “E” in CRE used to stand for the family Enterobacteriaceae but has been recently altered to the order Enterobacterales due to taxonomic changes [7]. If the older usage (Enterobacteriaceae) is to be persistently held, important genera like Proteus, Serratia, and Pantoea, despite their infrequent resistance to carbapenems, will be omitted from CRE.
One mechanism of resistance to carbapenem antibiotics in Enterobacterales is the production of carbapenemases, the enzymes that hydrolyze carbapenem antibiotics and are encoded by mobile genetic elements [8]. Approximately 30% of CRE are carbapenemase-producing CRE (CPE) that harbor the carbapenemases [9], which rapidly spread resistance [8].
The Ambler classification system, which is based on amino acid homology, shows four major classes of β-lactamases, viz., classes A, B, C, and D [10,11]. Classes A, C, and D β-lactamases share a serine residue in their active site (serine β-lactamases), while class B β-lactamases require zinc for their action (metallo-beta-lactamases, MBLs) [12,13]. Classes A, B, and D confer carbapenem resistance in Enterobacterales and have clinical importance for their role as healthcare-associated pathogens [5,12]. Clinically, the most significant class A carbapenemase is the Klebsiella pneumoniae carbapenemase (KPC) group [8]. Owing to the transmission from Klebsiella to other genera, KPC can be found in various Enterobacterales, including Escherichia coli, Citrobacter spp., Serratia marcescens, and Enterobacter spp. [12,14-17]. Clinically significant Class B carbapenemases are IMP (active on imipenem), Verona integron-encoded metallo-β-lactamase, and New Delhi metallo-β-lactamase (NDM) [10,12]. Class D carbapenemases are referred to as OXA carbapenemases due to their ability to hydrolyze oxacillin; OXA stands for oxacillinase [18]. There are many different variants or subtypes of carbapenemases, from KPC-1 to KPC-67, according to the order reported and amino acid substitutions [19-21].
The prevalence of CRE infection continues to increase globally [8]. In the United States, an active surveillance study showed that the overall annual incidence of CRE infection was 2.93 cases per 100,000 persons in seven U.S. metropolitan communities, and site-specific incidence rates ranged from 0.35 to 4.58 per 100,000 persons [22]. In a 2019 report, 13,100 cases of CRE infection were reported in the U.S., with 1,100 hospitalized patients deaths [23]. The first CPE reported was KPC-producing K. pneumoniae from North Carolina in 1996 [24], and by 2001, many reports of CPE from the northeastern U.S. had been published [25]. KPC-producing K. pneumoniae is mostly found in North America, Israel, Latin America, Italy, and Greece (Table 1) [8,25]. A CRE network study conducted in China in 2015 reported that the overall incidence of CRE infection was 4.05 per 100,000 patient-days (from 0.32 in Qinghai to 14.38 in Jiangsu), and 20.8% of CRE-infected patients were admitted to the ICU [26]. A study comprising active screening of CRE infections in hematology units in China reported that the CRE colonization rate in patients was 16.46% [27]. In a prospective cohort study of inpatients from the ICU and hematopoietic stem cell transplantation wards, the CRE colonization rate was 16.67% and KPC-producing K. pneumoniae was dominant [28]. National surveillance of CRE strains in China showed that KPC-2 and NDM were responsible for phenotypic resistance in 90% of CRE strains [29]. IMP-1, the first MBL, was reported in Japan in 1991 [30], and IMP is the carbapenemase found most abundantly in Japan [8,31]. A cross-sectional survey conducted in 22 acute care hospitals and 21 long-term care hospitals in Osaka, Japan reported that the carriage rate was 12.2% [32]. The first NDM-producing K. pneumoniae was discovered in 2009 in a patient who returned to Sweden from an Indian hospital [33]. NDM-producing organisms were found widely in Indian subcontinent and many European countries [12].
In Korea, the prevalence of CRE infection increased in 2015–2016 according to the national sentinel surveillance [34]. In June 2017, CRE infection was classified as a nationally notifiable communicable disease [35]. The reports of CRE infection cases have been increasing ever since. There were 11,954 cases in 2018, 15,369 in 2019, and 17,766 in 2020 [36]. In 2018–2019, the most commonly reported species was K. pneumoniae (51.9%–60.4%), the proportion of CPE in CRE was 49.8%–57.8%, and KPC was the most common carbapenemase. In a 2018, 1-year surveillance of CRE among 1,468 clinical isolates from hospitals in Seoul, K. pneumoniae and E. coli were the major isolates, 58.1% were CPE, and KPC-2 was the most commonly found carbapenemase [37]. In 2010, the first nosocomial clustering of NDM-1 producing K. pneumoniae occurred in a single tertiary hospital in Korea [38], and isolates with NDM subtypes were reported [37,39]. The percentage of patients infected with carbapenem-resistant organisms at a newly opened ICU was 2.3%–7.8% between 2005 and 2008 [40]. A study conducted in an emergency ICU setting in a tertiary hospital showed a 2.8% carriage rate at admission and a 2.6% (21/810) acquisition rate during the ICU stay (4.3 per 1,000 person-days) [41]. Among them, 42.9% were identified as CPE, and KPC was the most common carbapenemase. A recent study conducted in a medical ICU of an 816 bed hospital in Korea showed that 6.3% patients were positive for CRE from surveillance or clinical cultures, and the prevalence of CPE was 3.8% among ICU patients [42].
The presence of underlying comorbid conditions, prior antimicrobial exposure, indwelling catheters or devices, and prior use of healthcare facilities are major risk factors for CRE colonization (Table 2) [41-45]. These exposures are common during ICU stay; therefore, ICU stays themselves are a significant risk factor for CRE colonization [45]. The length of hospital or ICU stay has been found to be associated with CRE acquisition and colonization [41,45].
The use of carbapenem is a risk factor for CRE acquisition, but the proportion reported for carbapenem use among CRE-colonized patients varies from 15% to 75% [41,42,46,47]. The use of cephalosporins, β-lactams or β-lactams/β-lactamase inhibitors, aminoglycosides, fluoroquinolones, or glycopeptides is a risk factor for CRE colonization [41,42,45,48]. The cocolonization of MDROs including MRAB or ESBL-producing bacteria is more frequent in CRE colonized patients [41,43]. Since exposure to MDROs is frequent in healthcare settings, hospitalization within the previous 6–12 months and regular visits to outpatient clinics are major risk factors for CRE colonization [41,43,48,49]. A study predicting CRE carriage at the time of admission using the Illinois hospital discharge database showed that age, number of hospitalizations at shortterm acute care hospitals in the previous 365 days, length of hospital stay, and current admission to long-term acute care hospital (LTACH) are independent risk factors for CRE colonization [49].
Exposure to a long-term care facility (LTCF) is another significant risk factor for colonization of CRE. In four hospitals in a metropolitan area in the US, CRE colonization was detected in 8.3% of patients admitted from an LTCF, but it was 0% among community patients. Similarly, patients admitted from high-acuity LTCFs, e.g., skilled nursing facilities with ventilator care and LTACH, had 7.0-fold greater odds of colonization with KPC-producing CRE than patients from skilled nursing facilities without ventilator care [50]. The point prevalence of CRE colonization among patients admitted to LTCF in the District of Columbia in the US was 7.0% [51]. In Korea, the prevalence of CRE colonization in LTCFs is limited, but a report from national notifiable diseases showed that the proportion of CRE infection reported from nursing hospitals increased from 4.0% in 2018 to 10% in 2020 [52]. A recent study in a Korean hospital showed that previous admission in an LTCF was associated with CPE infection or colonization in the ICU [42]. Patients admitted from LTCF have a high risk of CRE carriage and can be a source of reintroduction of CRE to acute care hospitals because of the low number of well-trained infection control staff and limited facilities to control CRE [53].
Decreasing CRE acquisition in healthcare facilities is essential for infection control and may be an indicator of infection control in the hospital. Risk factors for acquisition include regular visits to the outpatient clinic, male sex, history of hospitalization within one year, and co-colonization with MRAB- or ESBL-producing bacteria [41,43].
Because treatment options are limited to few antibiotics and are often associated with delays or ineffective treatment, invasive infections caused by CRE are associated with a high mortality rate [48,54,55]. In a study including patients with CRE bacteremia, 22% of patients died within 14 days, and after adjusting for the severity of illness, underlying medical condition, and types of antibiotic treatment, the odds of dying within 14 days were 4.92 times greater for patients with CPE compared with those with non-carbapenemase producing CRE [56]. In another study conducted in a CRE epicenter in the US, 38% of patients with CRE bacteremia had septic shock, and 49% died within 30 days [57]. In a meta-analysis of mortality among hospitalized patients with a severe infection including pneumonia, complicated urinary tract infection, bacteremia, and complicated intra-abdominal infections attributable to CRE pathogens, CRE was associated with a significantly higher overall mortality (odds ratio, 3.39; 95% confidence interval, 2.35–4.89) [58].
Although CRE colonization can be detected on admission, clinical infection may not occur in some patients [41]. In a study on CRE acquisition rates in emergency ICUs, CRE acquisition was not an independent risk factor for in-hospital mortality [41]. However, in an observational cohort study conducted in New York City, 11% (36/338) patients were CRE-colonized at the time of admission. Of them, 47% developed a CRE infection within 30 days with a 10.8-fold increased odds of developing CRE infection at 30 days (95% confidence interval, 2.8–41.9) [48]. CRE colonization in critically ill patients is associated with at least a 2-fold increased risk of infection by the colonizing strain [54].
In general, hand hygiene and contact precautions are the mainstay of infection control measures for CRE, like other MDROs. Patients with CRE colonization or CRE infection should be housed in single patient rooms or isolated as a cohort [59,60]. Hand hygiene is the most critical measure to prevent CRE transmission, and it should be monitored carefully. Healthcare facilities should provide adequate hand hygiene stations with proper supplies [61]. Hand hygiene should be performed before donning a gown and gloves for contact precaution. A single-use gown and gloves should be donned before entering the patient’s room (isolation room), and the used gown and gloved should be taken off before exiting the patient’s room. And hand hygiene should be performed upon leaving the room [59]. In the current guideline, contact precaution should be continued until the three sequential CRE cultures are revealed negative [60]. In some patients, CRE can be colonized for over 12 months after initial acquisition, but there is no consensus regarding when to discontinue contact precaution in such patients (Figure 1) [62].
Devices, including central venous catheters, endotracheal tubes, urinary catheters, and nasogastric tubes, are risk factors for CRE colonization [42,63]. To reduce CRE colonization and device-associated infection, minimizing device use is essential; therefore, device use should be reviewed daily, and devices should be removed when no longer needed [59].
The environment can also be a reservoir of CRE. Equipment, such as stethoscopes, thermometers, blood pressure cuffs, etc., should be used individually for each patient. If the equipment is not disposable, it should be disinfected or sterilized before reuse. Terminal cleaning of rooms wherein CRE-infected patients have been admitted is vital because the environment can serve as a source for transmission [60]. A systematic review revealed that sinks, drains, and faucets were the most frequently colonized spaces [64]. Pseudomonas aeruginosa and A. baumannii were found in sink basins and Enterobacteriaceae in the drains. Some studies have reported CRE transmission between the environment and patients [64]. Reinforcing general infection control measures and chemical disinfection has been found to be effective in some outbreaks. Chemical disinfection using hydrogen peroxide, bleach, or acetic acid is also helpful [64,65].
Many hospitals in Korea have already become CRE-endemic as they have failed to control methicillin-resistant S. aureus (MRSA), VRE, carbapenem-resistant A. baumannii, and carbapenem-resistant P. aeruginosa (CRPA) [66]. The Korean National Healthcare-associated Infections Surveillance System (KONIS) was established in 2006, and it is being operated by the Korean Society for Healthcare-Associated Infection Control and Prevention as a national research project [67]. According to the KONIS 2016–2017 report, the proportion of resistant pathogens was as follows: MRSA 79.7%, VRE 6.5%, CRPA 45.2%, and carbapenem-resistant K. pneumoniae 12.1% [68].
Enhanced infection control using screening for early detection of the intestinal carrier of CRE is effective [12]. An ICU at a hospital in New York City, where carbapenem-resistant K. pneumonia was endemic, implemented screening for colonization. After obtaining a rectal swab sample for CRE culture, patients were isolated in rooms at the far end of an ICU. This study showed that the mean number of new patients per 1,000 patient-days per quarter year with cultures yielding carbapenem-resistant K. pneumoniae decreased from 9.7 before intervention to 3.7 after intervention [69]. In a study conducted in Hong Kong, active CRE surveillance at ICUs was found to be a cost-effective way to reduce CRE infection and mortality rate [70].
Active surveillance for CRE has been recommended in governments’ guidelines from many countries [59,60]. Some hospitals implement this intervention in the CRE outbreak setting, but it is helpful as a standard measure in the endemic settings [53] or in ICUs in Korea where CRE detection rates are increasing. Active surveillance with preemptive contact isolation is recommended to control CRE by reducing transmission and colonization pressure [71,72]. Patients with the risk factors for colonization or acquisition of CRE, including prior antimicrobial exposures, previous history of hospitalizations, regular visits to outpatient clinics, the presence of underlying comorbid conditions, indwelling catheters or devices, and exposure to LTCF, are recommended for active surveillance [41- 45,50]. Since most patients admitted to ICUs have the aforementioned risk factors and CRE colonization or acquisition is frequent in the ICUs, it is recommended to screen CRE carriers at the time of admission to ICUs.
Laboratory methods to screen for CRE have been developed, leading to improved detection of CRE carriage [71]. Two major screening methods to detect CRE from rectal or perianal swabs or stools are culture-based methods and molecular-based methods. Culture-based methods include the CDC screening method using selective broth enrichment of CRE [73], direct inoculation on MacConkey agar with the placement of carbapenem disks [74], and culture using chromogenic medium [12,71]. The CDC screening method using broth enrichment culture of a rectal swab with a carbapenem disk has a relatively longer turnaround time, but it is possible to carry out using reagents and skills commonly maintained in most clinical laboratories and is able to detect CRE with various carbapenemases [71]. According to isolates according to the carbapenemases, the overall sensitivities and specificities of CDC method are 57.6%–98.8% and 49.6%–100%, respectively [12,71]. Surveillance culture using chromogenic medium involves direct plating of rectal swabs in selective chromogenic media. It can shorten the turnaround time, but some media can target only limited types of carbapenemase producers and have markedly decreased sensitivity for other types of carbapenemases [11]. Moreover, the sensitivities and specifies vary according to the manufacturers and targeted carbapenemases [12,71], therefore, certain chromogenic media should be selected according to the local epidemiologic features.
Molecular assays detect the presence of specific carbapenemase genes. The commercial molecular assays include multiplex polymerase chain reaction, microarray and isothermal amplification [71]. These methods are advantageous because they are labor-saving and have faster turnaround times and higher sensitivity than culture-based methods. However, some of these assays cannot detect specific carbapenemase genes due to the assay design [71]. Preemptive contact isolation of patients newly admitted to ICUs that is continued until the surveillance test results are negative usually requires the use of faster molecular assays directly applied to rectal swabs or stools.
Some hospitals implement both methods (culture-based screening and molecular assays) simultaneously to detect CRE. Patients admitted to the ICU or those with high risks are handled with contact precaution preemptively, and rectal swabs for molecular assays and CRE cultures are obtained. Patients who test positive by the fast molecular assays are subjected to continued contact isolation, while those who test negative can be released from the preemptive contact isolation until the results of culture-based assays are available. Experts suggest that routine surveillance assays should be done weekly or regularly in high-risk units (Figure 1) [12,41,59,60]. The screening methods for surveillance should be decided after considering the purpose, the epidemic or endemic situation of each institution or regional location, and the laboratory capability of each hospital [12].
CRE colonization and infection are a significant problem in ICUs, and the prevalence of CRE is increasing. The CRE situation is serious in Korea as well as globally; CRE could become an endemic if not controlled. Strict infection control, including hand hygiene, contact precaution, device control, environmental cleaning, and early detection of CRE carriers with active surveillance methods, should be implemented in ICUs, and the attention of critical care staff is essential for controlling CRE.
▪ Due to limited treatment options, invasive carbapenemresistant Enterobacterales (CRE) colonization and infection in critically ill patients are associated with high mortality.
▪ The prevalence of CRE and carbapenemase-producing CRE continues to increase.
▪ Infection control measures, including hand hygiene, contact precaution, environmental control, and active surveillance should be implemented to reduce mortality due to CRE infection.
ACKNOWLEDGMENTS
This work was supported by clinical research grant from Pusan National University Hospital in 2020.
REFERENCES
1. Fridkin SK, Welbel SF, Weinstein RA. Magnitude and prevention of nosocomial infections in the intensive care unit. Infect Dis Clin North Am. 1997; 11:479–96.


2. Vincent JL, Rello J, Marshall J, Silva E, Anzueto A, Martin CD, et al. International study of the prevalence and outcomes of infection in intensive care units. JAMA. 2009; 302:2323–9.


3. Hidron AI, Edwards JR, Patel J, Horan TC, Sievert DM, Pollock DA, et al. NHSN annual update: antimicrobial-resistant pathogens associated with healthcare-associated infections: annual summary of data reported to the National Healthcare Safety Network at the Centers for Disease Control and Prevention, 2006-2007. Infect Control Hosp Epidemiol. 2008; 29:996–1011.
4. Centers for Disease Control and Prevention (CDC). Guidance for control of infections with carbapenem-resistant or carbapenemase-producing Enterobacteriaceae in acute care facilities. MMWR Morb Mortal Wkly Rep. 2009; 58:256–60.
5. Doi Y. Treatment options for carbapenem-resistant gram-negative bacterial infections. Clin Infect Dis. 2019; 69(Suppl 7):S565–75.


6. Centers for Disease Control and Prevention. CRE technical Information [Internet]. Atlanta (GA): U.S. Department of Health & Human Services;2019 [cited 2021 Mar 25]. Available from: https://www.cdc.gov/hai/organisms/cre/technical-info.html.
7. Adeolu M, Alnajar S, Naushad S, Gupta RS. Genome-based phylogeny and taxonomy of the ‘Enterobacteriales’: proposal for Enterobacterales ord. nov. divided into the families Enterobacteriaceae, Erwiniaceae fam. nov., Pectobacteriaceae fam. nov., Yersiniaceae fam. nov., Hafniaceae fam. nov., Morganellaceae fam. nov., and Budviciaceae fam. nov. Int J Syst Evol Microbiol. 2016; 66:5575–99.


8. Logan LK, Weinstein RA. The epidemiology of carbapenemresistant Enterobacteriaceae: the impact and evolution of a global menace. J Infect Dis. 2017; 215(suppl_1):S28–36.


9. Woodworth KR, Walters MS, Weiner LM, Edwards J, Brown AC, Huang JY, et al. Vital signs: containment of novel multidrug-resistant organisms and resistance mechanisms. United States, 2006-2017. MMWR Morb Mortal Wkly Rep. 2018; 67:396–401.
10. van Duin D, Doi Y. The global epidemiology of carbapenemase-producing Enterobacteriaceae. Virulence. 2017; 8:460–9.


11. Queenan AM, Bush K. Carbapenemases: the versatile betalactamases. Clin Microbiol Rev. 2007; 20:440–58.
12. Viau R, Frank KM, Jacobs MR, Wilson B, Kaye K, Donskey CJ, et al. Intestinal carriage of carbapenemase-producing organisms: current status of surveillance methods. Clin Microbiol Rev. 2016; 29:1–27.


14. Navon-Venezia S, Chmelnitsky I, Leavitt A, Schwaber MJ, Schwartz D, Carmeli Y. Plasmid-mediated imipenem-hydrolyzing enzyme KPC-2 among multiple carbapenem-resistant Escherichia coli clones in Israel. Antimicrob Agents Chemother. 2006; 50:3098–101.
15. Bratu S, Brooks S, Burney S, Kochar S, Gupta J, Landman D, et al. Detection and spread of Escherichia coli possessing the plasmid-borne carbapenemase KPC-2 in Brooklyn, New York. Clin Infect Dis. 2007; 44:972–5.


16. Hossain A, Ferraro MJ, Pino RM, Dew RB 3rd, Moland ES, Lockhart TJ, et al. Plasmid-mediated carbapenem-hydrolyzing enzyme KPC-2 in an Enterobacter sp. Antimicrob Agents Chemother. 2004; 48:4438–40.
17. Marchaim D, Navon-Venezia S, Schwaber MJ, Carmeli Y. Isolation of imipenem-resistant Enterobacter species: emergence of KPC-2 carbapenemase, molecular characterization, epidemiology, and outcomes. Antimicrob Agents Chemother. 2008; 52:1413–8.
19. Yoon EJ, Choi YJ, Park SH, Shin JH, Park SG, Choi JR, et al. A novel KPC variant KPC-55 in Klebsiella pneumoniae ST307 of reinforced meropenem-hydrolyzing activity. Front Microbiol. 2020; 11:561317.


20. Naas T, Oueslati S, Bonnin RA, Dabos ML, Zavala A, Dortet L, et al. Beta-lactamase database (BLDB): structure and function. J Enzyme Inhib Med Chem. 2017; 32:917–9.
21. Beta-Lactamase DataBase (BLDB). Beta-lactamase database: structure and function [Internet]. Le Kremlin-Bicêtre: BLDB;2021 [cited 2021 May 27]. Available from: http://bldb.eu/BLDB.php?prot=A#KPC.
22. Guh AY, Bulens SN, Mu Y, Jacob JT, Reno J, Scott J, et al. Epidemiology of carbapenem-resistant Enterobacteriaceae in 7 US communities, 2012-2013. JAMA. 2015; 314:1479–87.


23. Centers for Disease Control and Prevention. Antibiotic resistance threats in the United States. [Internet]. Atlanta (GA): U.S. Department of Health & Human Services;2019 [cited 2021 May 27]. Available from: https://www.cdc.gov/drugresistance/pdf/threats-report/2019-ar-threats-report-508.pdf.
24. Yigit H, Queenan AM, Anderson GJ, Domenech-Sanchez A, Biddle JW, Steward CD, et al. Novel carbapenem-hydrolyzing beta-lactamase, KPC-1, from a carbapenem-resistant strain of Klebsiella pneumoniae. Antimicrob Agents Chemother. 2001; 45:1151–61.
25. Munoz-Price LS, Poirel L, Bonomo RA, Schwaber MJ, Daikos GL, Cormican M, et al. Clinical epidemiology of the global expansion of Klebsiella pneumoniae carbapenemases. Lancet Infect Dis. 2013; 13:785–96.


26. Zhang Y, Wang Q, Yin Y, Chen H, Jin L, Gu B, et al. Epidemiology of carbapenem-resistant enterobacteriaceae infections: report from the China CRE Network. Antimicrob Agents Chemother. 2018; 62:e01882–17.


27. Huang XL, Wu SH, Shi PF, Xu LH, Chen C, Xie YP, et al. Active screening of intestinal carbapenem-resistant Enterobacteriaceae in high-risk patients admitted to the hematology wards and its effect evaluation. Zhonghua Xue Ye Xue Za Zhi. 2020; 41:932–6.
28. Yan L, Sun J, Xu X, Huang S. Epidemiology and risk factors of rectal colonization of carbapenemase-producing Enterobacteriaceae among high-risk patients from ICU and HSCT wards in a university hospital. Antimicrob Resist Infect Control. 2020; 9:155.


29. Zhang R, Liu L, Zhou H, Chan EW, Li J, Fang Y, et al. Nationwide surveillance of clinical carbapenem-resistant Enterobacteriaceae (CRE) strains in China. EBioMedicine. 2017; 19:98–106.


30. Watanabe M, Iyobe S, Inoue M, Mitsuhashi S. Transferable imipenem resistance in Pseudomonas aeruginosa. Antimicrob Agents Chemother. 1991; 35:147–51.


31. Ohno Y, Nakamura A, Hashimoto E, Matsutani H, Abe N, Fukuda S, et al. Molecular epidemiology of carbapenemase-producing Enterobacteriaceae in a primary care hospital in Japan, 2010-2013. J Infect Chemother. 2017; 23:224–9.


32. Yamamoto N, Asada R, Kawahara R, Hagiya H, Akeda Y, Shanmugakani RK, et al. Prevalence of, and risk factors for, carriage of carbapenem-resistant Enterobacteriaceae among hospitalized patients in Japan. J Hosp Infect. 2017; 97:212–7.


33. Yong D, Toleman MA, Giske CG, Cho HS, Sundman K, Lee K, et al. Characterization of a new metallo-beta-lactamase gene, bla(NDM-1), and a novel erythromycin esterase gene carried on a unique genetic structure in Klebsiella pneumoniae sequence type 14 from India. Antimicrob Agents Chemother. 2009; 53:5046–54.
34. Park JW, Lee E, Lee SJ, Lee H. Status of carbapenemase-producing Enterobacteriaceae incidences in Korea, 2015-2016. Public Health Wkly Rep. 2017; 10:1243–7.
35. Korea Disease Control and Prevention Agency. Infectious disease portal: Carbapenem-resistant Enterobacteriaceae infection [Internet]. Sejong: Ministry of Health and Welfare;2021 [cited 2021 March 25]. Available from: http://www.kdca.go.kr/npt/biz/npp/portal/nppSumryMain.do.
36. Korea Disease Control and Prevention Agency. Infectious disease portal [Internet]. Sejong: Ministry of Health and Welfare;2021 [cited 2021 March 25]. Available from: http://www.kdca.go.kr/npt/biz/npp/ist/simple/simplePdStatsMain.do.
37. Park SH, Kim JS, Kim HS, Yu JK, Han SH, Kang MJ, et al. Prevalence of carbapenem-resistant Enterobacteriaceae in Seoul, Korea. J Bacteriol Virol. 2020; 50:107–16.


38. Kim MN, Yong D, An D, Chung HS, Woo JH, Lee K, et al. Nosocomial clustering of NDM-1-producing Klebsiella pneumoniae sequence type 340 strains in four patients at a South Korean tertiary care hospital. J Clin Microbiol. 2012; 50:1433–6.


39. Yoon EJ, Kang DY, Yang JW, Kim D, Lee H, Lee KJ, et al. New Delhi metallo-beta-lactamase-producing Enterobacteriaceae in South Korea between 2010 and 2015. Front Microbiol. 2018; 9:571.


40. Kim BM, Jeon EJ, Jang JY, Chung JW, Park J, Choi JC, et al. Four year trend of carbapenem-resistance in newly opened ICUs of a university-affiliated hospital of South Korea. Tuberc Respir Dis (Seoul). 2012; 72:360–6.


41. Kang JS, Yi J, Ko MK, Lee SO, Lee JE, Kim KH. Prevalence and risk factors of carbapenem-resistant Enterobacteriaceae acquisition in an emergency intensive care unit in a tertiary hospital in Korea: a case-control study. J Korean Med Sci. 2019; 34:e140.


42. Kim YA, Lee SJ, Park YS, Lee YJ, Yeon JH, Seo YH, et al. Risk factors for carbapenemase-producing Enterobacterales infection or colonization in a Korean intensive care unit: a casecontrol study. Antibiotics (Basel). 2020; 9:680.


43. Lee HJ, Choi JK, Cho SY, Kim SH, Park SH, Choi SM, et al. Carbapenem-resistant Enterobacteriaceae: prevalence and risk factors in a single community-based hospital in Korea. Infect Chemother. 2016; 48:166–73.
44. Papadimitriou-Olivgeris M, Marangos M, Fligou F, Christofidou M, Sklavou C, Vamvakopoulou S, et al. KPC-producing Klebsiella pneumoniae enteric colonization acquired during intensive care unit stay: the significance of risk factors for its development and its impact on mortality. Diagn Microbiol Infect Dis. 2013; 77:169–73.
45. Swaminathan M, Sharma S, Poliansky Blash S, Patel G, Banach DB, Phillips M, et al. Prevalence and risk factors for acquisition of carbapenem-resistant Enterobacteriaceae in the setting of endemicity. Infect Control Hosp Epidemiol. 2013; 34:809–17.


46. Leavitt A, Navon-Venezia S, Chmelnitsky I, Schwaber MJ, Carmeli Y. Emergence of KPC-2 and KPC-3 in carbapenem-resistant Klebsiella pneumoniae strains in an Israeli hospital. Antimicrob Agents Chemother. 2007; 51:3026–9.
47. Wei ZQ, Du XX, Yu YS, Shen P, Chen YG, Li LJ. Plasmid-mediated KPC-2 in a Klebsiella pneumoniae isolate from China. Antimicrob Agents Chemother. 2007; 51:763–5.
48. McConville TH, Sullivan SB, Gomez-Simmonds A, Whittier S, Uhlemann AC. Carbapenem-resistant Enterobacteriaceae colonization (CRE) and subsequent risk of infection and 90- day mortality in critically ill patients, an observational study. PLoS One. 2017; 12:e0186195.
49. Lin MY, Ray MJ, Rezny S, Runningdeer E, Weinstein RA, Trick WE. Predicting carbapenem-resistant Enterobacteriaceae carriage at the time of admission using a statewide hospital discharge database. Open Forum Infect Dis. 2019; 6:ofz483.


50. Prabaker K, Lin MY, McNally M, Cherabuddi K, Ahmed S, Norris A, et al. Transfer from high-acuity long-term care facilities is associated with carriage of Klebsiella pneumoniae carbapenemase-producing Enterobacteriaceae: a multihospital study. Infect Control Hosp Epidemiol. 2012; 33:1193–9.


51. Reuben J, Donegan N, Wortmann G, DeBiasi R, Song X, Kumar P, et al. Healthcare antibiotic resistance prevalence: DC (HARP-DC). A regional prevalence assessment of carbapenem-resistant Enterobacteriaceae (CRE) in Healthcare Facilities in Washington, District of Columbia. Infect Control Hosp Epidemiol. 2017; 38:921–9.
52. Korea Disease Control and Prevention Agency. CRE infection continue to increase, strengthen infection control. Cheongju: Korea Disease Control and Prevention Agency;2020.
53. Schwaber MJ, Carmeli Y. An ongoing national intervention to contain the spread of carbapenem-resistant Enterobacteriaceae. Clin Infect Dis. 2014; 58:697–703.


54. Dickstein Y, Edelman R, Dror T, Hussein K, Bar-Lavie Y, Paul M. Carbapenem-resistant Enterobacteriaceae colonization and infection in critically ill patients: a retrospective matched cohort comparison with non-carriers. J Hosp Infect. 2016; 94:54–9.


55. Martín-Loeches I, Diaz E, Vallés J. Risks for multidrug-resistant pathogens in the ICU. Curr Opin Crit Care. 2014; 20:516–24.


56. Tamma PD, Goodman KE, Harris AD, Tekle T, Roberts A, Taiwo A, et al. Comparing the outcomes of patients with carbapenemase-producing and non-carbapenemase-producing carbapenem-resistant Enterobacteriaceae bacteremia. Clin Infect Dis. 2017; 64:257–64.
57. Satlin MJ, Chen L, Patel G, Gomez-Simmonds A, Weston G, Kim AC, et al. Multicenter clinical and molecular epidemiological analysis of bacteremia due to carbapenem-resistant Enterobacteriaceae (CRE) in the CRE epicenter of the United States. Antimicrob Agents Chemother. 2017; 61:e02349–16.


58. Martin A, Fahrbach K, Zhao Q, Lodise T. Association between carbapenem resistance and mortality among adult, hospitalized patients with serious infections due to Enterobacteriaceae: results of a systematic literature review and meta-analysis. Open Forum Infect Dis. 2018; 5:ofy150.


59. National Center for Emerging and Zoonotic Infectious Diseases. Facility guidance for control of carbapenem-resistant Enterobacteriaceae (CRE): November 2015 update CRE toolkit [Internet]. Atlanta (GA): U.S. Department of Health & Human Services;2015 [cited 2021 March 26]. Available from: https://www.cdc.gov/hai/pdfs/cre/CRE-guidance-508.pdf.
60. Korea Disease Control and Prevention Agency. Guideline for control of healthcare-associated infection, 2021. Cheongju: Korea Disease Control and Prevention Agency;2021.
61. Boyce JM, Pittet D; Healthcare Infection Control Practices Advisory Committee; HICPAC/SHEA/APIC/IDSA Hand Hygiene Task Force. Guideline for hand hygiene in health-care settings. Recommendations of the Healthcare Infection Control Practices Advisory Committee and the HICPAC/SHEA/APIC/IDSA Hand Hygiene Task Force. Society for Healthcare Epidemiology of America/Association for Professionals in Infection Control/Infectious Diseases Society of America. MMWR Recomm Rep. 2002; 51:1–45.
62. Kim YK, Song SA, Lee JN, Oh M, Jo KM, Kim HJ, et al. Clinical factors predicting persistent carriage of Klebsiella pneumoniae carbapenemase-producing carbapenem-resistant Enterobacteriaceae among patients with known carriage. J Hosp Infect. 2018; 99:405–12.


63. Papadimitriou-Olivgeris M, Marangos M, Fligou F, Christofidou M, Bartzavali C, Anastassiou ED, et al. Risk factors for KPCproducing Klebsiella pneumoniae enteric colonization upon ICU admission. J Antimicrob Chemother. 2012; 67:2976–81.
64. Kizny Gordon AE, Mathers AJ, Cheong EY, Gottlieb T, Kotay S, Walker AS, et al. The hospital water environment as a reservoir for carbapenem-resistant organisms causing hospitalacquired infections: a systematic review of the literature. Clin Infect Dis. 2017; 64:1435–44.
65. Smolders D, Hendriks B, Rogiers P, Mul M, Gordts B. Acetic acid as a decontamination method for ICU sink drains colonized by carbapenemase-producing Enterobacteriaceae and its effect on CPE infections. J Hosp Infect. 2019; 102:82–8.


66. Kim MN. Multidrug-resistant organisms and healthcare-associated infections. Hanyang Med Rev. 2011; 31:141–52.


67. Choi YH. Prospective nationwide healthcare-associated infection surveillance system in South Korea. J Korean Med Assoc. 2018; 61:21–5.


68. Kwak YG, Choi YH, Choi JY, Yoo HM, Lee SO, Kim HB, et al. Korean national healthcare-associated infections surveillance system, intensive care unit module report: summary of data from July 2016 through June 2017. Korean J healthc assoc Infect Control Prev. 2018; 23:25–38.


69. Kochar S, Sheard T, Sharma R, Hui A, Tolentino E, Allen G, et al. Success of an infection control program to reduce the spread of carbapenem-resistant Klebsiella pneumoniae. Infect Control Hosp Epidemiol. 2009; 30:447–52.


70. Ho KW, Ng WT, Ip M, You JH. Active surveillance of carbapenem-resistant Enterobacteriaceae in intensive care units: is it cost-effective in a nonendemic region? Am J Infect Control. 2016; 44:394–9.


71. Richter SS, Marchaim D. Screening for carbapenem-resistant Enterobacteriaceae: who, when, and how? Virulence. 2017; 8:417–26.


72. Arena F, Vannetti F, Di Pilato V, Fabbri L, Colavecchio OL, Giani T, et al. Diversity of the epidemiology of carbapenemase-producing Enterobacteriaceae in long-term acute care rehabilitation settings from an area of hyperendemicity, and evaluation of an intervention bundle. J Hosp Infect. 2018; 100:29–34.


73. Landman D, Salvani JK, Bratu S, Quale J. Evaluation of techniques for detection of carbapenem-resistant Klebsiella pneumoniae in stool surveillance cultures. J Clin Microbiol. 2005; 43:5639–41.
Figure 1.
Suggested preemptive contact precaution and routine surveillance assays to detect carbapenem-resistant Enterobacterales (CRE) in high risk settings.
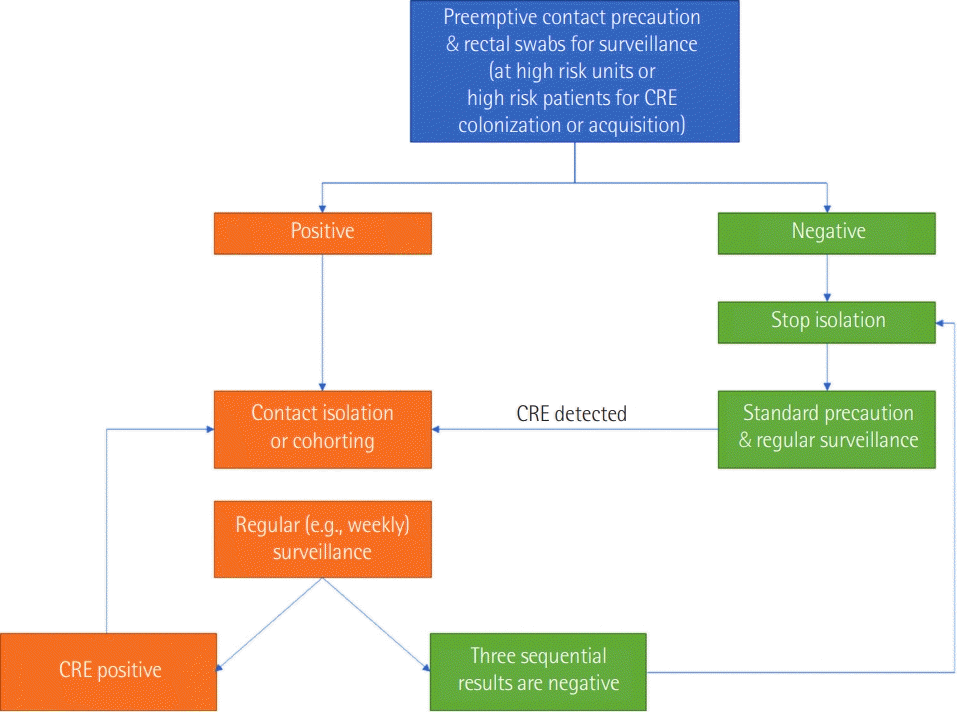
Table 1.
Table 2.
Risk factors for colonization of CRE