Abstract
Purpose
This study aims to investigate the feasibility of contouring target volume according to residual tumor and decreasing the dose to the tumor regression field after induction chemotherapy (IC) in locoregionally advanced nasopharyngeal carcinoma (NPC).
Materials and Methods
From August 2009 to August 2013, patients with stage III–IVB NPC were treated with IC and concurrent chemoradiotherapy. Gross tumor volume of nasopharynx (GTVnx)–residual and gross tumor volume of cervical lymph node (GTVnd)–residual were contoured according to post-IC residual primary tumor and any N+ disease, respectively. The tumor regression field was included in CTVnx1/CTVnd1 and prescribed a dose of 60 Gy. Outcomes and toxicities of all patients were evaluated.
Results
A total of 57 patients were enrolled. At a median follow-up of 68 months, three cases displayed locoregional recurrence and one case showed both distant metastasis and locoregional recurrence. All locoregional recurrences were in the GTVnx-residual/GTVnd-residual and in-field. The 5-year overall, locoregional relapse-free, distant metastasis-free, and progression-free survival rates were 82.2%, 87.7%, 85.8% and 80.3%, respectively.
The incidence of nasopharyngeal carcinoma (NPC) is high in south China and Southeast Asia [1]. Radiotherapy is the standard treatment modality, and a combination of radiotherapy and chemotherapy employed as the mainstay strategy [2]. Currently, induction chemotherapy (IC) combined with concurrent chemoradiotherapy is one of the most popular treatments for locoregionally advanced NPC, with confirmed therapeutic value. A phase III clinical trial [3] comparing the efficacy of IC plus concurrent chemoradiotherapy to that of chemoradiotherapy alone for treatment of locoregionally advanced NPC conducted by Sun et al. [3] showed that inclusion of IC significantly improves progression-free survival (PFS; 3-year PFS, 80% vs. 72%). The addition of IC effectively reduced distant metastasis, which could be transformed into overall survival (OS) benefit. In addition, multiple studies have reported a response rate of 73%-89% with significant tumor volume decreasing in NPC after IC [4-6], leading to challenge of target delineation for following radiotherapy.
Currently, accurate definition of the target volume of NPC after IC presents a major clinical challenge and remains a subject of extensive debate. According to Perez & Brady's Principles and Practice of Radiation Oncology, 6th edition [7], target volumes should be defined based on the extent of the tumor before IC. In 2009, the consensus [8] was that gross tumor volume (GTV) should be outlined based on the pre-IC tumor volume (cavity partially retracted) in locoregionally advanced head-and-neck cancer after IC and the irradiation dose should remain constant, regardless of whether or not the lesions achieve complete remission. However, evidencebased reports are lacking in the literature. According to the basic principles of radiobiology [7], the tumor control probability curve suggests that therapeutic radiation dose should be increased with a higher tumor burden to achieve radical treatment for clinically detectable tumors. Conversely, once gross tumor lesions transform into subclinical lesions that are undetectable with imaging methods after IC, the tumor burden decreases and the radiation dose may need to be altered accordingly. For other malignancies, such as lymphoma [9], small cell and non-small cell lung cancer [10,11], earlier studies have shown that reducing the dose of radiation therapy in the tumor regression areas after IC does not adversely affect efficacy. Furthermore, reduction of the radiation dose led to decreased toxicity, which improved the quality of life in patients.
In summary, for treatment of locoregionally advanced NPC, it is unreasonable to define the target volume after IC as the pre-IC volume, especially for cases showing significant tumor regression after IC. In the current study, methods for outlining the accurate target volume of NPC after IC and feasibility of appropriate reduction of the radiation dose in the tumor regression area were assessed, and follow-up analysis of patients conducted to determine long-term treatment efficacy and toxicity outcomes.
Patients were eligible if they had (1) biopsy-proven previously untreated World Health Organization type II or III NPC; (2) stage III–IVB according to the American Joint Committee on Staging of Cancer classification (AJCC 2009); (3) ages between 18 and 65 years; (4) absence of a second malignancy; (5) Karnofsky performance score ≥ 70; (6) adequate bone marrow reserve, liver function and renal function; and (7) adequate mental status to follow instructions.
Before treatment, all patients underwent bone scan, chest X-ray, abdominal ultrasound and electrocardiography assessments. Clinical examination of the head and neck, magnetic resonance imaging (MRI) scan of the nasopharynx and neck and electronic nasopharyngoscopy were performed before IC and after two and four cycles of IC.
The regimen of IC is a combination of taxanes, cisplatin and fluorouracil (TPF), comprising intravenous infusion of docetaxel 50 mg/m2 on day 1, intravenous infusion of cisplatin 60 mg/m2 on day 1 and continuous intravenous infusion of 5-fluorouracil 500 mg/m2/day through days 1-5 for 120 hours, three-weekly, for four cycles. If tumor shrinkage achieved partial remission or above after two cycles of IC, patients continued IC to four cycles and were administered concurrent chemoradiation (CCRT); otherwise, patients received CCRT after two cycles of IC. Concurrent chemotherapy (CCT) consisted of cisplatin 30 mg/m2 intravenous infusion on day 1, weekly, for 4-6 cycles.
Prior to radiotherapy, all patients were immobilized with head-and-neck thermoplastic masks in a supine position, followed by computed tomography simulation using 3-mm slices including the head and neck within 1 to 2 weeks after IC. All patients received definitive intensity-modulated radiation therapy (IMRT) in 3 weeks after IC.
Target volumes and organs at risk (OAR) were determined according to the International Commission on Radiation Units and Measurements reports (ICRU) 50 and 62 as well as our institutional treatment protocol [12-14]. The gross tumor volume of nasopharynx (GTVnx)–pre and the gross tumor volume of cervical lymph node (GTVnd)–pre referred to the primary tumor (including retropharyngeal lymph node metastasis) and the neck lymph node metastasis before IC, respectively. The GTVnx-residual was defined as the residual primary tumor (including retropharyngeal lymph node metastasis) after IC, and the GTVnd-residual was defined as the residual GTVnd-pre after IC. The CTVnx1 and CTVnd1 were defined as GTVnx-residual and GTVnd-residual plus 1 cm margin and 0.5-1 cm margin, respectively. When the tumor debulked so much that the CTVnx1 and CTVnd1 were not able to encompass the region of GTVnx-pre and GTVndpre, respectively, the CTVnx1 and CTVnd1 should be modified to cover the region of GTVnx-pre and GTVnd-pre, respectively. CTV2 was defined as CTVnx1 and CTVnd1 plus a 5-mm margin together with the bilateral cervical selective lymph drainage areas. In cases where the primary tumor achieved complete remission after IC, the final site of tumor regression was contoured as GTVnx-residual. If the lymph node achieved complete remission after IC, the regressed lymph node and invaded structures were outlined as CTVnd1. Planning target volume (PTV) would encompass the corresponding GTVs and clinical target volumes (CTVs) with a 3-mm margin in all directions including anterior, posterior, superior, inferior, left and right. The GTVnx-pre and GTVnd-pre were the pre-IC target volumes with no prescribed dose, thus, the PTVs of GTVnx-residual, GTVndresidual, CTVnx1, CTVnd1, and CTV2 were designated PGTVnx-residual, PGTVnd-residual, PCTVnx1, PCTVnd1, and PCTV2, respectively, and prescribed radiation doses of 68 Gy, 62-66 Gy, 60 Gy, 60 Gy, and 50 Gy, respectively, in 30 fractions. All treatments were delivered once daily for 5 days per week. The names, definitions and prescribed doses of the target volumes are listed in the Table 1, and examples of target delineation are presented in Figs. 1 and 2. A standard constraint set conforming to the Radiation Therapy Oncology Group (RTOG) 0225 protocol [15] was applied for optimization and evaluation. The aim was to achieve 95% of any PTV at or above the prescription dose, at least 95% of any PTV at 95% of the prescription dose, and no more than 10% of PGTVnx-residual/PGTVnd-residual at or above 110% of the prescription dose.
Tumor response after two and four cycles of IC was evaluated according to the Response Evaluation Criteria in Solid Tumors (RECIST), and classified as complete response (CR), partial response (PR), stable disease (SD), or progressive disease (PD). We characterized chemotherapy-related and radiotherapy-related toxic effects according to the Common Terminology Criteria for Adverse Events ver. 3.0. All patients were assessed weekly during treatment and followed up every 3 months during the first 2 years, every 6 months during the subsequent 3 years, and yearly thereafter until death.
Our primary endpoints were locoregional relapse-free survival (LRFS) and patterns of locoregional failure, classified as “in-field” if 95% recurrent tumor volume was within the 95% isodose, “marginal” if 20%-95% recurrent tumor volume was within the 95% isodose or “out-of-field” if < 20% recurrent tumor volume was within the 95% isodose [16]. Our secondary endpoints were OS, distant metastasis-free survival (DMFS) and PFS.
The Statistical Package for Social Sciences, ver. 25.0 (SPSS, IBM Corp., Armonk, NY) was employed for statistical analysis. Patient characteristics were summarized using the descriptive statistics. The paired t test (two-sided test, α=0.05) was applied to compare tumor volumes before and after IC. Survival rates were computed using the Kaplan-Meier method.
From August 2009 to August 2013, 57 patients were reviewed. Patient and tumor characteristics are presented in Table 2.
Chemotherapy was administered to all patients. In total, 51/57 patients (89.5%) completed four cycles of IC, two of 57 patients (3.5%) underwent CCRT after two cycles of IC due to the incidence of herpes zoster and grade 3 thrombocytopenia, and four of 57 (7%) were evaluated as SD after two cycles of IC and underwent a third course while waiting for CCRT. Overall, 46 of 57 patients (80.7%) received more than four cycles of CCT. Reasons for CCT discontinuation included mucositis, grade 3 leukopenia and grade 4 thrombocytopenia. All patients completed definitive IMRT.
During IC, four of 57 patients (6.0%) developed grade 3 leukopenia, one of 57 (1.8%) developed grade 3 thrombocytopenia and one of 57 (1.8%) developed herpes zoster. CCRT induced several severe toxic effects, such as grade 3 mucositis (10.5%), grade 3 xerostomia (1.8%), grade 3 leukopenia (17.5%), grade 3 thrombocytopenia (1.8%), and grade 4 thrombocytopenia (1.8%).
The post-IC tumor volume was significantly decreased (p < 0.01), compared with the pre-IC tumor volume (Table 3). After completion of IC, CR was observed in 10.5% patients, PR in 82.5% patients, and SD in 7% patients.
Follow-up was updated in October 2017 at a median follow-up time of 68 months (range, 9 to 93 months). Overall, nine patients showed progression, including three with locoregional relapse alone, five with distant metastasis alone, and one with both locoregional relapse and distant metastasis. All locoregional recurrences occurred in the GTVnxresidual/GTVnd-residual region and were in-field, with no development of marginal or out-of-field recurrence (Table 4). Ten patients died during follow-up, specifically, three with locoregional relapse alone, four with distant metastasis alone, one with locoregional relapse and distant metastasis, one with pancreatic cancer, and one of unknown reason. The 5-year LRFS, DMFS, PFS, and OS rates were determined as 87.7%, 85.8%, 80.3%, and 82.2%, respectively. The Kaplan-Meier estimates of LRFS, DMFS, PFS and OS are shown in Fig. 3.
Previous focus on the role of chemotherapy in treatment of NPC has been to reduce distant metastasis and increase the OS rate. However, limited information is available on outlining of the radiotherapy target volume for optimizing the post-IC response in NPC. Conclusions are yet to be made on how to define the target tumor volume and the appropriate irradiation dose after IC. The conventional view [8] is that the tumor regression field after IC is still defined as GTV and treated with the radical irradiation dose used for gross tumor. However, according to the basic principles of radiobiology [7,17], only a high tumor burden requires the higher dose of radiation therapy.
To achieve good treatment effects, subclinical lesions in NPC are usually recommended 60 Gy of radiation [6,18]. However, for gross tumors that have shrunk to subclinical lesions after chemotherapy, administration of the same irradiation dose as that for the gross tumor appears unreasonable. Therefore, determination of the tumor regression area after chemotherapy as a CTV and provision of an equivalent corresponding radiation dose is important. This modification should provide two main benefits to patients: (1) the highdose irradiation area is reduced, potentially resulting in a significant decrease in toxicity to patients, and (2) in tumors adjacent to the OAR, such as brain stem or optic chiasm, the tumor size is reduced by IC and the distance between the GTV and critical tissues increased, so that radical treatment is possible for patients who have only been able to undergo palliative care in the past.
For other malignant tumors, outlining target volumes after IC to reduce treatment-related toxicity has been discussed and a consensus reached. In lymphoma radiotherapy, involved node radiation therapy and involved site radiation therapy are the newest irradiation concepts with smaller radiation volumes and lower toxicity than the original larger-field radiation therapy and the classical involved-field radiation therapy [9]. The concept of involved node radiation therapy developed by the European Organization for Research on Treatment of Cancer [19] is that GTV is the lymph node remnant alone and CTV only includes the tumors that achieve CR after chemotherapy without preventive irradiation to other regions. Both retrospective and prospective studies have demonstrated achievement of equivalent or enhanced survival rates compared with involved-field radiation therapy, suggesting that the target volume after IC can be effectively decreased in accordance with tumor debulking [20-22]. There were similar explorations of reducing target volumes and prescribed doses in the treatment of non-small cell lung cancer [23] and small cell lung cancer [10].
A few researchers have explored methods for outlining the target tumor volume after IC for NPC. Yu et al. [24] defined clinically detectable lesions after IC as GTV and prescribed a dose of 68 Gy, while 63 Gy was administered to the tumor shrinkage area after IC. The local control rate was 100% at the median follow-up time of 9 months. Among 24 patients, only one showed distant metastasis 15 months after the completion of treatment. Xue et al. [25] outlined GTV according to the clinically detectable tumor area after IC and prescribed a dose of 60 Gy in the tumor shrinkage and upper neck drainage areas. The local control rate at three years in 103 patients was 91.9%. These results collectively support the feasibility of reducing the radiation dose for the tumor regression area after IC without compromising the therapeutic effect. In a prospective randomized controlled trial by Yang et al. [26], a prescribed dose of 64 Gy was administered in the tumor regression area after IC. No significant differences in 3-year OS, PFS, LRFS, and DMFS were evident between the experimental and control groups (87% vs. 82.3%, 83.4% vs. 74.7%, 93.9% vs. 91.8%, and 88.6% vs. 81.3%, respectively). In addition, treatment toxicity was significantly reduced.
In our study, a dose of 60 Gy was prescribed for the tumor regression area. Long-term follow-up revealed 5-year OS, DMFS, LRFS, and PFS rates of 82.2%, 85.8%, 87.7%, and 80.3%, respectively, analogous to reports in the past. It should be noted that patients enrolled in the study had stage III-IVB disease, most with large primary tumors and extensive nodal involvement (stage N2-3). Only four cases showed locoregional relapse in 5 years. All local recurrences were determined as GTVnx-residual or GTVnd-residual and recurrences located in-field. No recurrence within CTVnx1, CTVnd1, or CTV2 was observed, either marginal or outof-field. These results confirmed the feasibility of outlining the target volume and altering the prescription dose accordingly for optimizing the effects of radiation therapy.
For NPC adjacent to brain stem, optic chiasm, optic nerve and other important OARs, IC could aid in increasing the distance between the tumor and organ, allowing the use of radiotherapy as radical treatment instead of palliative care. For the example of Fig. 2, the tumor invaded ethmoidal cellules and orbital apex, with the right optic nerve suspiciously invaded. If the target area was outlined according to the pre-IC tumor, the choice would be to either sacrifice the dose coverage of the tumor or optic nerve function. A radical treatment would be compromised, and visual impairment may occur after treatment. However, after four IC cycles, the tumor area had diminished and the GTV area decreased. The shrinkage area altered from GTVnx-pre to CTVnx1. Accordingly, the prescribed dose was decreased, which not only achieved the purpose of radicalizing the tumor but also protected the right optic nerve and optic chiasma. Notably, even at 70 months after treatment, no locoregional recurrences were observed, along with no right eye vision impairment or other long-term toxicities.
Thus, with the reduced irradiation volume and corresponding dose, side-effects are also significantly reduced. Previous reports demonstrated a high brain damage rate in T3/4 NPC patients after radiotherapy, which had a significant impact on their quality of life. Zhou et al. [27] retrospectively analyzed radiation-related temporal lobe damage in NPC patients caused by IMRT and two-dimensional radiotherapy. Among 305 patients with NPC receiving IMRT, 16% showed radiation-induced TLI after 5 years, of which 15.5% T3 and 52.6% T4 patients had TLI. Su et al. [28] additionally conducted a retrospective analysis of patients with NPC undergoing IMRT. Five-year median follow-up studies revealed a TLI incidence in 14/83 T3 patients (16.9%) and 26/49 T4 patients (53.1%). However, analysis of long-term outcomes in our study showed that only 4/57 patients (7.0%) developed temporal lobe injury, all of which were in the T4 category. In addition, only two out of four displayed clinical symptoms. The current study included 40.4% T3 and 45.6% T4 patients. Our data showed a significantly decreased incidence of temporal lobe injury, compared to previous findings.
While patients with NPC clearly achieved a lower incidence of toxicity and better outcomes in our study, a number of issues still require attention in clinical practice. (1) We need to establish the optimal number of IC cycles required to effectively reduce the irradiation dose of the tumor regression area in NPC. Currently, no relevant recommended standards are available. In our analysis, if CR/PR was observed after two cycles of chemotherapy, treatment with IC was continued in terms of better tumor debulking and normal tissue repair, which contributed to better target delineation, as this would aid in determining the existence of residual tumor cells in the retracted area. Even after exposure to a higher number of IC cycles, toxicities were tolerable in patients and the distant metastasis rate would decrease. (2) Accurate evaluation of the tumor shrinkage area after chemotherapy is the key in radiotherapy target outlining. A multidisciplinary approach was used in which an imaging physician with extensive experience in reading head and neck tumor images was consulted to determine the extent of tumor. In cases where it was difficult to determine tumor volume, suspicious residual tumor was treated with the radical radiation dose. (3) For patients who achieved CR after chemotherapy, not all regression fields were treated as subclinical lesions. Instead, the center of primary tumor that regressed at the last cycle of IC was treated with high-dose radiation (68 Gy). The efficacy of 60 Gy irradiation treatment for the whole regression field including the primary tumor site in CR patients after chemotherapy remains to be established. (4) The number of enrolled patients was small because the main treatment modality for locoregionally advanced NPC was CCRT at the beginning of our study, and the exploration of IC was rare with only a few patients underwent IC combined with CCRT rather than CCRT alone. Besides, the target delineation after IC was debated, thus, this contouring method was only applied in our team with strict inclusion criteria considering the intensity of the treatment.
Except for these limitations, this investigation is a novel attempt to reduce the irradiation dose in the tumor shrinkage area after IC in NPC therapy. The long-term follow-up results showed a good therapeutic effect and low incidence of toxicity, supporting the theory that defining clinically and radiologically detectable tumors after IC as GTV while defining regression regions as subclinical lesions, presenting a feasible method for outlining the target region for radiotherapy. However, the sample size in our study was relatively small. Prospective randomized controlled clinical studies are thus further warranted to confirm our findings.
ACKNOWLEDGMENTS
This work was supported by grants from the Planned Science and Technology Project of Guangdong Province (No.2010B080701016 and No. 2012B031800301).
References
1. Razak AR, Siu LL, Liu FF, Ito E, O'Sullivan B, Chan K. Nasopharyngeal carcinoma: the next challenges. Eur J Cancer. 2010; 46:1967–78.


2. Pfister DG, Spencer S, Adelstein D, Adkins D, Brizel DM, Burtness BB, et al. NCCN clinical practice guidelines in oncology (NCCN Guidelines): head and neck cancers. Version 2.2016-October 11, 2016. Fort Washington, PA: National Comprehensive Cancer Network;2016.
3. Sun Y, Li WF, Chen NY, Zhang N, Hu GQ, Xie FY, et al. Induction chemotherapy plus concurrent chemoradiotherapy versus concurrent chemoradiotherapy alone in locoregionally advanced nasopharyngeal carcinoma: a phase 3, multicentre, randomised controlled trial. Lancet Oncol. 2016; 17:1509–20.
4. Hui EP, Ma BB, Leung SF, King AD, Mo F, Kam MK, et al. Randomized phase II trial of concurrent cisplatin-radiotherapy with or without neoadjuvant docetaxel and cisplatin in advanced nasopharyngeal carcinoma. J Clin Oncol. 2009; 27:242–9.


5. Fountzilas G, Ciuleanu E, Bobos M, Kalogera-Fountzila A, Eleftheraki AG, Karayannopoulou G, et al. Induction chemotherapy followed by concomitant radiotherapy and weekly cisplatin versus the same concomitant chemoradiotherapy in patients with nasopharyngeal carcinoma: a randomized phase II study conducted by the Hellenic Cooperative Oncology Group (HeCOG) with biomarker evaluation. Ann Oncol. 2012; 23:427–35.


6. Ma J, Mai HQ, Hong MH, Min HQ, Mao ZD, Cui NJ, et al. Results of a prospective randomized trial comparing neoadjuvant chemotherapy plus radiotherapy with radiotherapy alone in patients with locoregionally advanced nasopharyngeal carcinoma. J Clin Oncol. 2001; 19:1350–7.


7. Halperin EC, Brady LW, Perez CA, Wazer DE. Perez and Brady’s principles and practice of radiation oncology. 6th ed. Philadelphia, PA: Lippincott Williams &Wilkins;2013.
8. Salama JK, Haddad RI, Kies MS, Busse PM, Dong L, Brizel DM, et al. Clinical practice guidance for radiotherapy planning after induction chemotherapy in locoregionally advanced head-and-neck cancer. Int J Radiat Oncol Biol Phys. 2009; 75:725–33.


9. Illidge T, Specht L, Yahalom J, Aleman B, Berthelsen AK, Constine L, et al. Modern radiation therapy for nodal non-Hodgkin lymphoma-target definition and dose guidelines from the International Lymphoma Radiation Oncology Group. Int J Radiat Oncol Biol Phys. 2014; 89:49–58.


10. Kalemkerian GP, Loo BW Jr, Akerley W, Attia A, Chow LQ, Decker R. NCCN clinical practice guidelines in oncology (NCCN guidelines): small cell lung cancer. Version 3.2017-February 23, 2017. Fort Washington, PA: National Comprehensive Cancer Network;2017.
11. De Ruysscher D, Faivre-Finn C, Nestle U, Hurkmans CW, Le Pechoux C, Price A, et al. European Organisation for Research and Treatment of Cancer recommendations for planning and delivery of high-dose, high-precision radiotherapy for lung cancer. J Clin Oncol. 2010; 28:5301–10.


12. Sun Y, Yu XL, Luo W, Lee AW, Wee JT, Lee N, et al. Recommendation for a contouring method and atlas of organs at risk in nasopharyngeal carcinoma patients receiving intensitymodulated radiotherapy. Radiother Oncol. 2014; 110:390–7.


13. Sun Y, Yu XL, Zhang GS, Liu YM, Tao CJ, Guo R, et al. Reduction of clinical target volume in patients with lateralized cancer of the nasopharynx and without contralateral lymph node metastasis receiving intensity-modulated radiotherapy. Head Neck. 2016; 38 Suppl 1:E468–72.


14. Xiao WW, Huang SM, Han F, Wu SX, Lu LX, Lin CG, et al. Local control, survival, and late toxicities of locally advanced nasopharyngeal carcinoma treated by simultaneous modulated accelerated radiotherapy combined with cisplatin concurrent chemotherapy: long-term results of a phase 2 study. Cancer. 2011; 117:1874–83.
15. RTOG Foundation. RTOG 0225 protocol information [Internet]. Rockville, MD: National Cancer Institute;2008. [cited 2018 Jul 2]. Available from: https://www.rtog.org/ClinicalTrials/ProtocolTable/StudyDetails.aspx?study=0225.
16. Kong F, Ying H, Du C, Huang S, Zhou J, Chen J, et al. Patterns of local-regional failure after primary intensity modulated radiotherapy for nasopharyngeal carcinoma. Radiat Oncol. 2014; 9:60.


17. Fletcher GH. Clinical dose response curves of human malignant epithelial tumours. Br J Radiol. 1973; 46:151.
18. Tang LQ, Chen DP, Guo L, Mo HY, Huang Y, Guo SS, et al. Concurrent chemoradiotherapy with nedaplatin versus cisplatin in stage II-IVB nasopharyngeal carcinoma: an openlabel, non-inferiority, randomised phase 3 trial. Lancet Oncol. 2018; 19:461–73.


19. Girinsky T, van der Maazen R, Specht L, Aleman B, Poortmans P, Lievens Y, et al. Involved-node radiotherapy (INRT) in patients with early Hodgkin lymphoma: concepts and guidelines. Radiother Oncol. 2006; 79:270–7.


20. Verhappen MH, Poortmans PM, Raaijmakers E, Raemaekers JM. Reduction of the treated volume to involved node radiation therapy as part of combined modality treatment for early stage aggressive non-Hodgkin's lymphoma. Radiother Oncol. 2013; 109:133–9.


21. Girinsky T, Specht L, Ghalibafian M, Edeline V, Bonniaud G, Van Der Maazen R, et al. The conundrum of Hodgkin lymphoma nodes: to be or not to be included in the involved node radiation fields. The EORTC-GELA lymphoma group guidelines. Radiother Oncol. 2008; 88:202–10.


22. Maraldo MV, Aznar MC, Vogelius IR, Petersen PM, Specht L. Involved node radiation therapy: an effective alternative in early-stage hodgkin lymphoma. Int J Radiat Oncol Biol Phys. 2013; 85:1057–65.


23. De Ruysscher D, Faivre-Finn C, Moeller D, Nestle U, Hurkmans CW, Le Pechoux C, et al. European Organization for Research and Treatment of Cancer (EORTC) recommendations for planning and delivery of high-dose, high precision radiotherapy for lung cancer. Radiother Oncol. 2017; 124:1–10.


24. Yu Z, Luo W, Zhou QC, Zhang QH, Kang DH, Liu MZ. Impact of changing gross tumor volume delineation of intensity-modulated radiotherapy on the dose distribution and clinical treatment outcome after induction chemotherapy for the primary locoregionally advanced nasopharyngeal carcinoma. Ai Zheng. 2009; 28:1132–7.


25. Xue F, Hu C, He X. Induction chemotherapy followed by intensity-modulated radiotherapy with reduced gross tumor volume delineation for stage T3-4 nasopharyngeal carcinoma. Onco Targets Ther. 2017; 10:3329–36.


26. Yang H, Chen X, Lin S, Rong J, Yang M, Wen Q, et al. Treatment outcomes after reduction of the target volume of intensity-modulated radiotherapy following induction chemotherapy in patients with locoregionally advanced nasopharyngeal carcinoma: a prospective, multi-center, randomized clinical trial. Radiother Oncol. 2018; 126:37–42.


Fig. 1.
(A) In MRI before IC, the primary tumor located in the bilateral roof and left wall of nasopharynx, spread to bilateral middle nasal turbinate and bilateral pterygopalatine fossa anteriorly (green arrow), laterally extended to left petrous apex, left carotid canal and posteriorly to left clivus (white arrow). (B) In MRI after IC, the tumor obviously regressed. Tumor enhancement signal could only be seen in anterior roof of nasopharynx, bilateral choanae and bilateral pterygopalatine fossa (green arrow in B), delineated as GTVnx-residual. Signal of skull base bone destruction became unapparent (white arrow in B). Left petrous apex, left carotid canal and left clivus partially recovered in the bone window of computed tomography scan (white arrow in C), delineated as CTVnx1 and prescribed 60 Gy. (D) Red line, purple line, and blue line indicate GTVnxresidual, CTVnx1, and CTV2, respectively. (E) Dose color wash (60 Gy). Yellow line, pink line, green line, light yellow line, blue line, and light blue line (arrow) indicate PGTVnx-residual, GTVnx-pre, PCTVnx1, PCTV2, brain stem, and optic chiasm, respectively. (F) DVH. Orange line, green line, blue line, and red line indicate PGTVnx-residual, PCTVnx1, brain stem, and optic chiasm, respectively. MRI, magnetic resonance imaging; IC, induction chemotherapy; GTVnx, gross tumor volume of nasopharynx; CTV, clinical target volume; PGTV, planning target volume of GTV; PCTV, planning target volume of CTV; DVH, dose volume histogram.
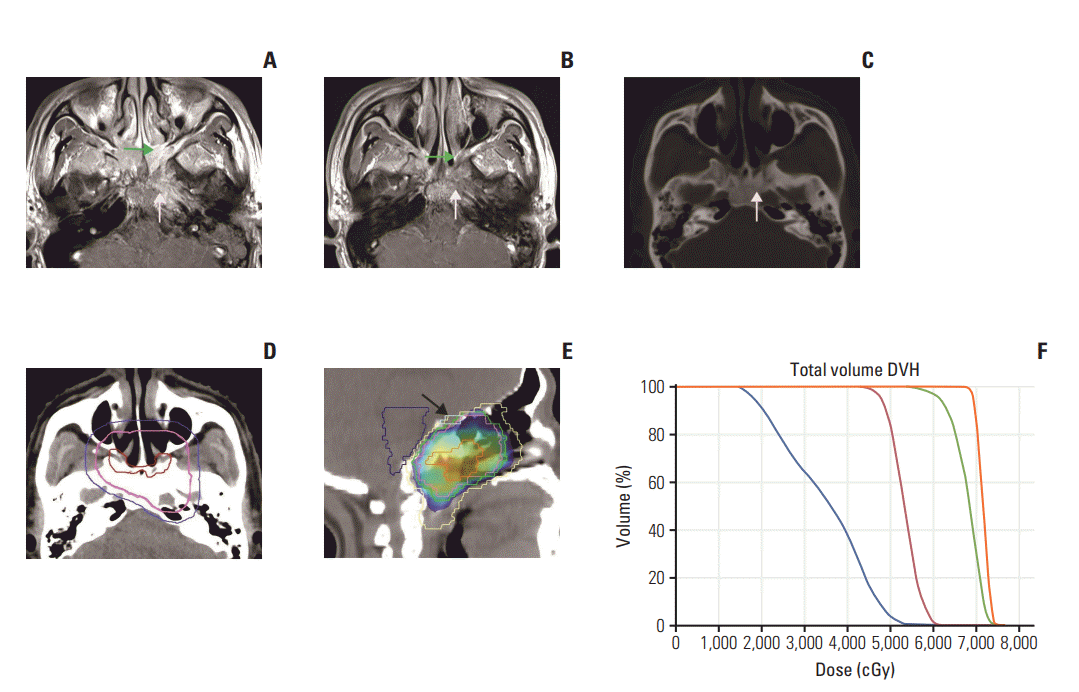
Fig. 2.
(A) In MRI before IC, the tumor invaded ethmoidal cellules and orbital apex, with the right optic nerve suspiciously invaded (white arrow). The patient suffered with inflammation after wadding for nasopharynx bleeding (red arrow in A). (B, C) The tumor volume was reduced and no tumor signal was founded in MRI after IC (white arrow in B) and computed tomography after IC (white arrow in C) in the same slice as A. (D) Therefore, the tumor regressed region was outlined as CTVnx1, prescribed 60 Gy (pink line, CTVnx1; blue line, CTV2 in D). (E) Dose color wash (60 Gy). Orange line and red line indicate PCTVnx1 and optic chiasm, respectively. (F) DVH. Green line, red line, and blue line, indicated PCTVnx1, right optic nerve, and optic chiasm, respectively. MRI, magnetic resonance imaging; IC, induction chemotherapy; CTV, clinical target volume; PCTV, planning target volume of CTV; DVH, dose volume histogram.
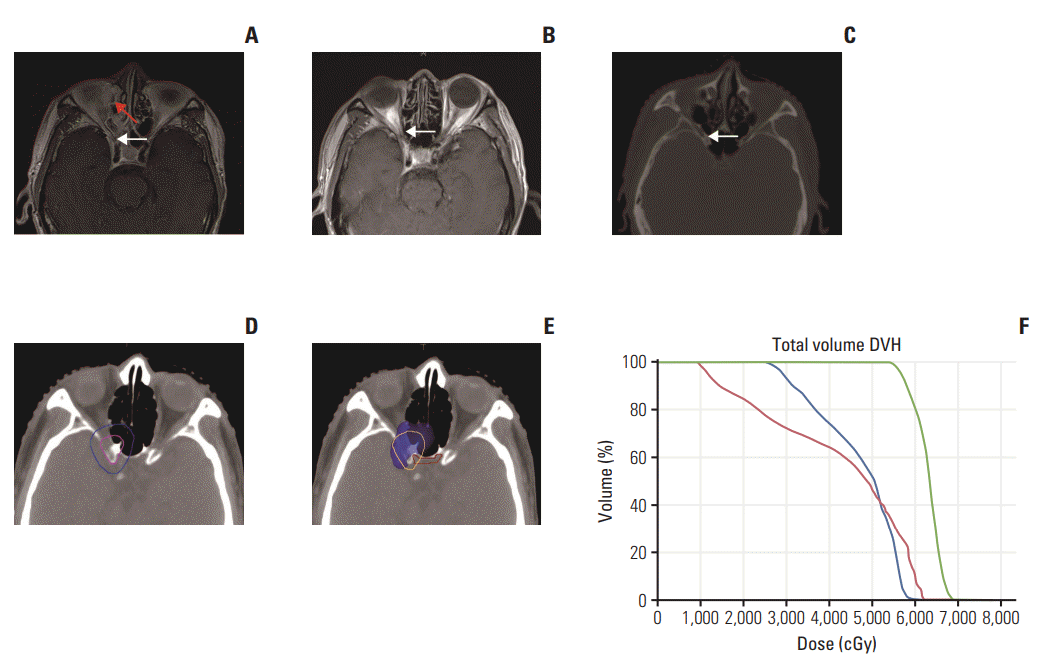
Fig. 3.
(A) Kaplan-Meier estimates of locoregional-free survival (5-year LRFS, 87.7%; 95% confidence interval [CI], 82.6 to 92.7). (B) Kaplan-Meier estimates of distant metastasis-free survival (5-year DMFS, 85.8%; 95% CI, 80.3 to 91.4). (C) KaplanMeier estimates of progression-free survival (5-year PFS, 80.3%; 95% CI, 73.0 to 87.6). (D) Kaplan-Meier estimates of overall survival (5-year OS, 82.2%; 95% CI, 75.9 to 88.5).
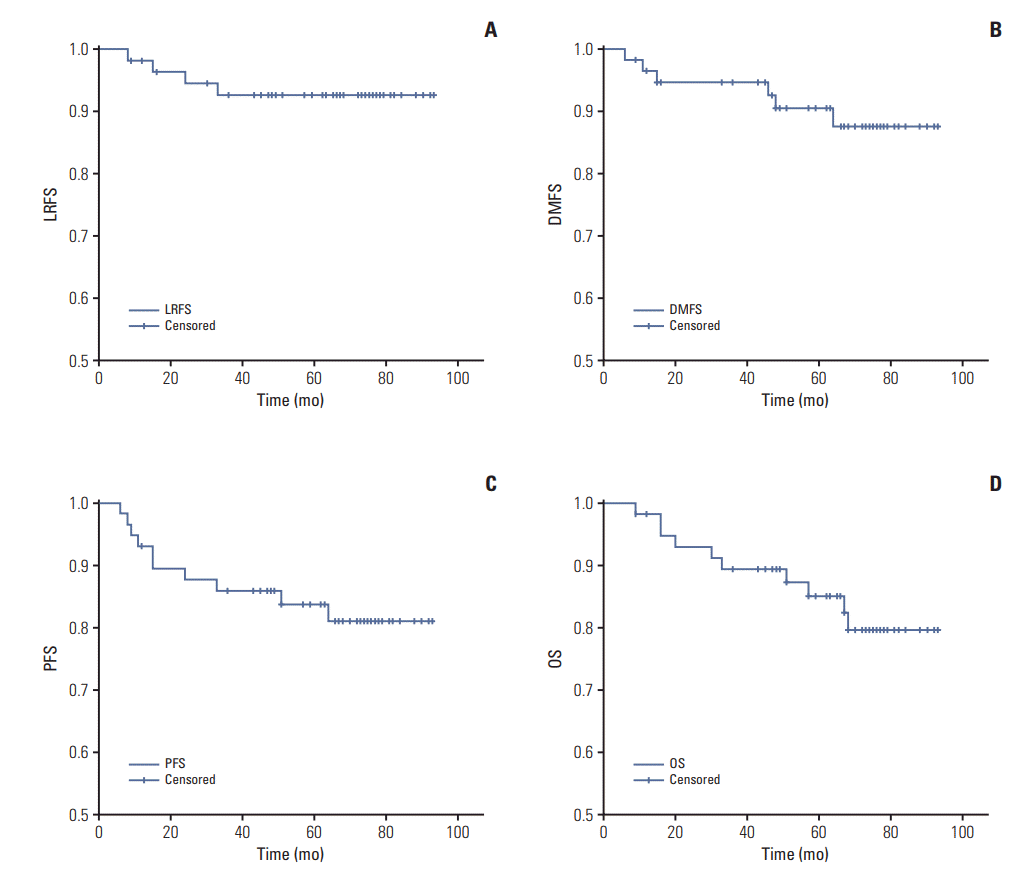
Table 1.
Definitions and prescribed dose of target volumes
Table 2.
Characteristics of the 57 NPC patients
Table 3.
Comparison of tumor volume before and after IC
Target volume | Pre-IC (cm3) | Post-IC (cm3) | p-value | Tumor shrinkage (%) |
---|---|---|---|---|
GTVnx | 63.7±45.6 | 21.8±23.7 | < 0.01 | 66.5 |
GTVnd | 21.7±21.8 | 7.5±9.4 | < 0.01 | 68.0 |
GTV | 85.4±51.0 | 29.3±26.6 | < 0.01 | 67.2 |
Table 4.
Clinical characteristics and patterns of locoregional failure of four patients
AJCC, American Joint Committee on Cancer; IC, induction chemotherapy; CCT, concurrent chemotherapy; GTVnx, gross tumor volume of nasopharynx; GTVnd, gross tumor volume of cervical lymph node; TR, time to relapse; TPF, a combination of taxanes, cisplatin and fluorouracil; Cis, cisplatin; S., superior; NP., nasopharynx; L., left; NC., nasal cavity; LN, lymph node; RPN, retropharyngeal lymph node; R., right.