Abstract
Background:
The aim of this study was to determine the prognostic value and optimal sampling time of serum S-100B protein for the prediction of poor neurological outcomes in post-cardiac arrest (CA) patients treated with therapeutic hypothermia (TH).
Methods:
We prospectively measured serum S100 calcium binding protein beta subunit (S-100B protein) levels 12 times (0–96 hours) after the return of spontaneous circulation (ROSC). The patients were classified into two groups based on cerebral performance category (CPC): the good neurological outcome group (CPC 1–2 at 6 months) and the poor neurological outcome group (CPC 3–5). We compared serial changes and serum S-100B protein levels at each time point between the two groups and performed receiver operating characteristic curve analysis for the prediction of poor neurological outcomes.
Results:
A total of 40 patients were enrolled in the study. S-100B protein levels peaked at ROSC (0 hour), decreased rapidly to 6 hours and maintained a similar level thereafter. Serum S-100B protein levels in the poor CPC group (n = 22) were significantly higher than in the good CPC group (n = 18) at all time points after ROSC except at 4 hours. The time points with highest area under curve were 24 (0.829) and 36 (0.837) hours. The cut-off value, the sensitivity (24/36 hours) and specificity (24/36 hours) for the prediction of poor CPC at 24 and 48 hours were 0.221/0.249 ug/L, 75/65% and 82.4/94.1%, respectively.
Recent advances in the emergency medical system (EMS), cardiopulmonary resuscitation (CPR) and post-cardiac arrest care have improved return of spontaneous circulation (ROSC) survival to hospital discharge and neurological recovery in patients with out-of-hospital cardiac arrest (OHCA). However, the overall outcomes of patients with OHCA still remain poor.[1–5]
The unfavorable outcomes reflect the unique and complex pathophysiological processes known as post-cardiac arrest syndrome in which OHCA patients with ROSC can develop brain injury, myocardial dysfunction, systemic ischemia or reperfusion injury in addition to previously developed lesions.[6] In particular, hypoxic-ischemic brain injury caused by an interruption of cerebral blood flow and reperfusion injury following ROSC are the leading causes of early mortality and morbidity in patients even after successful ROSC. In some cases, brain dysfunction occurs, causing a significant difference in the quality of life of patients and their families in addition to the increase in medical care spending.[7]
To minimize damage to the brain, an early measure predicting the severity of brain damage and outcomes is needed to provide intensive care including cerebral resuscitation in an appropriate and timely manner. Consequently, many related areas have been actively researched.
Mild therapeutic hypothermia (MTH) is reported as the only effective and proven approach to improve neurological outcomes and widely used as the standard treatment for patients following CA worldwide.[8,9]
However, predicting neurological outcomes after CA remains challenging. Up-to-date methods used to predict the severity of brain injury in CA survivors include several clinical and neuro-physiological parameters and radiological tests. However, the accuracy and sensitivity of these methods are low and tend to predict unfavorable neurological outcomes in most cases.[10] Additionally, most patients with ROSC after CPR are given various drugs including sedatives, anticonvulsants and muscle relaxants to prevent brain injury while they show unstable hemodynamics. These drugs are administered at higher doses to cause MTH, making an accurate neurological diagnosis and performing a neuroradiological test more difficult.[6] Any tests that are easy to perform repeatedly at the bedside without cost constraints and are high performing in terms of accuracy, sensitivity and specificity are very useful for early prediction of neurological outcomes.
Biochemical markers have great potential as a clinical tool for monitoring brain injury and predicting early outcomes. There are several biochemical tests used to evaluate the extent of brain damage. However, clinical studies on MTH are scarce and clinical data is insufficient. Among biochemical markers of brain injury, S-100 calcium binding protein B (S-100B) is highly specific to the central nervous system and serves as a glial cell marker while neuron-specific enolase (NSE) is a highly specific serum marker for neuronal degeneration. Since serum S-100B protein reflects the extent of hypoxic-ischemic brain damage, the level of S-100B protein can be useful in determining patient outcomes after MTH according to recent studies.[11–18] However, the overall frequency of blood sampling is low in most studies and the timing of blood sampling is different among studies, causing difficulty in determining real-time changes in brain injury. In addition, the cut-off values for predicting poor outcomes vary among studies, leading to variation in sensitivity and specificity.
In the present study, blood samples were obtained frequently at specific times to assess the changes in serum S-100B levels at each time to determine the effectiveness of the protein as a biochemical marker and the optimal measurement times for predicting neurological outcome based on the protein levels over time.
This study was conducted in the emergency room (ER) center at a regional hospital with 1,300 general ward beds and 100 intensive care unit beds. The hospital treats approximately 100,000 inpatients per year. Among the adult patients (age 18 years or above) admitted to the ER center due to non-traumatic OHCA from January to December 2010, those who underwent MTH after ROSC was achieved following CPR were evaluated for this study.
After emergency life saving measures such as CPR and cerebral resuscitation, standard care was provided in accordance with the 2008 American heart association and international liaison committee on resuscitation guidelines for CPR and emergency cardiovascular care. Patients were excluded if they died before the completion of MTH, had no biochemical tests, had a neurological disease including intracerebral hemorrhage, cerebral infarct, brain tumor, intracranial infection or dementia before CA and had any disease that could affect the biochemical composition of serum, including small cell lung cancer and renal failure before CA. When patients had ROSC following CPR, the informed consent was obtained after briefing patient families on the objectives of the study. The patients who did not agree to participate in the study were also excluded. This study was approved by the Ethics and Medical Research Committee of the hospital.
Patient variables were prospectively collected in the internally developed database on patients who underwent CPR, and included age, gender, patient health history, the location of CA, witness status, initiation of CPR by witnesses, CA-basic life support (BLS) and advanced cardiac life support (ACLS) time, BLS and ACLS-ROSC time, CA-ROSC time, cardiac rhythm on admission, causes of CA, the dose of epinephrine received during CPR, the number of electric shocks, the amount of vasopressor given over the 45-hr period after ROSC, acute physiology and chronic health evaluation II (APACHE II) and sequential organ failure assessment (SOFA) scores, arterial pH, serum lactate level, post anoxic seizure and target temperature and time taken during therapeutic hypothermia (TH).
Patients were treated with TH immediately after ROSC if they had glasgow coma scale > 8 and systolic blood pressure < 90 mmHg. TH was not administered to the patients who had traumatic CA or did not consent to TH. TH was also discontinued if the patients failed to maintain a systolic blood pressure < 90 mmHg for more than 12 hours despite sufficient intravenous fluid and vasopressor therapy.
The target temperature range was 32–34°C and either an external cooling or endovascular cooling method was used. Regarding the cooling device, the Arctic Sun®2000 & ArcticGelTM Pad (Medivance, Louisville, KY, USA) was used for external cooling and the Gard 3000® and CoolLine® Catheter (ZOLL, Chelsmford, MA, USA) was used for endovascular cooling. To reach the target temperature, 1 L of 4°C normal saline was used for infusion and bladder irrigation. All the patients underwent intubation and mechanical ventilation and sedatives and neuromuscular blocking agents were steadily administered to maintain appropriate sedation. Intracorporeal temperature was continuously monitored using a rectal thermometer. According to the preset TH protocol, the temperature was maintained in the target range for 24 hours and regulated in a range of 0.2–0.3°C every hour during the rewarming phase. After the initiation of TH, the temperature was maintained at 36.5°C for 72 hours.
A total of 12 blood samples were taken from each patient at 0, 2, 4, 6, 12, 18, 24, 36, 48, 60, 72 and 96 hours after ROSC. Collected blood samples were centrifugated at 3,000 rpm for 10 min and serum was kept in a container and frozen at −70°C before analysis.
Serum S-100B protein was measured with an electrochemiluminescence immunoassay (ECLIA) using the Elecsys 2020, including Elecsys S100® and NSE® kits (Roche Diagnostics, Mannheim, Germany). The range of detection was between 0.05 and 39 ug/L for each blood sample.
At 6 months after ROSC, the patients were classified based on cerebral performance category (CPC) score into the good outcome group (CPC score 1–2) and the poor outcome group (CPC score 3–5) and S-100B serum protein levels were compared at all time points.
A CPC score of 1 represents a slight cerebral disability but good cerebral performance resulting from mild neurological injury, score 2 represents a moderate cerebral disability, and independence in performing activities of daily living, score 3 represents severe cerebral disability, conscious, unstable and dependent, score 4 represents comatose and persistently vegetative state and score 5 represents brain death or death.
Patient variables and S-100B serum protein level at each time point were compared between the good outcome (CPC 1–2) and poor outcome groups (CPC 3–5). Since the total number of subjects between the 2 groups was less than 30, continuous variables were expressed as median and interquartile range (IQR) and nominal variables and class intervals were expressed as a number and frequency counts. A chi-square test or Fisher’s exact test was used to compare nominal variables and the Mann-Whitney U test was used to compare continuous variables.
Repeat measure analysis of variance (ANOVA) test was used to compare and analyze time-dependent changes in serum S100B protein levels between the good outcome and poor outcome groups. The cut-off values for optimal prediction of poor outcome were calculated based on the receiver operating characteristic curve (ROC curve) for time-dependent serum S100B level, the area under curve (AUC) value and Youden’s index. Sensitivity, specificity, positive predictive value (PPV) and negative predictive value (NPV) for each cut-off value were also calculated with 95% confidence intervals (CIs). To reduce false-positive results, the cut-off value associated with 100% specificity was determined at each time point. Statistical analysis was performed using a SPSS version 16.0 (SPSS Inc., Chicago, IL, USA) and MedCalc 12.7.7.0 (MedCalc Software, Mariakerke, Belgium). A p value of 0.05 was considered to indicate statistical significance.
A total of 399 OHCA patients were admitted to the hospital during the study period. Of those, 187 patients underwent CPR, 104 patients achieved ROSC (78 patients maintained spontaneous circulation for more than 20 min). Forty-four patients underwent MTH and 4 patients were excluded (1 non-consenting, 2 with renal failure and 1 with stroke). Finally, 40 patients were enrolled in the study.
The median age of subjects was 52 years (IQR 44–65.8 years). Of the 40 subjects, 31 were male (77.5%). CA occurred at home in 16 patients (40%), at work in 7 patients (17.5%), at a public location in 4 patients (10%) and in the ambulance in 4 patients (10%). Witnesses to the CA included a family member in 13 patients (32.5%) and bystanders in 9 patients (22.5%). There was no witness to the CA in 9 patients (22.5%) and CPR was initiated by bystanders in 15 patients (37.5%). The initial cardiac rhythm was ventricular fibrillation/tachycardia in 17 patients (42.5%), which was followed by asystole in 15 patients (37.5%), pulseless electrical activity in 8 patients (20%). The causes of CA were coronary heart disease in 21 patients (62.5%), non-coronary conditions in 13 patients (32.5%) and unidentified causes in 6 patients (15%). The median times from CA to BLS, ACLS and ROSC were 5.5 min (range, 2–10 min), 17 min (range, 10–23.5 min) and 30.5 min (range, 20–44.75 min), respectively. The median times taken from and the median time from BLS- and ACLS to ROSC were 27 min (range, 16–37 min) and 18.5 min (range, 6–29.25 min), respectively. The dose of epinephrine received during CPR was 3.5 mg (range, 1.25–8 mg) and the number of electric shocks was 1.5 (range, 0–6.75). The median APACHE II and SOFA scores were 22.5 (range, 18.25–27) and 9.5 (range, 7.25–11.75), respectively.
The target temperature for hypothermia was 34°C in 19 patients (47.5%), 33°C in 18 patients (45%) and 32°C in 3 patients (7.5%). Hypothermia was maintained by endovascular cooling in 22 patients (55%) and by external cooling in 18 patients (45%). Time from CA to start of TH was 193 min (range, 145– 288 min), time from start of TH to 34°C was 212 min (range, 150–300 min) and time from CA to 34°C and rewarming was 428 min (range, 320–581 min) and 660 min (range, 480–750 min), respectively. On admission, the median arterial pH was 7.065 (range, 6.973–7.20) and the median serum lactate level was 8.4 mmol/L (range, 7.0–12.0 mmol/L).
Based on neurological outcomes determined by the CPC at 6 months, good outcomes were achieved in 18 patients (45%, 11 with CPC 1 and 7 with CPC 2) whereas poor outcomes were observed in 22 patients (55%, 2 with CPC 3, 6 with CPC 4 and 14 with CPC 5).
The comparison of patient characteristics associated with neurological outcomes showed a significantly greater number of patients presented with shockable rhythms (ventricular fibrillation/ventricular tachycardia) (61.6% vs. 27.3%, p = 0.031) and coronary heart disease as a cause of CA (72.2% vs. 36.4%, p = 0.024) in the good outcome group compared with the poor outcome group. However, the frequency of post-anoxic seizure was significantly higher in the poor outcome group (68.2% vs. 35.3%, p = 0.024) than in the good outcome group. There were no significant differences between the two groups in terms of other patient characteristics. However, the poor outcome group required a longer time from CA to ACLS (16.0 min vs. 20 min, p = 0.070) and to rewarming (620 min vs. 720 min, p = 0.070) compared with the good outcome group.
Time-dependent serum S-100B protein levels of patients showed the highest level at 0 hour from ROSC. Then, serum S-100B protein in patients decreased within 6 hours and remained at a level similar to the 6-hr level (Fig.1).
The poor outcome group had significantly higher serum S-100B protein levels at all time points except for 4 hrs after ROSC (0.295 vs. 0.622 ug/L, p = 0.201) compared with the good outcome group (p = < 0.001–0.032; Table 2).
Based on time-dependent changes in serum S-100B protein, the decrease in the protein continued for 4–6 hours after ROSC in both groups. Then, the protein level remained almost unchanged in the good outcome group whereas it became elevated or decreased depending on the patient in the poor outcome group, although the overall change was not significant. The ANOVA analysis with repeated measures showed time-dependent changes in S-100B protein (F = 2.78, p < 0.019). However, there was no significant difference between the two groups (F = 1.23, p < 0.324; Fig. 2).
AUC values at each time point in the ROC curve analysis ranged from 0.619–0.837, showing the minimum value at 4 hours and the maximum value at 36 hours. AUC values > 0.7 were maintained at all time points except for 2 and 4 hours. AUC values > 0.8 were found at 36 hours (0.837), 24 hours (0.829), 72 hours (0.809) and 60 hours (0.807).
Cut-off values for S-100B ranged from 0.095 (48 hours) to 1.01 ug/L (0 hour) showing ranges of sensitivity from 65.0% (36 hours) to 90.5% (12 hours), specificity from 52.9% (12 hours) to 94.1% (36 hours), PPV from 66.7% (4 hours) to 92.9% (36 hours) and NPV from 62.5% (4 hours) to 86.7% (72 hours). For poor outcomes predicted by a specificity of 100%, the cut-off value ranged from 0.432 (48 hours) to 7.25 ug/L (0 hour), showing the ranges of sensitivity from 4.5% (2 and 4 hours) to 45% (48 hours) and NPV from 46.2% (2 and 4 hours) to 60.7% (48 hours).
The cut-off values corresponding with higher AUC values at 24 and 36 hours were 0.249 ug/L (0.685 ug/L) and 0.221 ug/L (0.612 ug/L), respectively, showing a sensitivity of 65 (35)% and 75 (40)%, PPV of 92.9% and 83.3% and NPV of 69.6 (56.7)% and 60.7 (58.6)%, respectively, with a specificity of 100% (Table 3) (Fig. 3 and 4).
Emergency medical service and CPR techniques have steadily improved and expanded, along with advances in post-CA care after ROSC. However, the long-term survival and neurological outcomes in patients after CA remain low in Korea.[3–5] Low initiation of bystander CPR, regional variations in EMS and different approaches to post-CA care among hospitals are likely responsible for lower long-term survival and unfavorable neurological outcomes compared with developed countries.
The majority of patients who survive after CPR may be left unconscious or have severe neurological symptoms due to brain injury, causing an emotional and financial burden on their families and society as a whole. Therefore, predicting neurological outcomes early after ROSC is vital for deciding on future treatment strategies including end-of-life decisions and amount and intensity of treatment.[19–21] Although many studies explored the possible predictors of neurological outcomes, neither accurate predictors nor adequate time to predict neurological outcomes has been established. In addition, the efficacy of TH, which is widely used clinically, has not been well-documented.
Because TH can alter the processes involved in brain injury and the use of sedatives and neuromuscular blocking agents may affect neurological assessment, results of TH-related studies cannot be applied to patients who do not undergo TH. Well-designed, prospective studies are therefore necessary to ensure the effects of TH.[10,22]
Among early predictors of neurological outcomes in patients after OHCA, cost-effective and easy-to-perform biochemical markers have been identified in previous studies. In particular, S-100B protein and NSE have been shown to play major roles.
S-100B is a dimeric calcium binding protein present in cells with a biological half-life of 2 hours. This protein is composed of 92 amino acids with a molecular weight of 21,000 - 10.7 kDa. As a heterodimer with an α-subunit and β-subunit, S100 (αβ form) protein is present in melanocytes and glial cells, whereas the homodimeric S100 (ββ form) protein is generally expressed by astrocytes and Schwann cells.[23] S-100B is produced by astrocytes, oligodendrocytes and lemmocytes and represents 0.2% of total brain proteins. Cerebrospinal fluid may leak following brain injury and flow into the bloodstream which can be measured when evaluating brain damage.[24]
In the present study, serum S-100B protein was repeatedly measured after ROSC and the poor outcome group maintained statistically significantly higher protein levels at all time points except 4 hours than the good outcome group. Several studies on TH also demonstrated a significant difference in the protein between the 2 groups. However, in previous studies measurements were performed less frequently over a shorter period of time compared with our study.
In the present study, AUC values of serum S-100B level used for predicting poor neurological outcomes were greater than 0.7 at all time points except 2 and 4 hours and AUC values > 0.8 were found at 36 hours (0.837), 24 hours (0.829), 72 hours (0.809) and 60 hours (0.807); no AUC value exceeded 0.9. AUC values varied among previous studies. Mörtberg et al.[16] reported an AUC value of 0.86 at 2 hours (cut-off value of 1.0 ug/L, sensitivity of 73% and specificity of 93%), 0.97 at 24 hours (cut-off value of 0.18 ug/L, sensitivity of 87% and specificity of 100%), which was the highest in their study. The timing of elevated AUC is similar to our results, but their predictive value was higher.
Stammet et al.[17] reported an AUC value of 0.84 (cut-off value of 0.03/0.3 ug/L, specificity of 100%, sensitivity of 76%, specificity of 78% and false positive rate of 22%). They reported the AUC values could increase to 0.95 and 0.91, respectively, when bispectral index or NSE was applied. Zellner et al.[18] reported an AUC value of 0/67 on admission and at 24 hours (cut-off value of 0.614/5.48 ug/L, sensitivity of 90%/18% and specificity of 31%/90%), 0.74 (cut-off value of 0.12/0.462 ug/L, sensitivity of 90%/35% and specificity of 50%/90%), stating their predictive value was not high. Tiainen et al.[11] compared patients who underwent TH and those with normal temperature (NT) and reported AUC values of 0.65 and 0.90, respectively, at 24 hours in the two groups (cut-off values of 0.21/0.19, sensitivity of 30%/59% and specificity of 100%/100%), 0.69/0.85 (TH/NT) at 36 hours (cut-off values of 0.21/0.49, sensitivity of 20%/18% and specificity of 96%/100%), 0.63/0.91 at 48 hours (cut-off values of 0.21/0.12, sensitivity of 22%/88% and specificity of 96%/100%), showing a higher predictive value in the NT group than the TH group. Their predictive value was lower than in the present study.
AUC values of serum S-100B level ranged from 0.63–0.97 depending on measured time points in previous studies and the AUC was higher at 24, 36 and 48 hours. However, the measurement period was shorter than the extended time course covering 60 and 72 hours in the present study, rendering the comparison difficult. However, the timing of elevated AUC is similar across the studies.
In the present study, cut-off values for S-100B ranged from 0.095 (48 hours) to 1.01 ug/L (0 hour) showing the ranges of sensitivity from 65.0% (36 hours) to 90.5% (12 hours), specificity from 52.9% (12 hours) to 94.1% (36 hours), PPV from 66.7% (4 hours) to 92.9% (36 hours) and NPV from 62.5% (4 hours) to 86.7% (72 hours). The cut-off value decreased within 4 hours and remained at similar levels thereafter. Regarding poor outcomes predicted by a specificity of 100%, the cut-off value ranged from 0.432 (48 hours) to 7.25 ug/L (0 hour). This elevated value at 0 hr rapidly increased within 24 hrs and remained at similar levels over 60 hours before rising again. The corresponding sensitivity ranged from 4.5% (2 and 4 hours) to 45% (48 hours) and NPV from 46.2% (2 and 4 hours) to 60.7% (48 hours). In previous studies, the cut-off values ranged from 0.03–2.35 ug/L, but the range increased to 0.21–5.48 ug/L depending on time points with 100% specificity. The cut-off values calculated in the present study are not consistent with these ranges, but all cut-off values showed a similar pattern of increasing at early time points and decreasing over time. Sensitivity and specificity values were similar in previous studies while showing high sensitivity at 24 hours.
S-100B protein levels had relatively good prognostic values at all time points. The prognostic value range was lower than reported in a previous study conducted with patients with NT but similar with a study comparing TH and NT groups. The prognostic values for neurological outcomes began to rise after 12 hours and continued for 96 hours. The optimal prediction time points were 24 and 36 hours showing AUC values of 0.829/0.837, respectively, cut-off values (100% specificity) of 0.221/0.249 (0.612/0.685 ug/L), sensitivity (100% specificity) of 75/65% (40/35%), specificity of 82.35/94.1%, PPV of 83.3/92.9% and NPV (PPV100%) of 73.7/69.6% (58.6/56.7%). Because AUC values increased to > 0.8 at 60 and 72 hours, these time points can be considered appropriate measurement times.
Changes in S-100B protein levels and measured time points found in the present study are consistent with the findings in previous studies. However, protein predictive values, cut-off values, sensitivity and specificity differed. The discrepancies are likely due to different baseline characteristics, including initial cardiac rhythms, causes and duration of CA, baseline with followed measured time points, control group and statistical programs among studies.
In the present study, a novel approach was taken to understand the changes in serum S-100B protein over an extended measurement period in which serum samples were collected frequently over a longer period of time compared with previous studies. In addition to the identification of specific and accurate time-dependent changes in serum S-100B protein associated with prognosis, we set the ROSC as the starting point for standardized measurements and performed frequent measurements during the extended period to suggest adequate monitoring time points for prediction accuracy.
The present study had several limitations. First, regional and social differences cannot be eliminated because the study was conducted at a hospital, rendering the representative nature of the sample uncertain. Second, the study results may be subject to variations in serum levels due to a small sample size. Third, the results are subject to variations in sampling time because the time from CA to ROSC varied among patients and between groups. Additionally, due to inferior pre-hospital emergency care, comparing the results of the present study with the findings of earlier studies is difficult, particularly with those conducted in developed countries. Finally, TH treatment was likely to affect serum S-100B protein level and variations in the time taken to induce hypothermia and duration might affect the results of the study. Further studies are necessary to determine changes in the protein levels between pre- and post-TH. To overcome these limitations, a standardized study design should be established to promote multicenter research aimed to improve understanding of the changes in biochemical markers and their prognostic importance in patients after CA.
In conclusion, the present study found a statistically significant association between serum S-100B protein levels and neurological outcomes in patients who underwent TH after ROSC showing a high predictive value of the protein. Thus, S-100B is confirmed to be a valid early marker for neurological damage. Based on the study results, the authors suggest 24 and 36 hrs as the optimal time points for measurement of S-100B protein for predicting unfavorable neurological outcomes. Further large scale, multicenter studies are necessary to determine more appropriate cut-off values.
References
1). Sasson C, Rogers MA, Dahl J, Kellermann AL. Predictors of survival from out-of-hospital cardiac arrest: a systematic review and meta-analysis. Circ Cardiovasc Qual Outcomes. 2010; 3:63–81.
2). Nolan JP, Laver SR, Welch CA, Harrison DA, Gupta V, Rowan K. Outcome following admission to UK intensive care units after cardiac arrest: a secondary analysis of the ICNARC case mix programme database. Anaesthesia. 2007; 62:1207–16.


3). Lee MG, Kim SJ, Choi DH, Jun DH, Yoo BD, Lee DP. Outcome of nontraumatic prehospital cardiac arrest. J Korean Soc Emerg Med. 2002; 13:428–33.
4). Kim SE, Eo EK, Cheon YJ, Jung KY, Park HS. Outcome in a tertiary emergency department for cardiopulmonary resuscitation for out-of-hospital cardiac arrest. J Korean Soc Emerg Med. 2005; 16:495–504.
5). Park KN, Choi SP, Lee MJ, Kim YM, Choi KH, Sung CH, et al. Clinical course of hypoxic-ischemic coma after cardiopulmonary resuscitation in Korea. J Korean Soc Emerg Med. 2005; 16:327–33.
6). Nolan JP, Neumar RW, Adrie C, Aibiki M, Berg RA, Bbttiger BW, et al. Post-cardiac arrest syndrome: epidemiology, pathophysiology, treatment, and prognostication: a scientific statement from the international liaison committee on resuscitation; the American heart association emergency cardiovascular care committee; the council on cardiovascular surgery and anesthesia; the council on cardiopulmonary, perioperative, and critical care; the council on clinical cardiology; the council on stroke. Resuscitation. 2008; 79:350–79.
7). Madl C, Grimm G, Kramer L, Yeganehfar W, Sterz F, Schneider B, et al. Early prediction of individual outcome after cardiopulmonary resuscitation. Lancet. 1993; 341:855–8.


8). Hypothermia after Cardiac Arrest Study Group. Mild therapeutic hypothermia to improve the neurologic outcome after cardiac arrest. N Engl J Med. 2002; 346:549–56.
9). Bernard SA, Gray TW, Buist MD, Jones BM, Silvester W, Gutteridge G, et al. Treatment of comatose survivors of out-of-hospital cardiac arrest with induced hypothermia. N Engl J Med. 2002; 346:557–63.


10). Wijdicks EF, Hijdra A, Young GB, Bassetti CL, Wiebe S; Quality Standards Subcommittee of the American Academy of Neurology. Practice parameter: prediction of outcome in comatose survivors after cardiopulmonary resuscitation (an evidence-based review): report of the quality standards subcommittee of the American academy of neurology. Neurology. 2006; 67:203–10.


11). Tiainen M, Roine RO, Pettilä V, Takkunen O. Serum neuron-specific enolase and S-100B protein in cardiac arrest patients treated with hypothermia. Stroke. 2003; 34:2881–6.


12). Hachimi-Idrissi S, Zizi M, Nguyen DN, Schiettecate J, Ebinger G, Michotte Y, et al. The evolution of serum astroglial S-100 beta protein in patients with cardiac arrest treated with mild hypothermia. Resuscitation. 2005; 64:187–92.
13). Derwall M, Stoppe C, Brücken D, Rossaint R, Fries M. Changes in S-100 protein serum levels in survivors of out-of-hospital cardiac arrest treated with mild therapeutic hypothermia: a prospective, observational study. Crit Care. 2009; 13:R58.
14). Rundgren M, Karlsson T, Nielsen N, Cronberg T, Johnsson P, Friberg H. Neuron specific enolase and S-100B as predictors of outcome after cardiac arrest and induced hypothermia. Resuscitation. 2009; 80:784–9.


15). Wennervirta JE, Ermes MJ, Tiainen SM, Salmi TK, Hynninen MS, Särkelä MO, et al. Hypothermia-treated cardiac arrest patients with good neurological outcome differ early in quantitative variables of EEG suppression and epileptiform activity. Crit Care Med. 2009; 37:2427–35.


16). Mörtberg E, Zetterberg H, Nordmark J, Blennow K, Rosengren L, Rubertsson S. S-100B is superior to NSE, BDNF and GFAP in predicting outcome of resuscitation from cardiac arrest with hypothermia treatment. Resuscitation. 2011; 82:26–31.


17). Stammet P, Wagner DR, Gilson G, Devaux Y. Modeling serum level of S-100β and bispectral index to predict outcome after cardiac arrest. J Am Coll Cardiol. 2013; 62:851–8.
18). Zellner T, Gärtner R, Schopohl J, Angstwurm M. NSE and S-100B are not sufficiently predictive of neurologic outcome after therapeutic hypothermia for cardiac arrest. Resuscitation. 2013; 84:1382–6.


19). Gray WA, Capone RJ, Most AS. Unsuccessful emergency medical resuscitation--are continued efforts in the emergency department justified? N Engl J Med. 1991; 325:1393–8.
20). Hamel MB, Phillips R, Teno J, Davis RB, Goldman L, Lynn J, et al. Cost effectiveness of aggressive care for patients with nontraumatic coma. Crit Care Med. 2002; 30:1191–6.


21). Geocadin RG, Buitrago MM, Torbey MT, Chandra-Strobos N, Williams MA, Kaplan PW. Neurologic prognosis and withdrawal of life support after resuscitation from cardiac arrest. Neurology. 2006; 67:105–8.


22). Booth CM, Boone RH, Tomlinson G, Detsky AS. Is this patient dead, vegetative, or severely neurologically impaired? Assessing outcome for comatose survivors of cardiac arrest. JAMA. 2004; 291:870–9.
Fig. 1.
Serial changes of S-100B protein level (total patients, n = 40). ROSC: return of spontaneous circulation.
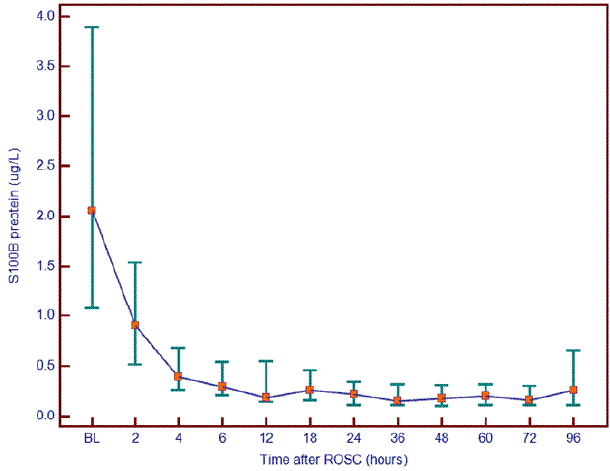
Fig. 2.
Serial changes of S-100B protein level according to neurological outcomes (Good CPC, n = 18 vs. Poor CPC, n = 22), data were presented by median value with 95% confidence interval. *p < 0.01, †p < 0.05. ROSC: return of spontaneous circulation; CPC: cerebral performance category.
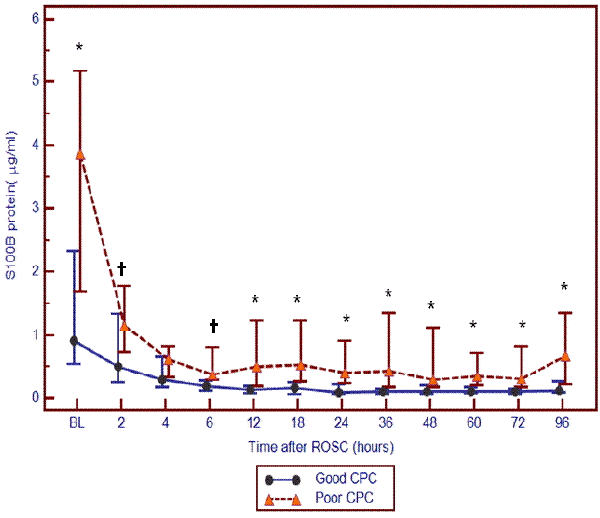
Fig. 3.
Receiver operating characteristic curve of S-100B protein level at 24 hrs for the prediction of poor cerebral performance category.
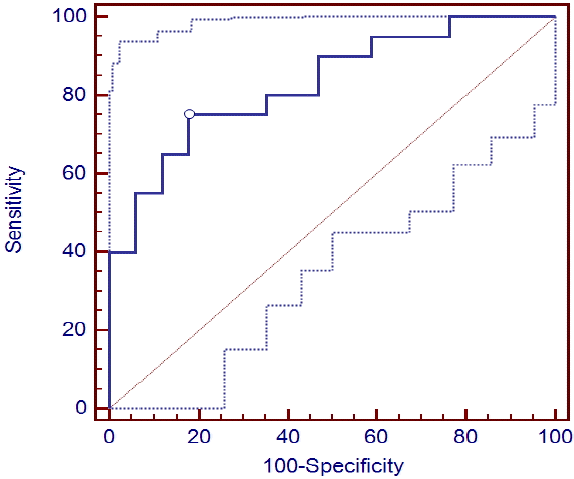
Fig. 4.
Receiver operating characteristic curve of S-100B protein level at 36 hrs for the prediction of poor cerebral performance category.
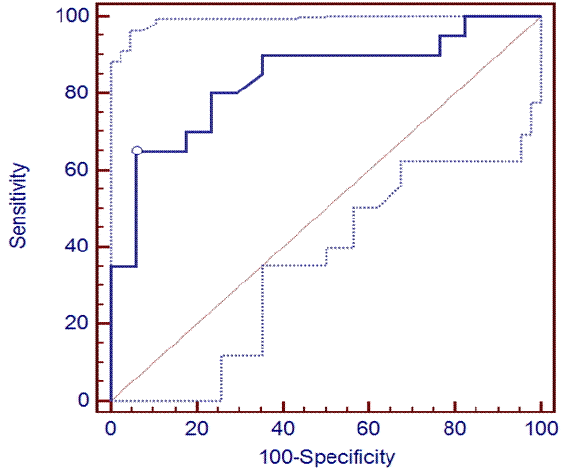
Table 1.
Baseline characteristics of the study population (n = 40)
Values are expressed as number (percentage) and median (interquartile range) as appropriate. CPR: cardiopulmonary resuscitation; BLS: basic life support; ACLS: advanced life support; ROSC: return of spontaneous circulation; APACHE: Acute Physiology and Chronic Health Evaluation; SOFA: Sequential Organ Failure Assessment; TH: therapeutic hypothermia; BL: baseline.
Table 2.
Comparison of serum S-100B protein levels between good and poor outcome patients
Table 3.
ROC analysis of S-100B level for the prediction of poor neurological outcomes (n = 40)