Abstract
When patients with severe respiratory failure are treated with venovenous extracorporeal membrane oxygenation (VV-ECMO), severe pulmonary hypertension due to right ventricular (RV) failure is possible. This is a serious complication that requires immediate therapeutic intervention. We report an extraordinary experience of additional venoarterial extracorporeal membrane oxygenation (VA-ECMO) support for RV failure in a patient who was being treated with VV-ECMO as a bridge to lung transplantation. A 61-year-old man was diagnosed with acute exacerbation of idiopathic pulmonary fibrosis. While waiting for lung transplantation, he was placed on VV-ECMO and developed RV failure. After insertion of additional VA ECMO, RV dysfunction was dramatically improved. He underwent heart-lung transplantation after 23 days of dual ECMO support.
Lung transplantation is gradually becoming a worldwide standard treatment for patients with a broad spectrum of end-stage respiratory diseases.[1] However, the mortality rate of patients awaiting lung transplantation is higher than those waiting for other solid organs. Approximately 15% of patients on the waiting list have died each year while awaiting transplantation.[2] In such patients, venovenous extracorporeal membrane oxygenation (VV-ECMO) can be the inevitable therapeutic option for survival, especially for refractory ventilatory failure patients. However, right ventricular (RV) failure could occur during treatment with VV-ECMO.[3] This is a serious complication with a high mortality that requires immediate intervention, including additional inotropic agents, hybrid ECMO configuration such as veno-arterial and venous (VAV) cannulation, or complete transition to veno arterial extracorporeal membrane oxygenation (VA-ECMO).[4,5] Herein, we report our extraordinary experience of additional VA-ECMO support for RV failure in patients who were being treated with VV-ECMO as a bridge to lung transplantation. To our knowledge, this is the first case of a successful bridge to transplantation through the dual ECMO support.
A 61-year-old man was admitted to emergency room with 1-weak history of dyspnea. His symptoms included a productive cough and chest pain. He had been diagnosed as pathologically proved idiopathic pulmonary fibrosis (IPF) with usual interstitial pneumonia 7 years ago. Since being diagnosed with IPF, he had been being given systemic corticosteroid, but his chest radiography revealed diffuse ground-glass opacity and reticular opacity in both lungs (Fig. 1). Chest computed tomography (CT) revealed increased extent of reticular opacity, traction bronchiectasis and newly developed multifocal ground glass opacity lesions in both lungs in comparison with previous CT. Based on examination result, we diagnosed acute exacerbation of IPF. Empiric broad-spectrum intravenous antibiotics were initiated for presumed community-acquired pneumonia and doses of systemic corticosteroid were increased. But he developed progressive hypoxemia requiring 100% oxygen through high flow nasal cannula. Because the improvement of his condition was not expected, we decided to perform lung transplantation. He was admitted to the intensive care unit and began receiving mechanical ventilation. However, He developed worsening hypoxemia and hypercarpnia while waiting for transplantation, despite advanced mechanical ventilation with increased PEEP, increased mean airway pressures, and inhaled nitric oxide.
Finally, he was placed on VV-ECMO and cannulated via bilateral femoral veins. Although hypoxemia and hypercarpnia was improved, right heart function began to worsen after 23 days of ECMO. Liver function was also progressively aggravated and progressive hypoxemia occurred in spite of full VV-ECMO support. We ruled out the possibility of pulmonary thromboembolism because anticoagulation with heparin was being done and the level of D-dimer was not elevated. At 29 days of VV-ECMO duration, we performed echocardiography for the evaluation of RV function. Echocardiography revealed D-shape of left ventricle (LV) with severe RV dysfunction. RV cavity size increased from 3.2 cm to 5.4 cm and RV systolic pressure was elevated from 59.76 mmHg to 94.92 mmHg. Although LV systolic function and cavity size was normal, flattening of the inter-ventricular septum was found. We added inotropic agents such as epinephrine and milrinone but RV dysfunction was not improved. On the 35th day of VV-ECMO, we decided to add VA-ECMO for RV decompression. He was cannulated via right internal jugular vein (for drainage) and right femoral artery (for return) (Fig. 2). Initial setting of each ECMO was as following. VV-ECMO: sweep 5, flow 3.6 L/min, 2,750 RPM, FiO2 1.0; VA-ECMO: sweep 2, flow 2.0 L/min, 2,050 RPM, FiO2 1.0 (Fig. 3). After adding VA-ECMO, hypoxemia was immediately improved. PaO2 and SpO2 increased from 46.3 mmHg/85.1% to 85.6 mmHg/96.2% (Table 1). Because vascular ultrasonography showed a decreased blood flow below right popliteal artery, we inserted a catheter for distal perfusion through right superficial femoral artery. Two days later, we performed follow up echocardiographic examination and it revealed that RV dysfunction was also dramatically improved. RV cavity size decreased from 5.4 cm to 4.4 cm and RV systolic pressure decreased from 94.92 mmHg to 65.13 mmHg (Fig. 4). And liver function was improved, too (Table 1).
After additional 23 days of dual ECMO support, he underwent heart-lung transplantation with proper mental state and no evidence of multi-organ failure. During the total 58 days of ECMO support, three oxygenators were exchanged and there was no major complication except superficial venous thrombosis at early phase of VV-ECMO. Unfortunately, the operation was problematic. Extensive adhesiolysis was performed because of severe pleural adhesion. It prolonged operative time and worsened function of transplanted heart. On postoperative day 1, the patient expired due to postoperative bleeding despite massive transfusion.
When patients with severe respiratory failure are treated with VV-ECMO, severe pulmonary hypertension due to RV failure could develop.[3] Although the incidence of RV failure during VV-ECMO has not been known exactly, this is a serious complication that result in poor prognosis[4] and requires immediate therapeutic intervention. Current management of RV failure includes inotropic support to enhance RV contractility, pulmonary vasodilation to reduce RV afterload, and volume resuscitation to maintain RV preload.[6–8] However, in hemodynamically unstable patients, this medical intervention is not sufficiently effective. Balloon atrial septostomy can improve hemodynamics in patients with RV failure by unloading the RV.[9] However, current data indicate that patients with severe RV failure and cardiogenic shock respond poorly to balloon atrial septostomy.[10] Therefore, mechanical support is essential in patients with life-threatening RV failure. In such cases, use of the right ventricular assist device (RVAD) has been described.[11] However, use of the RVAD support in severe pulmonary hypertension resulted in severe pulmonary hemorrhage and even further elevation of the pulmonary artery (PA) pressure higher than systemic blood pressure.
In a recent report, replacing the RVAD by VA-ECMO improved hemodynamics and decreased the rate of pulmonary hemorrhage. Berman and colleagues[5,12] have commented that VA-ECMO may be the optimal strategy in RV failure cases resulting from pressure overload caused by pulmonary hypertension. Use of the VA-ECMO will decompress the RV, decrease the PA pressure and facilitate preservation and recovery of end-organ function.[5,11] For this reason, in such patients who were placed on VV-ECMO, conversion to VA- from VV-ECMO may be considered. However, in case of VA-ECMO, differential hypoxemia, which is also known as “blue head syndrome”,[13] can develop in patients with irreversible respiratory failure and normal LV systolic function. One solution is to place another venous return catheter in the internal jugular vein to allow oxygenated blood to flow through the heart (VAV-ECMO). This configuration can improve oxygen delivery to the upper body. However, it relies on adequate cardiac function, which is problematic in decompensated pulmonary arterial hypertension.[14] Additionally, because VAV-ECMO have two outflow catheters, it is also difficult to regulate arterial and venous outflow, respectively. Therefore, it might be alternative solution to add another VA-ECMO to the patient who had already been placed on VV-ECMO like our patient (dual ECMO). As compared with only VA-ECMO, dual ECMO can lower the possibility for development of differential hypoxemia, and have advantage of making it easier to regulate each ECMO outflow in comparison with hybrid VAV-ECMO. We were easily able to regulate each ECMO outflow and find an optimal flow. Through the dual ECMO support, we could decompress RV and simultaneously improve systemic oxygenation without worrying about development of differential hypoxemia.
In summary, we reported our extraordinary experience of successful bridge to transplantation in a patient who was supported by dual ECMO. Because this was the first successful case using dual ECMO, we can hardly conclude that this pioneering attempt will be also effective for other patients with the same condition. Additionally, because dual ECMO support requires two ECMO machines for only one patient, the problem of medical cost and resource utilization should be considered. However, we suggest that dual ECMO might be an option for the management of RV failure developed during VV-ECMO support in acute respiratory distress syndrome (ARDS) patient.
References
1). Cooper JD, Pearson FG, Patterson GA, Todd TR, Ginsberg RJ, Goldberg M, et al. Technique of successful lung transplantation in humans. J Thorac Cardiovasc Surg. 1987; 93:173–81.


2). Valapour M, Skeans MA, Heubner BM, Smith JM, Schnitzler MA, Hertz MI, et al. OPTN/SRTR 2012 Annual Data Report: lung. Am J Transplant. 2014; 14(Suppl 1):139–65.


3). Abstracts of the 23rd Annual European Society of Intensive Care Medicine (ESICM) Congress. Barcelona, Spain. October 9–13, 2010. Intensive Care Med. 2010; 36(Suppl 2):86–433.
4). D'Alonzo GE, Barst RJ, Ayres SM, Bergofsky EH, Brundage BH, Detre KM, et al. Survival in patients with primary pulmonary hypertension: Results from a national prospective registry. Ann Intern Med. 1991; 115:343–9.
5). Berman M, Tsui S, Vuylsteke A, Klein A, Jenkins DP. Life-threatening right ventricular failure in pulmonary hypertension: RVAD or ECMO? J Heart Lung Transplant. 2008; 27:1188–9.


6). Piazza G, Goldhaber SZ. The acutely decompensated right ventricle: pathways for diagnosis and management. Chest. 2005; 128:1836–52.
7). MacLaren G, Combes A, Bartlett RH. Contemporary extracorporeal membrane oxygenation for adult respiratory failure: Life support in the new era. Intensive Care Med. 2012; 38:210–20.


8). Green EM, Givertz MM. Management of acute right ventricular failure in the intensive care unit. Curr Heart Fail Rep. 2012; 9:228–35.


9). Hopkins WE, Ochoa LL, Richardson GW, Trulock EP. Comparison of the hemodynamics and survival of adults with severe primary pulmonary hypertension or Eisenmenger syndrome. J Heart Lung Transplant. 1996; 15:100–5.
10). Rich S, Dodin E, McLaughlin VV. Usefulness of atrial septostomy as a treatment for primary pulmonary hypertension and guidelines for its application. Am J Cardiol. 1997; 80:369–71.


11). Gregoric ID, Chandra D, Myers TJ, Scheinin SA, Loyalka P, Kar B. Extracorporeal membrane oxygenation as a bridge to emergency heart-lung transplantation in a patient with idiopathic pulmonary arterial hypertension. J Heart Lung Transplant. 2008; 27:466–8.


12). Berman M, Tsui S, Vuylsteke A, Snell A, Colah S, Latimer R, et al. Successful extracorporeal membrane oxygenation support after pulmonary thromboendarterectomy. Ann Thorac Surg. 2008; 86:1261–7.


13). Kitamura M, Shibuya M, Kurihara H, Akimoto T, Endo M, Koyanagi H. Effective cross-circulation technique of venoarterial bypass for differential hypoxia condition. Artif Organs. 1997; 21:786–8.


14). Abrams DC, Brodie D, Rosenzweig EB, Burkart KM, Agerstrand CL, Bacchetta MD. Upper-body ECMO as a strategy in decompensated pulmonary arterial hypertension. Pulm Circ. 2013; 3:432–5.
Fig. 2.
(A) Chest and (B) plain abdominal radiography showing four catheters of dual extracorporeal membrane oxygenation (ECMO). V: venous catheter of venoarterial extracorporeal membrane oxygenation (VA-ECMO) through right internal jugular vein; A: arterial catheter of VA-ECMO through right femoral artery; Vin: inflow catheter of venovenous extracorporeal membrane oxygenation (VV-ECMO) through right femoral vein; Vout: outflow catheter of VV-ECMO through left femoral vein.
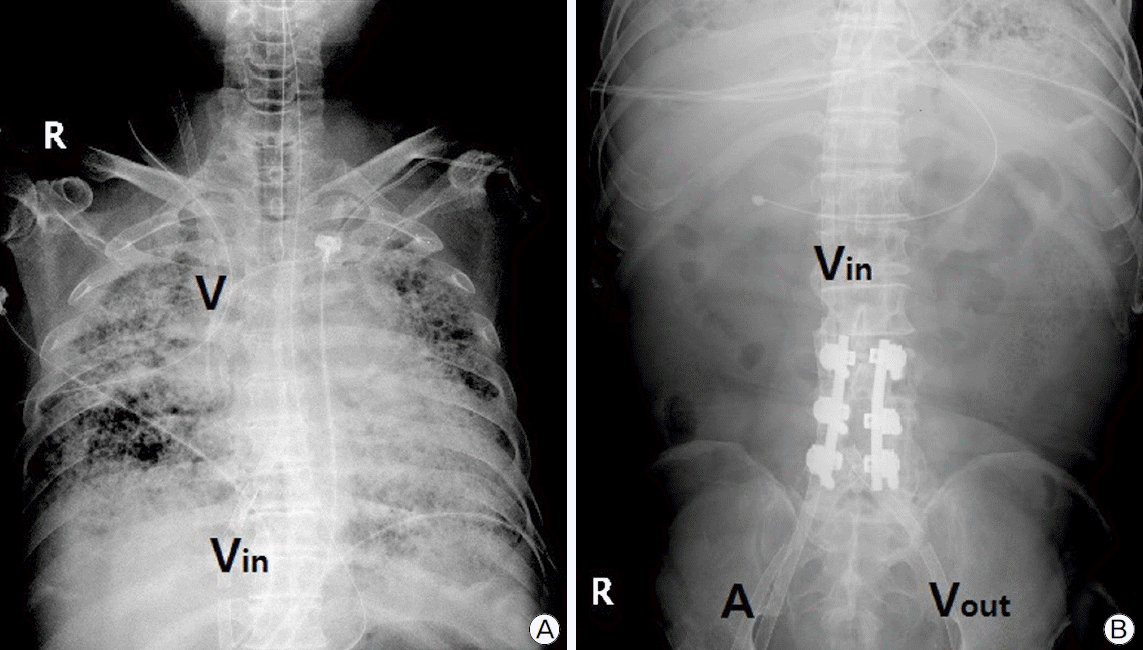
Fig. 3.
Conceptual diagram of dual extracorporeal membrane oxygenation. ARDS: acute respiratory distress syndrome; RV: right ventricule; LV: left ventricle; VA: venoarterial; VV: venovenous; ECMO: extracorporeal membrane oxygenation.
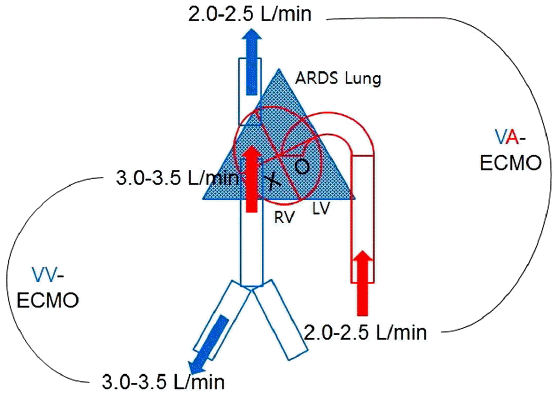
Fig. 4.
Measurement of right ventricular systolic pressure (A) before and (B) after dual extracorporeal membrane oxygenation support.
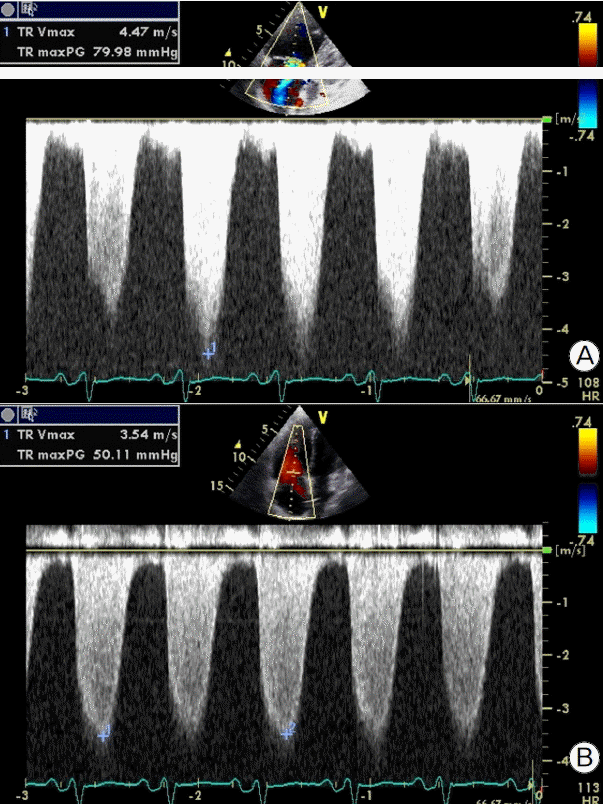
Table 1.
Changes in clinical parameters
VV: venovenous; ECMO: extracorporeal membrane oxygenation; FiO2: fraction of inspired oxygen; PIP: peak inspiratory pressure; PEEP: positive end-expiratory pressure; ABGA: arterial blood gas analysis; AST: aspartate aminotransferase; ALT: alanine aminotransferase; IU: international unit; RVSP: right ventricular systolic pressure.