Abstract
Purpose
The purpose of this study was to identify novel plasma biomarkers for distinguishing nasopharyngeal carcinoma (NPC) patients from healthy individuals who have positive Epstein-Barr virus (EBV) viral capsid antigen (VCA-IgA).
Materials and Methods
One hundred seventy-four plasma cytokines were analyzed by a Cytokine Array in eight healthy individuals with positive EBV VCA-IgA and eight patients with NPC. Real-time polymerase chain reaction, Western blotting, enzyme-linked immunosorbent assay (ELISA), and immunohistochemistry were employed to detect the expression levels of macrophage migration inhibitory factor (MIF) and CC chemokine ligand 3 (CCL3) in NPC cell lines and tumor tissues. Plasma MIF and CCL3 were measured by ELISA in 138 NPC patients, 127 EBV VCA-IgA negative (VN) and 100 EBV VCA-IgA positive healthy donors (VP). Plasma EBV VCA-IgA was determined by immunoenzymatic techniques.
Results
Thirty-four of the 174 cytokines varied significantly between the VP and NPC group. Plasma MIF and CCL3 were significantly elevated in NPC patients compared with VN and VP. Combination of MIF and CCL3 could be used for the differential diagnosis of NPC from VN cohort (area under the curve [AUC], 0.913; sensitivity, 90.00%; specificity, 80.30%), and combination of MIF, CCL3, and VCA-IgA could be used for the differential diagnosis of NPC from VP cohort (AUC, 0.920; sensitivity, 90.00%; specificity, 84.00%), from (VN+VP) cohort (AUC, 0.961; sensitivity, 90.00%; specificity, 92.00%). Overexpressions of MIF and CCL3 were observed in NPC plasma, NPC cell lines and NPC tissues.
Nasopharyngeal carcinoma (NPC) is a malignant neoplasm of the head and neck, which is rare in most countries but common in southern regions of China [1,2]. Epstein-Barr virus (EBV) infection was strongly associated with NPC development [3,4]; hence, the immunoglobulin antibody against the EBV viral capsid antigen (VCA-IgA) and EBV early antigen (EA-IgA) were used as common screening markers for NPC [5]. As a substantial proportion of people in the world have been infected with EBV, the traditional screening biomarker VCA-IgA was not satisfactory for distinguishing NPC patients from high-risk individuals who have positive anti-EBV antibodies [6,7], leading to unnecessary pathological examinations and mental stress for the patients.
The combination of a number of antibodies against EBV antigens, including VCA-IgA, EA-IgA, EBNA1-IgA, Zat-IgA, and Rat-IgG, has been developed to improve the specificity of diagnosis [8,9]. In addition, antibodies against EBV antigens combined with EBV DNA load in plasma were also found to increase the specificity of NPC diagnosis [10,11]. However, the results of antibody tests alone have proven insufficiently accurate to diagnose NPC. Additionally, EBV DNA tests appear to be of limited value for diagnosing NPC patients with early-stage disease and local recurrence [10,11]. All of these problems drive us to search for novel biomarkers for increasing the specificity and positive predictive value (PPV) of NPC diagnosis.
Apart from EBV-related biomarkers, numerous studies have demonstrated that secreted proteins were up-regulated in NPC tumor cells and may be additional biomarkers for NPC diagnosis. Chang et al. [12] found that macrophage inflammatory protein-3α was a novel serum marker for NPC detection. Hsin et al. [13] reported that CXCL9 is a good diagnostic marker for determining NPC. These studies suggested that biomarkers produced by NPC cells combined with EBV-related biomarkers could improve the diagnostic specificity for NPC. Protein microarray technology can detect many components simultaneously, such as cytokines and chemokines [14]. In this study, we used protein microarray technology to identify potential plasma biomarkers for distinguishing NPC patients from healthy individuals with positive EBV antibodies. We identified macrophage migration inhibitory factor (MIF) and CC chemokine ligand 3 (CCL3) as novel markers, which were significantly elevated in the plasma of NPC patients compared to healthy individuals with positive EBV antibodies. MIF is a multi-functional cytokine, which is associated with inflammation and tumorigenesis. Recently, MIF was reported to be highly expressed in a number of tumors, including NPC, colorectal cancer and lung cancer [15-17]. CCL3 is a cytokine belonging to CC subfamily, and is involved in diverse proinflammatory responses [18]. CCL3 was reported up-regulated in numerous tumors, such as chronic myeloid leukemia and renal cell carcinoma [19,20]. Nevertheless, the diagnostic significance of plasma MIF and CCL3 in human NPC has not yet been elucidated. Therefore, this study aimed to investigate the plasma MIF and CCL3 levels in NPC patients and evaluate their diagnostic efficiency compared with the traditional NPC tumor marker VCA-IgA. We also demonstrated that MIF and CCL3 may be complementary to VCA-IgA to improve the specificity of NPC diagnosis.
The immortalized nasopharyngeal epithelial cell lines NPEC1, NPEC2 [21], N5-Tert were cultured in Keratinocyte-SFM (Invitrogen, Carlsbad, CA) media. The NPC cell lines SUNE2, CNE1, S18, S26, 5-8F, and 6-10B were grown in RPMI 1640 (Invitrogen) supplemented with 5% fetal bovine serum. For the collection of supernatant of the cell lines, cells were grown to approximately 80% confluence (approximately 5×105) in 35-mm culture dishes, and then incubated in 2-mL serum-free media for 24 hours. Then, the conditioned media were collected and centrifuged at 800 ×g for 5 minutes to eliminate suspended cells. The collected cellular supernatant was stored at –80°C until use.
The plasma samples from 138 NPC patients (age, 15 to 69 years; median, 45 years; 100 males and 38 females) were collected at the time of diagnosis before any treatment at the Sun Yat-sen University Cancer Center from 2011 to 2013. All nasopharyngeal cancer patients received confirmed diagnoses by pathological examination. TNM stage was established based on the seventh edition of the International Union for Cancer Control/American Joint Committee on Cancer staging system for NPC. The patient characteristics are described in Table 1. The 127 EBV VCA-IgA negative healthy controls (VN) were all volunteers undergoing routine health examinations or individuals presenting with otolaryngological-related, non-neoplastic diseases (age, 17 to 75 years; median, 38 years; 59 males and 68 females) at the Sun Yat-sen University Cancer Center. The 100 EBV VCA-IgA positive healthy control (VP) samples were collected from healthy donors with at the Physical Examination Center of the Sun Yat-Sen University Cancer Center (age, 21 to 88 years; median, 42 years; 43 males and 56 females), and those healthy donors were confirmed have non-neoplastic diseases in the nasopharynx by naso-pharyngeal biopsy during the 5 years of follow-up, subjects with the following conditions were not included, history of inflammatory diseases, fever of unknown. And all these were assessed by clinical history, physical examination, routine laboratory tests. Plasma was extracted after centrifugation at 3,000 rpm for 15 minutes and stored at –80°C until use.
We used 20 formalin-fixed and paraffin-embedded NPC tumor specimens for immunohistochemistry, and these samples were obtained at the Sun Yat-Sen University Cancer Center from January of 2010 to November of 2011.
A Human Cytokine Antibody Array (Series 2000, Ray-Biotech, Norcross, GA) was used to screen plasma biomarkers related to NPC, which analyzed 174 human cytokines related to angiogenesis, immunity and tumor proliferation pathways. Samples were analyzed according to the manufacturer’s instructions. Two replicates per antibody were used to increase the accuracy, and the coefficient of variation (CV) was less than 10%.
The Trizol reagent (Invitrogen) was used to extract total RNA from cell lines according to the manufacturer’s instructions. A reverse transcriptase kit (Invitrogen) was used to synthesize cDNA from 2 μg of the total RNA.
The quantification of target and reference (β-actin) genes were performed on a LightCycler 480 II (Roche, Applied Science, Rotkreuz, Switzerland) using a SYBR green-based assay (Bio-Rad, Hercules, CA). We used oligonucleotide primers for detecting MIF, and CCL3 as follows: MIF, forward 5′-CACAGTGCCCAGACCCTACAGC-3′ and reverse 5′-GCTTGCTGTAGGAGCGGTTCTG-3′; CCL3, forward 5′-GCTCTCTGCAACCAGTTCTCTG-3′ and reverse 5′-TTCTGGACCCACTCCTCACTG-3′; β-actin, forward 5′-GTGAAGGTGACAGCAGT-CGGT-3′ and reverse 5′-AAGTGGGGTGGCTTTTAGGAT-3′. All the experiments were performed in triplicate.
Cellular proteins were separated by sodium dodecyl sulfate polyacrylamide gel electrophoresis and transferred to polyvinylidene difluoride membranes. Then, they are incubated with antibodies to MIF (1:1,000, ab65869, Abcam, Cambridge, MA), CCL3 (1:250, ab65869, Abcam), and α-tubulin (1:3,000, ab9781, Abcam) at 4°C overnight, then treated with an horseradish peroxidase‒conjugated secondary antibody.
Formalin-fixed, paraffin-embedded NPC sections were incubated with rabbit polyclonal anti-MIF antibody (1:250, Abcam) and rabbit polyclonal anti-CCL3 antibody (1:50, Abcam) overnight at 4°C. After washing, a horseradish peroxidase-conjugated anti-rabbit secondary antibody was added and sections were incubated at 37°C for 30 minutes. The tissue sections were then developed with 3-diaminobenzidine tetrahydrochloride for 10 seconds, followed by counterstaining with 10% Mayer’s hematoxylin. The degree of immunostaining was reviewed by two independent observers.
Plasma MIF and CCL3 levels in tested samples were determined using the RayBiotech sandwich enzyme-linked immunosorbent assay (ELISA) kits according to the manufacturer’s instructions (RayBiotech Systems). Briefly, we add 100 μL/well of test plasma or standard into 96-well plates and incubated them for 2.5 hours. Subsequently, 100 μL/well of the biotinylated antibody solution was added and incubated for 1 hour. Next, 100 μL/well of Streptavidin solution was added and incubated for 45 minutes. Finally, 100 μL/well of TMB One-Step Substrate Reagent was added, and the reaction was stopped by addition of stop solution; the absorbance was measured at 450 nm. Each test included a standard control (CV < 10%).
Plasma EBV titers were determined with the IEA method supplied by the Shanghai Institute of Biological Products according to the manufacturer’s protocol [8]. B95 cell lines were prepared for VCA-IgA antibody on glass slides, the test plasma at a dilution of 1:10 was applied, and this was followed by 2-fold serial dilutions. The antibody titer of test plasma was the reciprocal of the highest dilution showing brown within 15% of the cells. The levels of EBV VCA-IgA were determined by titration, with cut-off values set at 1:40.
All statistical analyses were performed using SPSS ver. 20.0 (IBM Corp., Armonk, NY); Mann-Whitney U tests were used to analyze the relationships between the expression of protein and the clinicopathological features, and the MIF and CCL3 levels were expressed as median and 95% confidence interval. Kruskal-Wallis tests were used to analyze the differences of MIF and CCL3 concentration among more than two groups. The efficacy of MIF and CCL3 were evaluated by area under the curve (AUC). The cut-off value for MIF and CCL3 were defined as the value with the maximization of Yuden index. Furthermore, sensitivity, specificity, PPV, and negative predictive value were used to compare the efficiency of diagnosis among MIF, CCL3, and VCA-IgA. All statistical tests were two-sided, and p-value less than 0.05 was considered statistically significant.
Prior to use of these plasma and tissues, informed consent was obtained from each of the participants. All patients provided written informed consent. This experiment was approved by the Institute Research Ethics Committee of the Cancer Center of Sun Yat-Sen University, Guangzhou, China.
The levels of 174 cytokines in plasma samples from eight VP and eight VP NPC patients were detected by Human Cytokine Antibody Array 2000 (S1 Fig.). Thirty-four of the 174 cyto-kines were significantly different between the two groups (p < 0.05) (Fig. 1A). These cytokines were associated with angiogenesis, tumor growth, tumor migration and tumor immunity. We first selected 10 top up-regulated proteins (MIF, SELE, PDGFAB, CCL24, TIMP-2, VEGF-A, CCL1, CCL3, CCL17, and PDGFAA) from the list of Thirty-four potential markers (The fold change and p-value were shown in Fig. 1B). To confirm the array data, the ten protein were tested by two publically available microarray GEO datasets (GSE12452 and GSE53819), MIF and CCL3 were significantly increased in GSE12452 and GSE53819 (Fig. 1C), and the detail data was shown in S2 Fig.
MIF was selected for further validation in 25 VN, 15 VP and 40 patients with NPC. The MIF protein levels was significantly higher in the NPC cohort than VN (p < 0.001) or VP (p < 0.001) (Fig. 2A). Real-time reverse transcriptase-polymerase chain reaction (RT-PCR), ELISA, and Western blotting were employed to detect mRNA and protein levels of MIF in several NPC cell lines (CNE1, SUNE2, S18, S26, 6-10B, and 5-8F) and immortalized nasopharyngeal epithelial cell lines (NEPC1, NEPC2, and N5-tert) respectively. Compared with the immortalized cell lines, most tumor cell lines exhibited both of higher MIF (Fig. 2A-C). Taken together, our data indicates that MIF was frequently up-regulated in NPC.
To further investigate the exact expression state of MIF in vivo, the protein levels of MIF was determined by immunohistochemistry. MIF protein was highly expressed in the tumor cells and detected in 16 of 20 NPC samples (80%). Moreover, the normal nasopharyngeal epithelium samples showed lower or no expression of MIF (a and b in Fig. 2E). The positive staining signals were observed within the cytoplasm of tumor cells. We observed various levels of MIF protein in tumor cells: low (c and d in Fig. 2E), medium (e and f in Fig. 2E) and high (g and h in Fig. 2E).
CCL3 was also selected for further validation in 25 VN, 15 VP, and 40 NPC, the same observations were made for CCL3 plasma (Fig. 3A). And CCL3 was detected in NPC cell lines by RT-PCR (Fig. 3B), ELISA (Fig. 3C), and Western blotting (Fig. 3D), our data indicates that CCL3 was up-regulated in NPC. CCL3 was highly expressed in tumor cells and detected in 18 of 20 NPC patients (90%). And CCL3 was up-regulated in NPC tissues (c-h in Fig. 3E) compared with normal nasopharyngeal epithelium (a and b in Fig. 3E).
The plasma levels of MIF and CCL3 in the VN (n=127), VP (n=100), and patients with NPC (n=138) are presented in Fig. 4A and B. The plasma levels of MIF in patients with NPC were significantly higher than VN (p < 0.001) and VP (p < 0.001), the plasma levels of MIF in VP were significantly higher than VN (p < 0.001), and the mean levels of MIF were 6.08 ng/mL (range, 0.84 to 40.22 ng/mL) in VN, 11.74 ng/mL (range, 1.98 to 62.60 ng/mL) in VP, and 25.04 ng/mL (range, 2.99 to 69.86 ng/mL) in patients with NPC (Fig. 4A). Similarly, CCL3 levels in patients with NPC were significantly higher than VN (p < 0.001) and VP (p < 0.001), the CCL3 levels in VP were significantly higher than VN (p=0.004) (Fig. 4B), and the mean levels of CCL3 were 69.25 pg/mL (range, 14.93 to 335.80 pg/mL) in VN, 97.28 pg/mL (range, 23.09 to 382.10 pg/mL) in VP, and 189.0 pg/mL (range, 90.18 to 503.30 pg/mL) in patients with NPC (Fig. 4B).
The relationship among the plasma levels of MIF, CCL3 and the clinicopathological parameters are presented in Table 1. The levels of MIF had a significant association with sex (p=0.014) and T classification (p=0.012) but not with age, N classification or overall stage. Associations between CCL3 and the clinic characteristics were not significantly, with the exception of overall stage (p=0.001).
We aimed to evaluate the specificity, the appropriate cutoffs for MIF and CCL3 were obtained when the sensitivity achieved 90% (Table 2). In Fig. 5A, we show that the AUC of MIF and CCL3 for distinguishing NPC from the VN cohort were 0.843 (95% CI, 0.797 to 0.888) and 0.874 (95% CI, 0.829 to 0.919), respectively. The AUC was 0.913 (95% CI, 0.878 to 0.947) when combination of MIF and CCL3, and the combination achieved higher specificity (80.30%) compared with MIF (57.50%) and CCL3 (73.20%) (Fig. 5A). The AUC values of plasma MIF, CCL3, and VCA-IgA in discriminating the NPC patients from VP cohort were 0.732 (95% CI, 0.669 to 0.796), 0.824 (95% CI, 0.769 to 0.879), and 0.736 (95% CI, 0.668 to 0.805), respectively. The combination of MIF, CCL3, and VCA-IgA, AUC 0.905 (95% CI, 0.863 to 0.947), exhibited a higher specificity (84%) than MIF (37%), CCL3 (62%), and VCA-IgA (43%) (Fig. 5B). The AUC of plasma MIF, CCL3, VCA-IgA, and combination of the three markers in discriminating the NPC patients from (VN+VP) cohort were 0.782 (95% CI, 0.735 to 0.829), 0.852 (95% CI, 0.814 to 0.889), 0.881 (95% CI, 0.846 to 0.917), and 0.961 (95% CI, 0.942 to 0.980), respectively (Fig. 5C). And the combination achieved a better specificity (90.90%) than MIF (47.30%), CCL3 (68.60%), and VCA-IgA (77.53%). In addition, the PPV of the combination were also higher than that of MIF, CCL3, and VCA-IgA in VN vs. NPC, VP vs. NPC, and (VN+VP) vs. NPC. In conclusion, our findings suggested that the combination of MIF, CCL3, and VCA-IgA could improve the specificity and PPV of NPC diagnosis.
The development of NPC is closely associated with genetic susceptibility, environmental factors and EBV infection [22]. At present, the gold standard of NPC diagnosis depends on a biopsy of the suspected tumor site with the aid of nasopharyngeal endoscopy, followed by histopathologic assessment. However, nasopharyngeal biopsy sampling is invasive and painful. So, detection of antibodies against EBV antigens, such as VCA-IgA, is currently used for serological screening for NPC. These EBV-related markers were proved to be sensitive for detecting incident NPC; however, the specificity and PPV of these markers are considered relatively low [22]. The low specificity and PPV might be due to the large number of subjects in the world that have been infected with EBV, as EBV antibodies may present in circulation for a long time. Driven by the demand for accurate diagnosis of NPC, other non-EBV related biomarkers of NPC are needed to improve the diagnostic specificity.
In this study, we included healthy individuals with positive EBV VCA-IgA as well as NPC patients and screened their plasma samples with a protein array assessing 174 cytokines. Thirty-four potential markers that significantly clustered both groups of patients were identified. Quantitative analysis by ELISA of those proteins in 25 VN, 15 VP, and 40 patients of NPC confirmed that MIF and CCL3 levels in NPC patients were significantly different from those in the VP cohort (p < 0.001) and the VN cohort (p < 0.001). Moreover, we confirmed that MIF and CCL3 were up-regulated at the protein and RNA levels in NPC cell lines and tumor tissues. In addition, MIF and CCL3 expression were observed mostly within the cytoplasm of tumor cells but not in normal nasopharyngeal epithelia.
MIF is a proinflammatory cytokine produced by macrophages and cancer cells [23]. Tumor-derived MIF has been shown to promote the growth of NPC cells though the MIF/CXCL8/CXCR2 axis. Increased expression of MIF in NPC cells also contributes to angiogenesis by inducing upregulation of interleukin-8 expression in an autocrine EBV-independent pathway, and induces cell invasion and metastasis of NPC [24]. Thus, it is associated with poor prognosis of patients with NPC [25]. These studies supported the idea that MIF played important roles in the development of NPC. Although various studies on MIF roles in NPC have been performed, the potential diagnostic value of plasma MIF in NPC has not been reported. Plasma MIF has been investigated as a tumor marker in a number of cancers, such as colorectal cancer, gastric cancer and oral squamous cell carcinoma [16,26]. CCL3 belongs to the cytokines family and are produced by many cells, especially from monocytes, macrophages, T and B lymphocytes, neutrophils, dendritic cells, astrocytes, osteoblasts, eosinophils, basophils, fibroblasts, and natural killer cells [18]. Cancer cells interact directly and indirectly with host inflammatory cells, and this tumor-associated inflammatory response may lead to an alteration in cancer cell biology by up-regulation of cytokines and inflammatory mediators, such as tumor necrosis factor, interleukin-1α/β, interleukin-2, interferon (IFN)-7, IFN-γ, and these cytokines are trigger the production of CCL3 production [27]. Three receptors CCR1, CCR4, and CCR5 were reported for CCL3. Horuk [28] reported that the receptors CCR5 and CXCR4 as important co-receptors for HIV-1 infection on CD4+ target cells. Yuan et al. [29] reported that CCL3 along with its receptor CCR1, through Ca+ ion channels promoted the migration of malignant hepatoma cells. It show us that CCL3 played a significant role in hepatocellular carcinoma invasion and metastasis. CCL3 facilitates the migration of hepatoma by changing the concentration intracellular Ca+, the CCL3-CCR1 axis may play an important role in HCC invasion and metastasis [29]. Our study aimed to explore their utility as a tumor marker in NPC.
To avoid bias and assessment of the potential diagnostic value of MIF and CCL3 in NPC with more accuracy, we increased the number plasma samples. Similar to the preliminary study, the levels of plasma MIF and CCL3 in the NPC cohort were also significantly higher than that of the VP cohort and VN cohort, and the AUC of MIF for diagnosing NPC from VN, VP, and (VN+VP) were 0.843, 0.732, and 0.782, the AUC of CCL3 for diagnosing NPC from VN, VP, and (VN+VP) were 0.874, 0.824, and 0.852. Combination of MIF and CCL3 could be used for the differential diagnosis of NPC from VN cohort (sensitivity 90.00%/specificity 80.30%), combination of MIF, CCL3, and VCA-IgA could be used for diagnosis of NPC from VP cohort (sensitivity 90.00%/specificity 84.00%), from (VN+VP) cohort (sensitivity 90.00%/specificity 92.00%). Moreover, the combination scheme increased the PPV for NPC detecting, suggesting that the combination of MIF, CCL3, and VCA-IgA could differentiate NPC patients from VN, VP, and (VN+VP) individuals more accurately.
In this study, there was no correlation between plasma MIF concentration and patients’ disease characteristics, except for sex (p=0.014) and T classification (p=0.012). Associations between CCL3 and the clinic characteristics were not significantly, with the exception of overall stage (p=0.001). This study is the first to suggest that MIF and CCL3 may represent a potential non-EBV plasma markers for the diagnosis of NPC. Moreover, the results revealed that the combined detection of MIF, CCL3, and VCA-IgA could increase the specificity for the serological screening and diagnosis of NPC. This test might be useful to reassure high-risk family members that they are not at an elevated risk of NPC within the next few years.
In conclusion, the present study measured the protein levels of MIF in combination with the CCL3 chemokine in plasma for the first time. We have found that plasma MIF and CCL3 were significantly elevated in NPC patients and identified plasma MIF and CCL3 as a promising and potential complementary biomarkers to VCA-IgA for more effective detection of NPC. The combination of MIF, CCL3 with the traditional NPC tumor marker VCA-IgA may significantly improve the specificity of detection of NPC. Although further analysis of more patients is needed to verify these results, this inexpensive, rapid and accessible method potentially elevates the diagnostic efficiency of NPC from high-risk individuals who have positive VCA-IgA.
Electronic Supplementary Material
Supplementary materials are available at Cancer Research and Treatment website (https://www.e-crt.org).
ACKNOWLEDGMENTS
This study was supported by National Natural Science Foundation of China (Grant No. 81472008; NO. 81271902).
References
1. Razak AR, Siu LL, Liu FF, Ito E, O'Sullivan B, Chan K. Nasopharyngeal carcinoma: the next challenges. Eur J Cancer. 2010; 46:1967–78.


2. Cao SM, Guo X, Li NW, Xiang YQ, Hong MH, Min HQ. Clinical analysis of 1,142 hospitalized cantonese patients with nasopharyngeal carcinoma. Ai Zheng. 2006; 25:204–8.
3. Chen CJ, Liang KY, Chang YS, Wang YF, Hsieh T, Hsu MM, et al. Multiple risk factors of nasopharyngeal carcinoma: Epstein-Barr virus, malarial infection, cigarette smoking and familial tendency. Anticancer Res. 1990; 10:547–53.
4. Liu JP, Cassar L, Pinto A, Li H. Mechanisms of cell immortalization mediated by EB viral activation of telomerase in nasopharyngeal carcinoma. Cell Res. 2006; 16:809–17.


6. Karray H, Ayadi W, Fki L, Hammami A, Daoud J, Drira MM, et al. Comparison of three different serological techniques for primary diagnosis and monitoring of nasopharyngeal carcinoma in two age groups from Tunisia. J Med Virol. 2005; 75:593–602.


7. Neel HB 3rd, Pearson GR, Taylor WF. Antibodies to Epstein-Barr virus in patients with nasopharyngeal carcinoma and in comparison groups. Ann Otol Rhinol Laryngol. 1984; 93(5 Pt 1):477–82.


8. Yi Z, Yuxi L, Chunren L, Sanwen C, Jihneng W, Jisong Z, et al. Application of an immunoenzymatic method and an immunoautoradiographic method for a mass survey of nasopharyngeal carcinoma. Intervirology. 1980; 13:162–8.


9. Xia C, Zhu K, Zheng G. Expression of EBV antibody EA-IgA, Rta-IgG and VCA-IgA and SA in serum and the implication of combined assay in nasopharyngeal carcinoma diagnosis. Int J Clin Exp Pathol. 2015; 8:16104–10.
10. Tay JK, Chan SH, Lim CM, Siow CH, Goh HL, Loh KS. The role of Epstein-Barr virus DNA load and serology as screening tools for nasopharyngeal carcinoma. Otolaryngol Head Neck Surg. 2016; 155:274–80.


11. Chan KC. Plasma Epstein-Barr virus DNA as a biomarker for nasopharyngeal carcinoma. Chin J Cancer. 2014; 33:598–603.


12. Chang KP, Hao SP, Chang JH, Wu CC, Tsang NM, Lee YS, et al. Macrophage inflammatory protein-3alpha is a novel serum marker for nasopharyngeal carcinoma detection and prediction of treatment outcomes. Clin Cancer Res. 2008; 14:6979–87.
13. Hsin LJ, Kao HK, Chen IH, Tsang NM, Hsu CL, Liu SC, et al. Serum CXCL9 levels are associated with tumor progression and treatment outcome in patients with nasopharyngeal carcinoma. PLoS One. 2013; 8:e80052.


15. Li J, Mo HY, Xiong G, Zhang L, He J, Huang ZF, et al. Tumor microenvironment macrophage inhibitory factor directs the accumulation of interleukin-17-producing tumor-infiltrating lymphocytes and predicts favorable survival in nasopharyngeal carcinoma patients. J Biol Chem. 2012; 287:35484–95.


16. Lee H, Rhee H, Kang HJ, Kim HS, Min BS, Kim NK, et al. Macrophage migration inhibitory factor may be used as an early diagnostic marker in colorectal carcinomas. Am J Clin Pathol. 2008; 129:772–9.


17. Gamez-Pozo A, Sanchez-Navarro I, Calvo E, Agullo-Ortuno MT, Lopez-Vacas R, Diaz E, et al. PTRF/cavin-1 and MIF proteins are identified as non-small cell lung cancer biomarkers by label-free proteomics. PLoS One. 2012; 7:e33752.


18. Menten P, Wuyts A, Van Damme J. Macrophage inflammatory protein-1. Cytokine Growth Factor Rev. 2002; 13:455–81.


19. Blunt MD, Koehrer S, Dobson RC, Larrayoz M, Wilmore S, Hayman A, et al. The dual Syk/JAK inhibitor cerdulatinib antagonizes B-cell receptor and microenvironmental signaling in chronic lymphocytic leukemia. Clin Cancer Res. 2017; 23:2313–24.


20. Wu Y, Li YY, Matsushima K, Baba T, Mukaida N. CCL3-CCR5 axis regulates intratumoral accumulation of leukocytes and fibroblasts and promotes angiogenesis in murine lung metastasis process. J Immunol. 2008; 181:6384–93.


21. Xiong D, Du Y, Wang HB, Zhao B, Zhang H, Li Y, et al. Non-muscle myosin heavy chain IIA mediates Epstein-Barr virus infection of nasopharyngeal epithelial cells. Proc Natl Acad Sci U S A. 2015; 112:11036–41.


22. Tsao SW, Yip YL, Tsang CM, Pang PS, Lau VM, Zhang G, et al. Etiological factors of nasopharyngeal carcinoma. Oral Oncol. 2014; 50:330–8.


23. Bach JP, Rinn B, Meyer B, Dodel R, Bacher M. Role of MIF in inflammation and tumorigenesis. Oncology. 2008; 75:127–33.


24. Liao B, Zhong BL, Li Z, Tian XY, Li Y, Li B. Macrophage migration inhibitory factor contributes angiogenesis by up-regulating IL-8 and correlates with poor prognosis of patients with primary nasopharyngeal carcinoma. J Surg Oncol. 2010; 102:844–51.


25. Meyer-Siegler KL, Leifheit EC, Vera PL. Inhibition of macrophage migration inhibitory factor decreases proliferation and cytokine expression in bladder cancer cells. BMC Cancer. 2004; 4:34.


26. DE Souza MB, Curioni OA, Kanda JL, DE Carvalho MB. Serum and salivary macrophage migration inhibitory factor in patients with oral squamous cell carcinoma. Oncol Lett. 2014; 8:2267–75.


27. Maurer M, von Stebut E. Macrophage inflammatory protein-1. Int J Biochem Cell Biol. 2004; 36:1882–6.


28. Horuk R. Development and evaluation of pharmacological agents targeting chemokine receptors. Methods. 2003; 29:369–75.


29. Yuan Y, Liu J, Liu Z, He Y, Zhang Z, Jiang C, et al. Chemokine CCL3 facilitates the migration of hepatoma cells by changing the concentration intracellular Ca. Hepatol Res. 2010; 40:424–31.
Fig. 1.
Cluster analysis of antibody-based cytokine microarray in eight healthy individuals with positive Epstein-Barr virus viral capsid antigen (S1-S8) and eight patients with nasopharyngeal carcinoma (NPC) (S9-S16). (A) The analysis shows that 34 cytokines divided healthy person from patients with NPC. (B) The fold change of 10 top up-regulated proteins from the list of 34 potential markers. (C) The expression of cytokines in Dodd’s and Qian’s dataset.

Fig. 2.
Expression of macrophage migration inhibitory factor (MIF) in nasopharyngeal carcinoma (NPC) plasma, NPC cell lines, and NPC tumor tissues. (A) Determination by enzyme-linked immunosorbent assay of plasma baseline levels of MIF in 25 viral capsid antigen (VCA-IgA) negative (VN), 15 VCA-IgA positive (VP), and 40 patients of NPC. Inbox bares show median levels for each cytokine of each group of person. The levels of mRNA and protein in the immortalized nasopharyngeal epithelial cell lines (NPEC1, NPEC2, and N5-Tert) and NPC cell lines were determined by real-time polymerase chain reaction (B) and Western blotting (D). Expression level was normalized by β-actin and α-tubulin, respectively. Error bars represent standard deviations (SD) calculated from three parallel experiments. (C) The level of MIF in supernatant was measured by enzyme-linked immunosorbent assay. (E) The normal nasopharyngeal epithelial tissue showed lower or no expression of MIF. Low, medium, and high expression of MIF were showed in the NPC tissues.
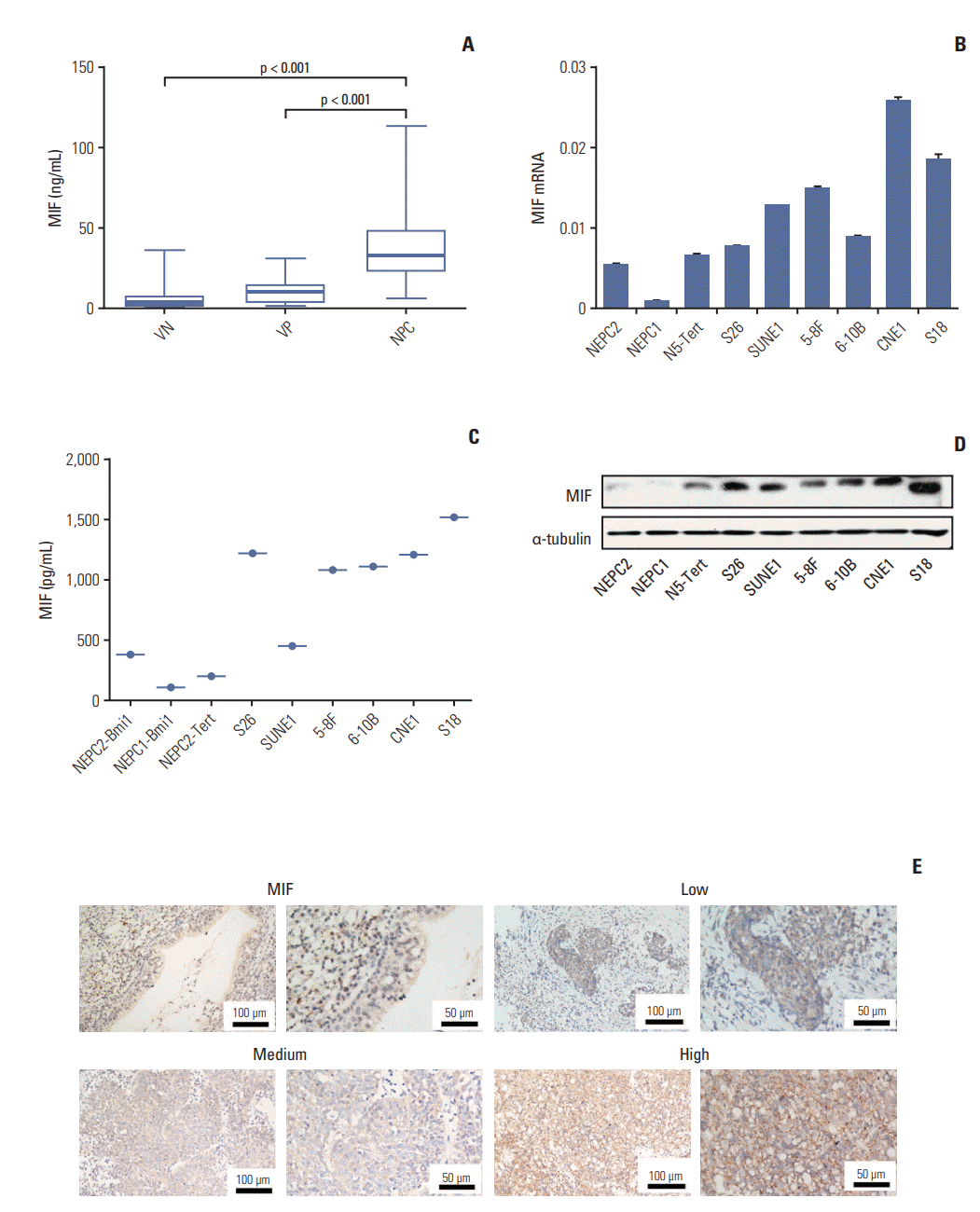
Fig. 3.
Expression of CC chemokine ligand 3 (CCL3) in nasopharyngeal carcinoma (NPC) plasma, NPC cell lines, and NPC tumor tissues. (A) Determination by enzyme-linked immunosorbent assay (ELISA) of plasma baseline levels of CCL3 in 25 viral capsid antigen (VCA-IgA) negative (VN), 15 VCA-IgA positive (VP), and 40 patients of NPC. Inbox bares show median levels for each cytokine of each group of person. The levels of mRNA and protein in the immortalized nasopharyngeal epithelial cell lines (NPEC1, NPEC2, and N5-Tert) and NPC cell lines were determined by real-time polymerase chain reaction (B) and Western blotting (D). Expression level was normalized by β-actin and α-tubulin, respectively. Error bars represent standard deviations calculated from three parallel experiments. (C) The level of CCL3 in supernatant was measured by ELISA. (E) The normal nasopharyngeal epithelial tissue showed lower or no expression of CCL3. Low, medium, and high expression of CCL3 were showed in the NPC tissues.
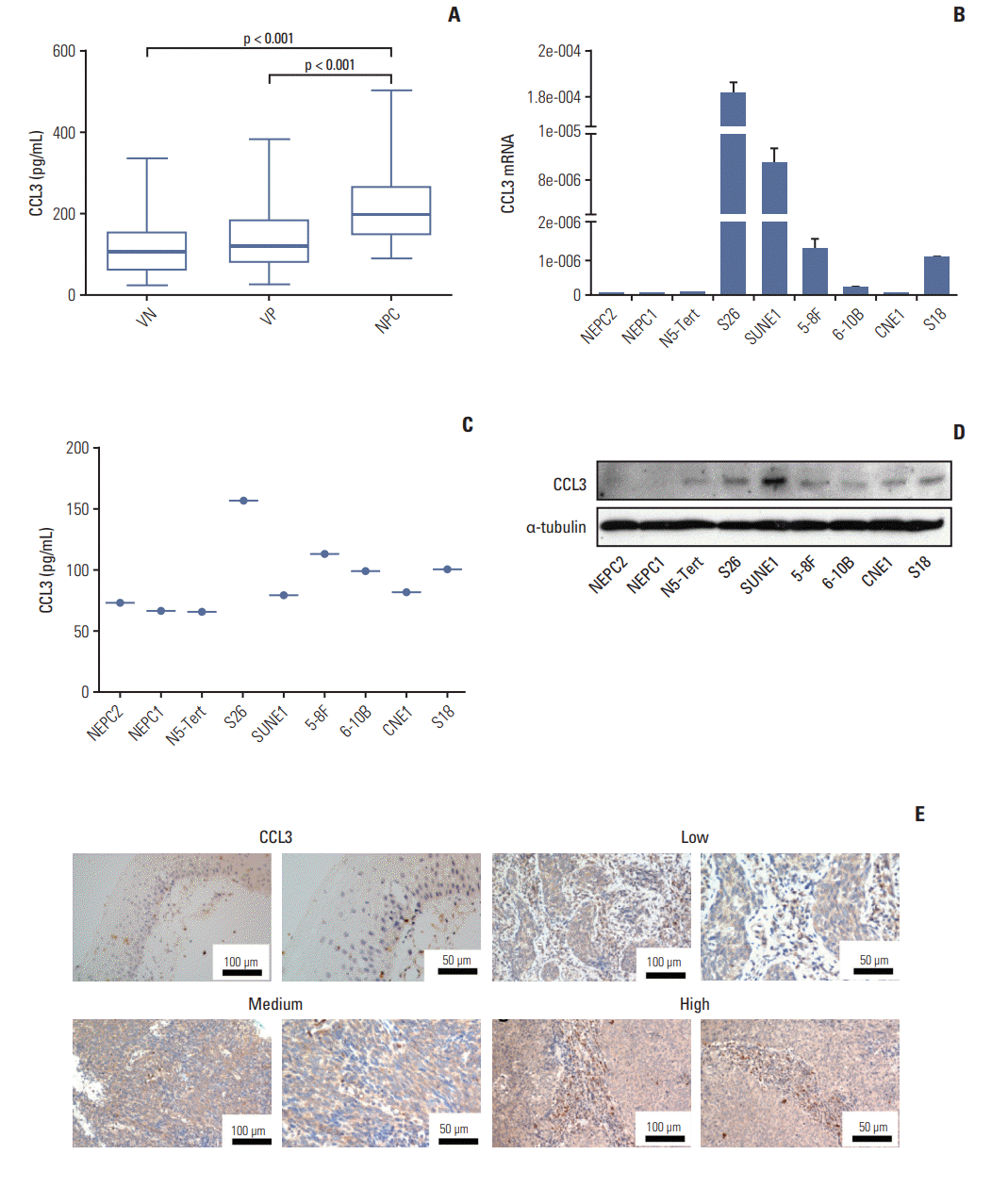
Fig. 4.
The plasma concentration of macrophage migration inhibitory factor (MIF) and CC chemokine ligand 3 (CCL3) in the test cohort. Plasma levels of MIF (A) and CCL3 (B) were measured in viral capsid antigen (VCA-IgA) negative cohort, VCA-IgA positive cohort and NPC patient. Bottom, MIF and CCL3 plasma levels in different groups. p-value was obtained by Kruskal-Wallis test.

Fig. 5.
Diagnosis efficacy of macrophage migration inhibitory factor (MIF), CC chemokine ligand 3 (CCL3), and viral capsid antigen (VCA-IgA) in the diagnosis of nasopharyngeal carcinoma (NPC). (A) Receiver operating characteristic (ROC) curves for diagnosing NPC from VCA-IgA negative (VN) cohort (MIF: area under the curve [AUC], 0.843; CCL3: AUC, 0.874; MIF+CCL3: AUC, 0.913). (B) ROC curves for diagnosing NPC from VCA-IgA positive (VP) cohort (MIF: AUC, 0.732; MIF: AUC, 0.824; VCA-IgA: AUC, 0.736; MIF+CCL3+VCA-IgA: AUC, 0.920). (C) ROC curves for the diagnostic strength to identify NPC from (VN+VP) using MIF, CCL3 and VCA-IgA (MIF: AUC, 0.782; MIF: AUC, 0.852; VCA-IgA: AUC, 0.881; MIF+CCL3+VCA-IgA: AUC, 0.961).
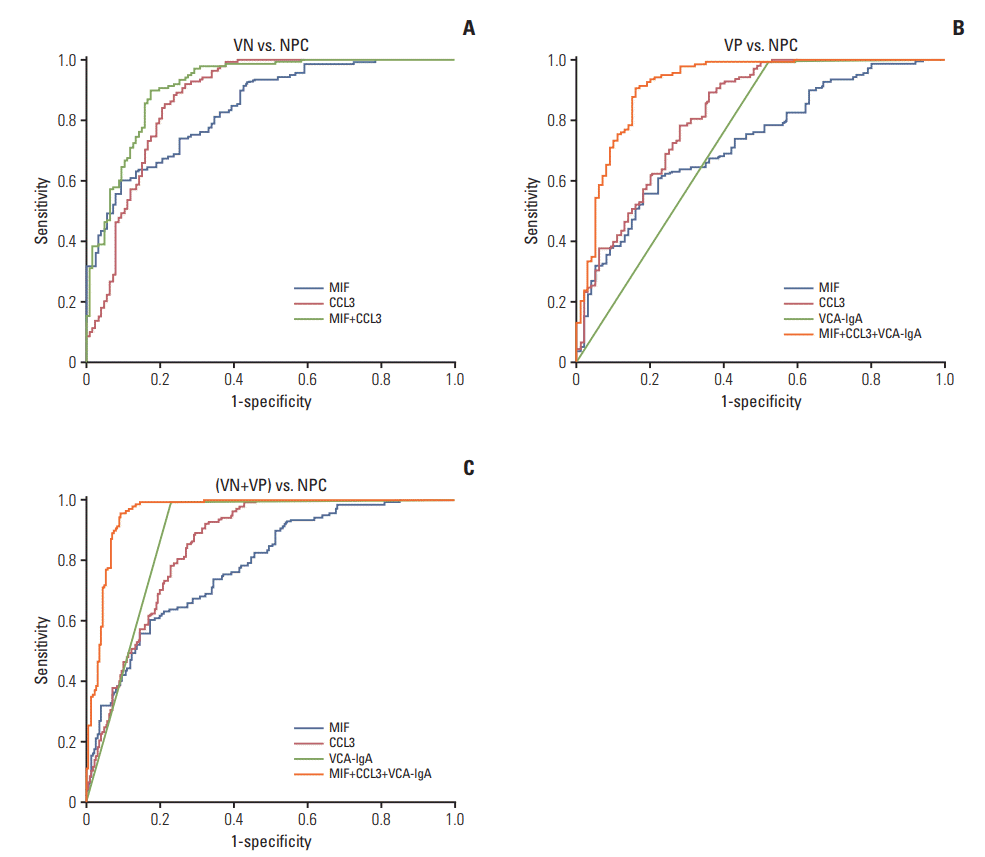
Table 1.
Levels of MIF, CCL3 and clinical characteristics in 138 untreated NPC patients
Charateristic | No. of patients | MIF (ng/mL) | p-valuea) | CCL3 (pg/mL) | p-valuea) |
---|---|---|---|---|---|
Age (yr) | |||||
< 45 | 66 | 22.30 (4.78-62.62) | 0.051 | 193.8 (90.18-489.7) | 0.434 |
≥ 45 | 72 | 29.15 (2.99-69.86) | 184.7 (96.88-503.3) | ||
Sex | |||||
Female | 38 | 15.06 (3.45-69.86) | 0.014 | 186.7 (90.18-369.6) | 0.788 |
Male | 100 | 27.50 (2.99-62.63) | 190.9 (96.88-503.3) | ||
pT status | |||||
pT1-2 | 41 | 20.86 (2.99-62.63) | 0.012 | 178.8 (90.18-375.7) | 0.078 |
pT3-4 | 97 | 30.57 (3.45-69.86) | 196.6 (100.9-503.3) | ||
pN status | |||||
pN 0-1 | 64 | 21.49 (3.45-62.62) | 0.175 | 185.7 (97.56-489.7) | 0.274 |
pN 2-3 | 74 | 29.84 (2.99-69.86) | 198.9 (90.18-503.3) | ||
Overall stage | |||||
Stage I-II | 21 | 16.46 (4.78-62.62) | 0.170 | 156.0 (97.56-263.5) | 0.001 |
Stage III | 62 | 23.87 (2.99-62.63) | 217.0 (96.88-503.5) | ||
Stage IV | 55 | 32.77 (4.47-69.86) | 186.0 (90.18-428.6) |
Table 2.
Diagnostic performance of MIF, CCL3, and VCA-IgA for the differential diagnosis of NPC