Abstract
Purpose
Control of metastatic spread of colorectal cancer (CRC) remains as a major therapeutic challenge. [V4 Q5 ]dDAVP is a vasopressin peptide analog with previously reported anticancer activity against carcinoma tumors. By acting as a selective agonist of arginine vasopressin type 2 membrane receptor (AVPR2) present in endothelial and tumor cells, [V4Q5]dDAVP is able to impair tumor aggressiveness and distant spread. Our aim was to evaluate the potential therapeutic benefits of [V4Q5]dDAVP on highly aggressive CRC disease using experimental models with translational relevance.
Materials and Methods
Murine CT-26 and human Colo-205 AVPR2-expressing CRC cell lines were used to test the preclinical efficacy of [V4Q5]dDAVP, both in vitro and in vivo.
Results
In syngeneic mice surgically implanted with CT-26 cells in the spleen, sustained intravenous treatment with [V4Q5]dDAVP (0.3 µg/kg) dramatically impaired metastatic progression to liver without overt signs of toxicity, and also reduced experimental lung colonization. The compound inhibited in vivo angiogenesis driven by Colo-205 cells in athymic mice, as well as in vitro endothelial cell migration and capillary tube formation. [V4Q5]dDAVP exerted AVPR2-dependent cytostatic activity in vitro (IC50 1.08 µM) and addition to 5-fluorouracil resulted in synergistic antiproliferative effects both in CT-26 and Colo-205 cells.
Conclusion
The present preclinical study establishes for the first time the efficacy of [V4Q5]dDAVP on CRC. These encouraging results suggest that the novel second generation vasopressin analog could be used for the management of aggressive CRC as an adjuvant agent during surgery or to complement standard chemotherapy, limiting tumor angiogenesis and metastasis and thus protecting the patient from CRC recurrence.
With an annual incidence of approximately 1 million cases and a mortality of more than 500,000 patients per year, colorectal cancer (CRC) represents a central public health concern worldwide [1]. In particular, metastatic CRC disease is still associated with a poor prognosis and a high mortality rate, mainly due to hepatic and lung secondary lesions and limitations in the standard therapies. In this clinical setting the overall median survival is 24 months and only 10% of the patients survive more than 5 years. Taken altogether, there is a clear need to develop novel therapeutic strategies to improve the survival of advanced or recurrent CRC patients.
Our group was the first to report the antimetastatic and angiostatic activity of the hemostatic drug desmopressin (1-deamino-8-D-arginine vasopressin; dDAVP) as part of a drug repurposing program in oncology [2,3]. dDAVP is a first generation synthetic derivative of vasopressin (AVP), that acts as a selective agonist on arginine vasopressin type 2 membrane receptor (AVPR2) present in microvascular endothelial cells [4] and several human tumor cell lines, including breast, prostate, lung cancer, and CRC [3,5,6]. After AVPR2 agonistic stimulation in endothelium, dDAVP induces an acute release of von Willebrand factor, an hemostatic [4] and pro-apoptotic glycoprotein known to inhibit metastatic spread of malignant cells [7,8], recruit leukocytes [9], and cause vascular normalization [10]. By acting directly on cancer cells, dDAVP also triggers antiproliferative signalling pathways and favors the formation of angiostatin (ANG), a potent inhibitor of angiogenesis that is generated by cancermediated proteolysis of plasminogen [3]. Due to its safe hemostatic profile and anticancer effects, dDAVP has been evaluated as a perioperative adjunctive therapy in a phase II dose-escalation trial in patients with breast cancer (NCT-01606072) [11] and is currently being evaluated in a phase II trial exploring safety, tolerability, and symptom control in CRC patients with rectal bleeding (NCT01623206). Considering its selective effects on AVPR2-expressing malignant and vascular tissue, dDAVP appears as a promising lead compound for the development of novel peptide analogs with enhanced anticancer efficacy. Lead drug dDAVP was rationally modified after conducting structure-activity studies using alanine-scanning to determine key aminoacidic positions for its biological activity. As a result of this structure-based drug derivatization novel peptidic analog [V4Q5]dDAVP (1-deamino-4-valine-5-glutamine-8-D-arginine vasopressin) was developed. [V4Q5]dDAVP was selected from a wide panel of dDAVP analogs with different sequence and structural modifications, mainly aimed at the N-terminal loop of the molecule, which has a key role in ligand-receptor interaction and antitumor activity [12-14]. Novel compound was evaluated in highly aggressive breast, lung and prostate cancer preclinical models, deploying enhanced cytostatic, antimetastatic and angiostatic effects in comparison to parental peptide dDAVP [5,15,16]. Interestingly, [V4Q5]dDAVP direct cytostatic effects on tumor cells were associated to AVPR2 specific signaling pathways, G0/G1 phase cell cycle arrest and activation of adenylate cyclase/cAMP/protein kinase A (PKA) axis. Acute toxicology studies were also conducted in different animal models, suggesting that intravenous administration of [V4Q5]dDAVP is safe at doses at least 300-fold above than required for antiangiogenic/antimetastatic effects [15].
Monstein et al. [17] presented a complete characterization of vasopressin receptor expression in the human gastrointestinal tract and surrounding tissues. They demonstrated that AVPR2 is widely expressed in normal and malignant biopsy samples from colon, rectum, ileum, duodenum, stomach, esophagus and gallbladder. Additionally, 7 years ago our group reported for the first time AVPR2 expression in human Colo-205 and murine CT-26 CRC cell lines [6].
Unfortunately, as occurs in many types of cancers, CRC patients are diagnosed once the disease is at an advanced stage. In this clinical scenario. therapy includes combined administration of several cytotoxic agents such as 5-fluorouracil (5-FU) in addition to surgery or radiotherapy [18]. Despite the significant improvement in CRC management, the efficacy of current treatment schemes is reduced due to lack of selectivity, high associated toxicity and development of resistance mechanisms. This demands the development of alternative therapeutic strategies with novel molecular targets involved in CRC progression.
The current study aimed at testing the use of vasopressin analog [V4Q5]dDAVP on highly aggressive CRC experimental models, in order to evaluate its preclinical anticancer efficacy with particular interest in key pathological processes related to disease progression such as metastatic spread to liver and lung, cellular growth and angiogenesis induction. Potential therapeutic benefits of [V4Q5]dDAVP addition to standard-of-care chemotherapy were also explored in vitro.
[V4Q5]dDAVP was obtained from Romikin S.A. (member of CHEMO group, Argentina) following GMP standards or purchased from American Peptide Company Inc. (member of BACHEM group, Torrence, CA). Product and lot number: 369184 and 1507008T, respectively). Peptide purity was 98%-99% as assesed by high-performance liquid chromatography-mass spectrometry. Peptide sequence is as follows: Mpr-Tyr-Phe-Val-Gln-Cys-Pro-DArg-Gly-NH2 (disulfide bridge between Mpr-Cys). In order to achieve work concentrations, [V4Q5]dDAVP was diluted in physiological saline solution. The chemotherapeutic drug 5-FU was provided by Bristol-Myers Squibb (Princeton, NJ).
CT-26 (ATCC CRL-2638) and Colo-205 (ATCC CCL-222) cancer cell lines were obtained from the American Type Culture Collection. CT-26 is a highly aggressive and metastatic KRAS-mutated murine colon carcinoma [19]. Colo-205 is a human colon adenocarcinoma cell line bearing the BRAF (V600E) mutation [20]. Colo-205 cells grow as mixed, adherent and suspension cultures. Tumor cell lines were grown in RPMI (Gibco, Rockville, MD) plus 10% fetal bovine serum (FBS), 2 mM glutamine and 80 μg/mL gentamycin, at 37°C in a humidified atmosphere of 5% CO2. HMVEC-L human microvascular endothelial cell line was obtained from Cascade Biologics and cultured in gelatin coated plates using endothelial cell medium with specific growth factors (EGM-2 MV Bullet Kit, Lonza, Milano, Italy). All cells were harvested using a tripsin/EDTA solution (Gibco) diluted in phosphate buffered saline.
Inbred immunocompetent female BALB/c AnN and outbred athymic female N:NIH(S)-nu mice aged 8 weeks with a weight of aproximately 30 and 22 g, respectively, were purchased from UNLP (Universidad Nacional de La Plata, Buenos Aires, Argentina), and, after randomization, housed at five mice per cage in our animal house facility at the National University of Quilmes. Food and water were provided ad libitum and general health status of the animals was monitored daily.
To evaluate the impact of [V4Q5]dDAVP sustained treatment on colorectal tumor progression and metastatic spread to liver a mixture containing 1.5×104 CT-26 CRC cells and serum-free growth medium was surgically injected in the spleen of syngeneic BALB/c mice. For splenic inoculation, mice were anesthetized, followed by left upper quadrant laparotomy and splenic exteriorization. Animals were monitored for water consumption, weight and general behavioral status, and sacrificed by cervical dislocation 21 days after CT-26 inoculation. [V4Q5]dDAVP peptide was injected at a dose of 0.3 μg/kg intravenously, being a clinically relevant dose with widely acknowledged antitumor properties in mice [15], 30 minutes before tumor cell injection, and continued on a three times a week basis until the end of the protocol. This specific treatment schedule was chosen given that peptides such as [V4Q5]dDAVP, or its parental compound dDAVP, can induce tachyphylaxis with daily applications. To investigate the presence of metastases, liver, intestines, and spleen were removed, weighted and fixed in buffered formalin after animals were sacrificed. Surface hepatic nodules were counted under a dissecting microscope, and lesions were further confirmed by histopathology.
To evaluate [V4Q5]dDAVP effect on blood-borne experimental metastases, 2×105 CT-26 cells in serum-free growth medium were injected into the tail vein of mice [15]. On day 21, colonized lungs were excised, weighted, fixed in Bouin´s solution and photographed. Pulmonary lesions and differences between experimental groups were further confirmed by histopathology. [V4Q5]dDAVP was administered using two 0.3 μg/kg intravenous doses, the first at time zero and the second 24 hours after tumor cell injection.
To evaluate the effects on CRC-induced angiogenesis, a modified Matrigel plug assay was conducted. A mixture containing 500 μL of Matrigel, heparin (50 U/mL) and 2.5×106 Colo-205 tumor cells in 100 μL serum-free growth medium was injected subcutaneously into athymic mice. Treatment consisted of three weekly intravenous doses of [V4Q5]dDAVP of 0.3 μg/kg. Animals were sacrificed 14 days after cell injection. Plugs were recovered and scanned at high resolution. The extent of vascularization was assessed by the amount of hemoglobin detected in the implants using the Drabkin method (Sigma-Aldrich, St. Louis, MO). The mean optical density of plugs from control group was taken as 1 (relative hemoglobin content).
[V4Q5]dDAVP capacity to modulate microvascular endothelial cell morphogenesis and migration was evaluated. In vitro endothelial cell morphogenesis assay was performed using Matrigel-coated 24-well plates (BD Biosciences, San Jose, CA) [3]. Briefly, 1×105 AVPR2-positive HMVEC-L cells were incubated with [V4Q5]dDAVP (1 μM) and, after allowing capillary tube formation for 24 hours, randomly chosen fields were photographed at ×100 magnification and quantification was conducted. The number of capillary-like tubes formed in control cultures was taken as 100%. Direct effect of [V4Q5]dDAVP on endothelial cell migration was evaluated using the Transwell migration assay using a modified Boyden chamber inserted with polyethylene terephthalate filter membrane containing 8-μm pores in 24-well plates (Greiner Bio-One, Monroe, NC). 1×105 HMVEC-L cells suspended in 300 μL of serum-free medium were seeded onto the upper compartment of the Transwell chamber. The lower chamber was filled with medium containing FBS as chemoattractant and [V4Q5]dDAVP using a final concentration of 1 μM. After incubation for 24 hours the medium in the upper chamber was removed and the filters were fixed with crystal violet 0.5% and MeOH 20% for 10 minutes. The cells remaining on the upper surface of the filter membrane were then completely removed by wiping with a cotton swab. The migrated cells were counted from five randomly selected fields (×200 magnification) under an inverted microscope.
Total RNA from 1×106 Colo-205, MCF-7, or H125 cells was purified with Trizol. RNA was reverse transcribed with SuperScript III first-Strand (Thermo Fisher Scientific Inc., Waltham, MA) according to the manufacturer's protocol. The following specific forward and reverse primers were used: for AVPR2, 5′-CTGGCCAAGGACACTTCATC-3′ and 5′-GAAGGCAGCTGAGCTTC-3′; for hypoxanthine phosphoribosyl transferase 1 (HPRT1), 5´-AACGTCTTGCTCGAGATGTG-3´ and 5′-GCTTTGATGTAATCCAGCAGG-3´. Quantitative real time polymerase chain reaction was performed using SYBR Green PCR Master Mix (Thermo Fisher Scientific Inc.) and StepOne Real-Time PCR System (Applied Biosystems, Foster City, CA). The following thermal cycling conditions were used: 48°C for 30 minutes, 95°C for 10 minutes, 40 cycles of 95°C for 15 seconds followed by 60°C for 60 seconds. Each sample was analyzed in triplicate and mean cycle threshold values (Ct) were used for further analysis. Ct values were normalized for HPRT1 expression levels and normalized to control samples. Relative quantification values were calculated as 2(-ΔΔCt).
In vitro experiments were performed using nanomolar and low micromolar concentrations of the peptide, a range consistent with the in vivo dosage, as reported [5,15,16]. Colony forming ability was examined by the 2D clonogenic assay for adherent murine CRC cells. CT-26 cells were grown in complete medium with [V4Q5]dDAVP (0.1-1.5 μM) and after 72 hours, medium with tested compound was renewed. Seven days after cell seeding, clonogenic cultures were fixed and stained with crystal violet, and colonies of more than 50 tumor cells were counted.
Antiproliferative effect against rapidly growing tumor cells was measured using the MTT and MTS assays (Sigma-Aldrich) for high density adherent or non adherent cell cultures, respectively. Briefly, cells were plated in 96-well flat bottom plates at a density of 2.5×103 per 200 μL in complete RPMI medium, allowed to attach overnight, and then treated for 72 hours with tested compounds. Following previously published protocols, in order to calculate the 50% inhibitory concentrations (IC50) colorectal cells were treated using micromolar concentrations of 5-FU (0.1-5 or 1-500 μM for CT-26 or Colo-205 cells, respectively) [6]. Combination studies using [V4Q5]dDAVP (1 μM) plus sub-IC50 of 5-FU (250-500 nM or 2.5-5 μM, depending on cell type), were also conducted. MTT or MTS reagent was added to each well and the plate incubated for 4 hours. In the case of MTT, after solubilisation of formazan crystals by using dimethyl sulfoxide the absorbance of each well was measured at 570 nm. In the case of MTS, direct measurement of absorbance at 490 nm was conducted. The optical density of untreated control cells was taken as 100% viability.
All statistical analyses were performed using the PRISM 6 GraphPad Software Inc. (La Jolla, CA) or Compusyn software (ComboSyn Inc., Paramus, NJ). To compare differences between two experimental groups two-tailed Mann Whitney or t tests were used for non-parametric or normal distribution of data, repectively. In case of more than two experimental groups, ANOVA analysis with Tukey's multiple comparisons post-test was used. Kruskal-Wallis analysis with Dunn's multiple comparisons post-test was used in case of non-parametric distribution of data. In tumor progression protocols, growth rates represent the slopes of the linear regressions of the tumor volumes over time. In combinational studies sinergy was determined when combinational index (CI) was < 1. Differences were considered statistically significant at a level of p < 0.05. Data were presented as median with range (non parametric) or mean±standard deviation or standard error of mean (parametric).
Liver metastases is the leading direct cause of cancerrelated deaths in CRC [21,22]. Taking this clinical concern into account we first evaluated the impact of [V4Q5]dDAVP treatment on metastatic spread to liver in animals surgically implanted in the spleen with CT-26 cells (Fig. 1). All animals from both experimental groups displayed visible liver metastases, with a maximum of 57 macroscopic nodules per mouse as observed in the control group (Fig. 1B). In this experimental setting, perioperative plus sustained intravenous administration of [V4Q5]dDAVP caused a remarkable decrease in liver metastatic burden. Total liver metastases in [V4Q5]dDAVP-treated animals was three times lower than control group with 9 (2-23) versus 31.5 (21-57) nodules in [V4Q5]dDAVP and saline vehicle groups, respectively (median with range) (Fig. 1C, left). Moreover, the number of macrometastatic lesions (metastatic nodules larger than 1 mm) drop from 20 (14-33), as found in the control group, to 2.5 (1-12) after [V4Q5]dDAVP treatment (Fig. 1C, right). Additionally, as shown in Fig. 1A, some degree of reduction in primary splenic disease was also observed; however, no statistical significance was achieved after analyzing spleen weights (371 mg [146-871] vs. 200 mg [103-436] in control and [V4Q5]dDAVP groups, respectively). Values expressed as median and range (p=0.17). Differences in number and extension of metastatic lesions can be clearly observed in representative sections of colonized livers as depicted in Fig. 2. Administration of [V4Q5]dDAVP in mice was not associated with overt toxic effects and no significant differences in mouse body weight were found when compared to the control group throughout the experiment (S1 Fig.). These results are in concordance with previously reported toxicological and safety studies, where, after intravenous doses of up to 100 μg/kg of [V4Q5]dDAVP, no altered biochemical and hematological parameters or signs of toxicity were observed [15].
Given that liver and lungs are the two most frequent sites of metastatic spread of CRC [21], we further tested the effects of [V4Q5]dDAVP administration on experimental metastatic spread to lung of CRC cells in BALB/c mice (Fig. 3). After analyzing colonized lung weights as a gross measure of metastatic tumor burden, [V4Q5]dDAVP intravenous treatment given before and after tumor cell injection inhibited pulmonary metastasis formation by 36% in comparison to saline vehicle-treated animals (Fig. 3B). Histological assessment of metastatic burden in lungs was also conducted. Multiple subpleural and intrapulmonary metastatic nodules were visualized in controls (Fig. 3C). In contrast, lungs from animals receiving [V4Q5]dDAVP treatment showed a few peripheral nodules of small sizes, with a well-conserved pulmonary parenchyma (Fig. 3C).
It is well known that high vascular density and overexpression of pro-angiogenic biomarkers in CRC correlate with disease progression [23]. In this setting we evaluated the effect of [V4Q5]dDAVP administration on in vivo angiogenesis (Fig. 4) triggered by human AVPR2-expressing CRC cells (S2 Fig.) in nude mice. As observed in Fig. 4, 2 weeks of intravenous treatment using [V4Q5]dDAVP was capable of reducing Colo-205 cell-induced angiogenic response by nearly 60% in contrast with saline vehicle-treated animals (Fig. 4A and B).
Additionally, direct modulation of AVPR2-expressing microvascular cell migration and morphogenesis was assessed (Fig. 4C and D). After overnight incubation with [V4Q5]dDAVP endothelial cell chemotaxis was reduced by 26% versus control. [V4Q5]dDAVP was also capable of modulating capillary-like tube formation by microvascular cells, a key early event in novel vessel development, decreasing morphogenic capacity by 33% (Fig. 4C and D).
Growth-modulating activity was evaluated in low and high density CRC cell cultures exposed to [V4Q5]dDAVP (Fig. 5). Long-term in vitro treatment using [V4Q5]dDAVP reduced clonogenic growth of highly aggressive CT-26 CRC cells, obtaining an IC50 of 1.08 μM (Fig. 5A). Impairment of tumor cell colony formation was completely reverted after pre-incubation with the selective AVPR2 antagonist tolvaptan (Fig. 5B), hinting a AVPR2-dependency of [V4Q5]dDAVP in vitro activity. 5-FU is a standard chemotherapy agent widely used in the treatment of malignancies in the gastrointestinal tract. After IC50 determination for 5-FU in both CRC cell lines (Fig. 5C), we explored potential combinational benefits on exponentially growing murine CT-26 and human Colo-205 cells by adding [V4Q5]dDAVP to sub-IC50 concentrations of 5-FU (Fig. 5D). Combined in vitro treatments resulted in a synergistic inhibition (CI < 1) (S3 and S4 Tables) of CRC cell growth in comparison to cytotoxic or [V4Q5]dDAVP single agent therapies, especially after combining [V4Q5]dDAVP (1 μM) with 0.5 μM (Fig. 5D, left) or 5 μM (Fig. 5D, right) concentrations of 5-FU in the CT-26 or Colo-205 cell lines, respectively.
As previously described, CRC is a relevant public health issue, with approximately 50% of patients eventually developing metastatic disease. To our knowledge this is the first preclinical report of the antitumor effects of the synthetic peptide [V4Q5]dDAVP using highly aggressive and metastatic CRC experimental models. In CRC 80% of all metastases occur in the liver [21,22]. Given the highly relevant biological functions of the hepatic tissue and their poor prognosis and elevated incidence, liver lesions associated to CRC attract particular interest of both clinicians and researchers. In this work sustained treatment using clinically relevant intravenous doses of [V4Q5]dDAVP was capable of impairing spread and growth of CRC cells in the liver. Moreover, [V4Q5]dDAVP effects on lung colonization by CRC cells were also evaluated. Lungs are the second most common site of seeding of CRC, with pulmonary metastasis occurring in approximately 10% of patients who undergo resection of the primary tumor [21]. In this setting, [V4Q5]dDAVP has also great clinical applicability given the fact that it was capable of reducing pulmonary metastatic burden in an experimental model of massive CRC cell seeding in the lung.
Numerous studies in CRC patients associate high intratumoral vascular density and vascular endothelial growth factor (VEGF) expression in the primary tumor with an increase in disease recurrence and metastasis development [23]. In the present study we assessed the effect of [V4Q5]dDAVP treatment on CRC-associated angiogenesis. After 2 weeks of sustained intravenous treatment using [V4Q5]dDAVP we were able to reduce by 60% the early vascular development induced by Colo-205 cells, a highly aggressive and chemorefractory human BRAFV600E positive CRC model. As previously reported by our group, [V4Q5]dDAVP was able to impair early angiogenesis induction and reduce vascular density in hormone-independent breast cancer tumors [15]. [V4Q5]dDAVP, as well as its parental peptide dDAVP, seems to modulate tumor angiogenesis by inducing the formation of ANG, a potent angiogenesis inhibitor that is generated by cancer-mediated proteolysis of plasminogen [3,24]. Additionally, after analyzing main angiogenic modulators using mRNA microarrays in AVPR2 expressing breast cancer cells, dDAVP was also able to modulate key proangiogenic proteins, including a dramatic reduction of VEGF-B and plateletderived growth factor, and a complete inhibition of HIF-1A expression. These results suggest that [V4Q5]dDAVP may modulate angiogenesis by different mechanisms including increased production of endogenous angiostatic molecules and reduction of the expression of different promoters of endothelial growth. However, the specific mechanisms responsible for [V4Q5]dDAVP antiangiogenic effects remain to be elucidated.
Despite surgery being the mainstay of treatment of CRC, recent evidence suggests that surgery may also promote metastatic relapse through different deleterious mechanisms, including, but not limited to, tumor cell shedding, immunosuppression, increased production of growth factors, and proangiogenic signaling [25,26]. After analyzing its activity on CRC metastatic spread and growth, as well as its angiostatic effect, our data suggest that [V4Q5]dDAVP may be potentially used to minimize spread or survival of residual malignant cells perioperatively and during the early postoperative period in surgical procedures of colon and other gastrointestinal tumors in order to protect the CRC patient from local or distant recurrence.
Direct effect of [V4Q5]dDAVP on clonogenic or high density CRC cell cultures was also evaluated. [V4Q5]dDAVP concentrations of 1 μM or higher were able to inhibit tumor cell colony formation, and such cytostatic effects were completely reverted by AVPR2 chemical blockade using specific antagonist tolvaptan. In line with these findings, complete reversal of in vitro [V4Q5]dDAVP activity after AVPR2 chemical blockade was also reported by our group in highly aggressive breast cancer cells [15]. Moreover, we recently showed that after siRNA-mediated knockdown of AVPR2 expression in human lung carcinoma cells, [V4Q5]dDAVP antiproliferative effects were significantly attenuated, confirming AVPR2-dependency of [V4Q5]dDAVP cytostatic action in malignant cells [5,27]. In this experimental setting, the development of a CRC cell model with AVPR2 gene knockout or knockdown is highly interesting, and should be pursued with the aim of corroborating previously described findings. We previously demonstrated that in vitro antiproliferative activity of [V4Q5]dDAVP was associated with partial cell cycle arrest, intracellular cAMP elevation and PKA activation. Adenylate cyclase/cAMP/PKA axis has been correlated in many studies to cancer cell growth inhibition and antiproliferative signaling events. Increases in cAMP intracellular levels and PKA activation using cAMP analogs or cAMP elevating agents such as forskolin, can induce growth arrest in CRC and other tumor cell lines, through different mechanisms including PKA-dependent inhibition of ERK activation or p38 mitogen-activated protein kinase–dependent induction of apoptosis [28,29]. Nevertheless, thorough studies focusing on intracellular signaling pathways triggered by [V4Q5]dDAVP are necessary to further understand the implications of vasopressin analogs and their receptors on CRC biology.
Given that that therapeutic effects of cytotoxic agents in CRC are often limited by adverse effects due to low selectivity, complex dosing and high toxicity, potential benefits of combining [V4Q5]dDAVP with chemotherapy were explored in the present study. In this experimental setting [V4Q5]dDAVP plus 5-FU elicited synergistic cytostatic activity on rapidly growing CRC cells. Despite it has been previously reported that other cytostatic and proapoptotic G proteincoupled receptor ligands can enhance the antiproliferative activity of 5-FU [30], we are unable to specifically determine what kind of cross talk between AVPR2 agonists and 5-FU accounts for the described synergistic effects. In this regard, proper chemotherapy regimens for CRC in combination with [V4Q5]dDAVP remain to be evaluated in preclinical models with translational relevance.
Taking into account its capacity to modulate tumor cell behavior, metastatic spread and tumor-associated angiogenesis, selective vasopressin analogs like the novel compound [V4Q5]dDAVP are interesting candidates for further development as adjuvant agents for CRC treatment in combination to standard chemotherapy or used in a perioperative setting.
Electronic Supplementary Material
Supplementary materials are available at Cancer Research and Treatment website (http://www.e-crt.org).
ACKNOWLEDGMENTS
This work was supported by the National Institute of Cancer (grant No. INC2016/2018 to DFA), the National Agency for the Promotion of Science and Technology (ANPCYT, Argentina, earlycareer grant No. PICT2016/2309 to JG) and Chemo-Romikin S.A. JG, DEG, GVR and DFA are members of the National Research Council (CONICET, Argentina). MP is a postdoctoral research fellow from CONICET and VIS is a postdoctoral research fellow from ANPCYT. NTS is a doctoral research fellow from CONICET. The authors would also like to gratefully acknowledge the work of Dr. Nancy B. Iannucci who passed away recently and the generous assistance of Dr. Alejandra Scursoni in histopathological assessment.
References
1. Ferlay J, Soerjomataram I, Dikshit R, Eser S, Mathers C, Rebelo M, et al. Cancer incidence and mortality worldwide: sources, methods and major patterns in GLOBOCAN 2012. Int J Cancer. 2015; 136:E359–86.


2. Alonso DF, Skilton G, Farias EF, Bal de Kier Joffe E, Gomez DE. Antimetastatic effect of desmopressin in a mouse mammary tumor model. Breast Cancer Res Treat. 1999; 57:271–5.


3. Ripoll GV, Garona J, Pifano M, Farina HG, Gomez DE, Alonso DF. Reduction of tumor angiogenesis induced by desmopressin in a breast cancer model. Breast Cancer Res Treat. 2013; 142:9–18.


4. Kaufmann JE, Oksche A, Wollheim CB, Gunther G, Rosenthal W, Vischer UM. Vasopressin-induced von Willebrand factor secretion from endothelial cells involves V2 receptors and cAMP. J Clin Invest. 2000; 106:107–16.


5. Pifano M, Garona J, Capobianco CS, Gonzalez N, Alonso DF, Ripoll GV. Peptide agonists of vasopressin V2 receptor reduce expression of neuroendocrine markers and tumor growth in human lung and prostate tumor cells. Front Oncol. 2017; 7:11.


6. Ripoll GV, Garona J, Hermo GA, Gomez DE, Alonso DF. Effects of the synthetic vasopressin analog desmopressin in a mouse model of colon cancer. Anticancer Res. 2010; 30:5049–54.
7. Mochizuki S, Soejima K, Shimoda M, Abe H, Sasaki A, Okano HJ, et al. Effect of ADAM28 on carcinoma cell metastasis by cleavage of von Willebrand factor. J Natl Cancer Inst. 2012; 104:906–22.


8. Terraube V, Marx I, Denis CV. Role of von Willebrand factor in tumor metastasis. Thromb Res. 2007; 120 Suppl 2:S64–70.


9. Kawecki C, Lenting PJ, Denis CV. von Willebrand factor and inflammation. J Thromb Haemost. 2017; 15:1285–94.


10. Starke RD, Ferraro F, Paschalaki KE, Dryden NH, McKinnon TA, Sutton RE, et al. Endothelial von Willebrand factor regulates angiogenesis. Blood. 2011; 117:1071–80.


11. Weinberg RS, Grecco MO, Ferro GS, Seigelshifer DJ, Perroni NV, Terrier FJ, et al. A phase II dose-escalation trial of perioperative desmopressin (1-desamino-8-d-arginine vasopressin) in breast cancer patients. Springerplus. 2015; 4:428.


12. Czaplewski C, Kazmierkiewicz R, Ciarkowski J. Molecular modeling of the human vasopressin V2 receptor/agonist complex. J Comput Aided Mol Des. 1998; 12:275–87.
13. Kowalczyk W, Prahl A, Derdowska I, Sobolewski D, Olejnik J, Zabrocki J, et al. Analogues of neurohypophyseal hormones, oxytocin and arginine vasopressin, conformationally restricted in the N-terminal part of the molecule. J Med Chem. 2006; 49:2016–21.


14. Pastrian MB, Guzman F, Garona J, Pifano M, Ripoll GV, Cascone O, et al. Structure-activity relationship of 1-desamino-8-D-arginine vasopressin as an antiproliferative agent on human vasopressin V2 receptor-expressing cancer cells. Mol Med Rep. 2014; 9:2568–72.


15. Garona J, Pifano M, Orlando UD, Pastrian MB, Iannucci NB, Ortega HH, et al. The novel desmopressin analogue [V4Q5]dDAVP inhibits angiogenesis, tumour growth and metastases in vasopressin type 2 receptor-expressing breast cancer models. Int J Oncol. 2015; 46:2335–45.
16. Iannucci NB, Ripoll GV, Garona J, Cascone O, Ciccia GN, Gomez DE, et al. Antiproliferative effect of 1-deamino-8-D-arginine vasopressin analogs on human breast cancer cells. Future Med Chem. 2011; 3:1987–93.


17. Monstein HJ, Truedsson M, Ryberg A, Ohlsson B. Vasopressin receptor mRNA expression in the human gastrointestinal tract. Eur Surg Res. 2008; 40:34–40.


18. Marques I, Araujo A, de Mello RA. Anti-angiogenic therapies for metastatic colorectal cancer: current and future perspectives. World J Gastroenterol. 2013; 19:7955–71.


19. Castle JC, Loewer M, Boegel S, de Graaf J, Bender C, Tadmor AD, et al. Immunomic, genomic and transcriptomic characterization of CT26 colorectal carcinoma. BMC Genomics. 2014; 15:190.


20. Khan S, Cameron S, Blaschke M, Moriconi F, Naz N, Amanzada A, et al. Differential gene expression of chemokines in KRAS and BRAF mutated colorectal cell lines: role of cytokines. World J Gastroenterol. 2014; 20:2979–94.
21. Riihimaki M, Hemminki A, Sundquist J, Hemminki K. Patterns of metastasis in colon and rectal cancer. Sci Rep. 2016; 6:29765.


22. Kim KY, Kim NK, Cha IH, Ahn JB, Choi JS, Choi GH, et al. Novel methods for clinical risk stratification in patients with colorectal liver metastases. Cancer Res Treat. 2015; 47:242–50.


23. Rmali KA, Puntis MC, Jiang WG. Tumour-associated angiogenesis in human colorectal cancer. Colorectal Dis. 2007; 9:3–14.


24. Ripoll G, Iannucci N, Giron S, Cascone O, Gomez D, Alonso D. Angiostatic activity of 1-deamino-8-D-arginine vasopressin and novel peptide analogues in breast cancer cells. Cancer Res. 2008; 68(9 Suppl):295.
25. Horowitz M, Neeman E, Sharon E, Ben-Eliyahu S. Exploiting the critical perioperative period to improve long-term cancer outcomes. Nat Rev Clin Oncol. 2015; 12:213–26.


26. Hirai T, Matsumoto H, Kubota H, Yamaguchi Y. Regulating surgical oncotaxis to improve the outcomes in cancer patients. Surg Today. 2014; 44:804–11.


27. Pifano M, Garona J, Sobol NT, Alberto M, Alonso DF, Ripoll GV. Search of vasopressin analogs with antiproliferative activity on small-cell lung cancer: drug design based on two different approaches. Future Med Chem. 2018; 10:879–94.


28. McEwan DG, Brunton VG, Baillie GS, Leslie NR, Houslay MD, Frame MC. Chemoresistant KM12C colon cancer cells are addicted to low cyclic AMP levels in a phosphodiesterase 4-regulated compartment via effects on phosphoinositide 3-kinase. Cancer Res. 2007; 67:5248–57.


Fig. 1.
Intravenous administration of [V4Q5]dDAVP inhibits metastatic progression of colorectal cancer cells to liver. Mice were inoculated intrasplenically with 1.5×104 CT-26 colorectal carcinoma cells, and after 21-day hepatic metastases were evaluated. [V4Q5]dDAVP peptide was injected at a dose of 0.3 μg/kg intravenous 30 minutes before tumor cell injection, and continued on a three times a week basis until the end of the protocol. (A) Spleens with CT-26 primary tumors from control or [V4Q5]dDAVP-treated mice after 21 days of tumor cell inoculation. (B) Superficial macroscopic colorectal metastasis in liver from control (top) or [V4Q5]dDAVP group (bottom) are depicted. (C) Quantification of total (left) or macrometastatic (> 1 mm of diameter) (right) liver nodules per mice. Metastatic nodules per mouse are expressed as median with range (box and whiskers). Mice weight is expressed as mean and SD. *p < 0.05 and **p < 0.01, unpaired t test.
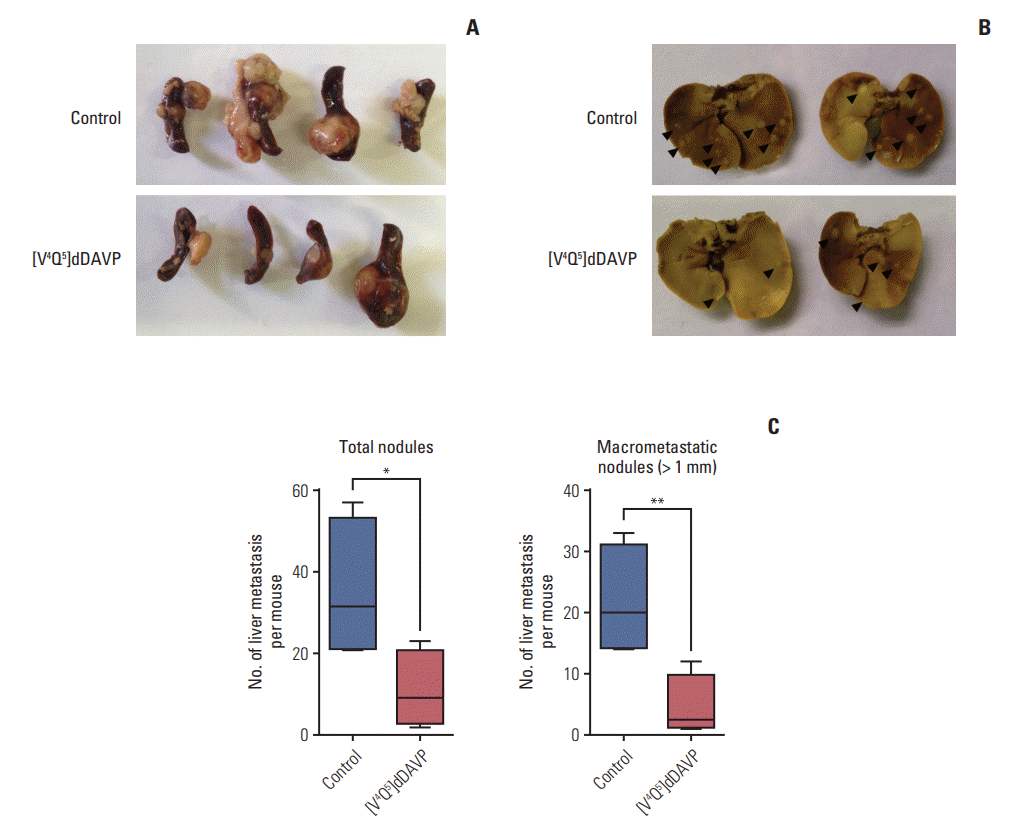
Fig. 2.
Histopathological assessment of colorectal cancer liver metastatic spread inhibition by [V4Q5]dDAVP. Representative microphotographs of livers from control (A, B) mice receiving saline solution, and mice treated with [V4Q5]dDAVP (C, D). Superficial (A, C) and intrahepatic (B, D) metastatic lesions are indicated with “M” in bold (H&E staining, ×40; insets ×200 [magnificated dashed square]). Scale bars=500 μm and 200 μm, respectively.
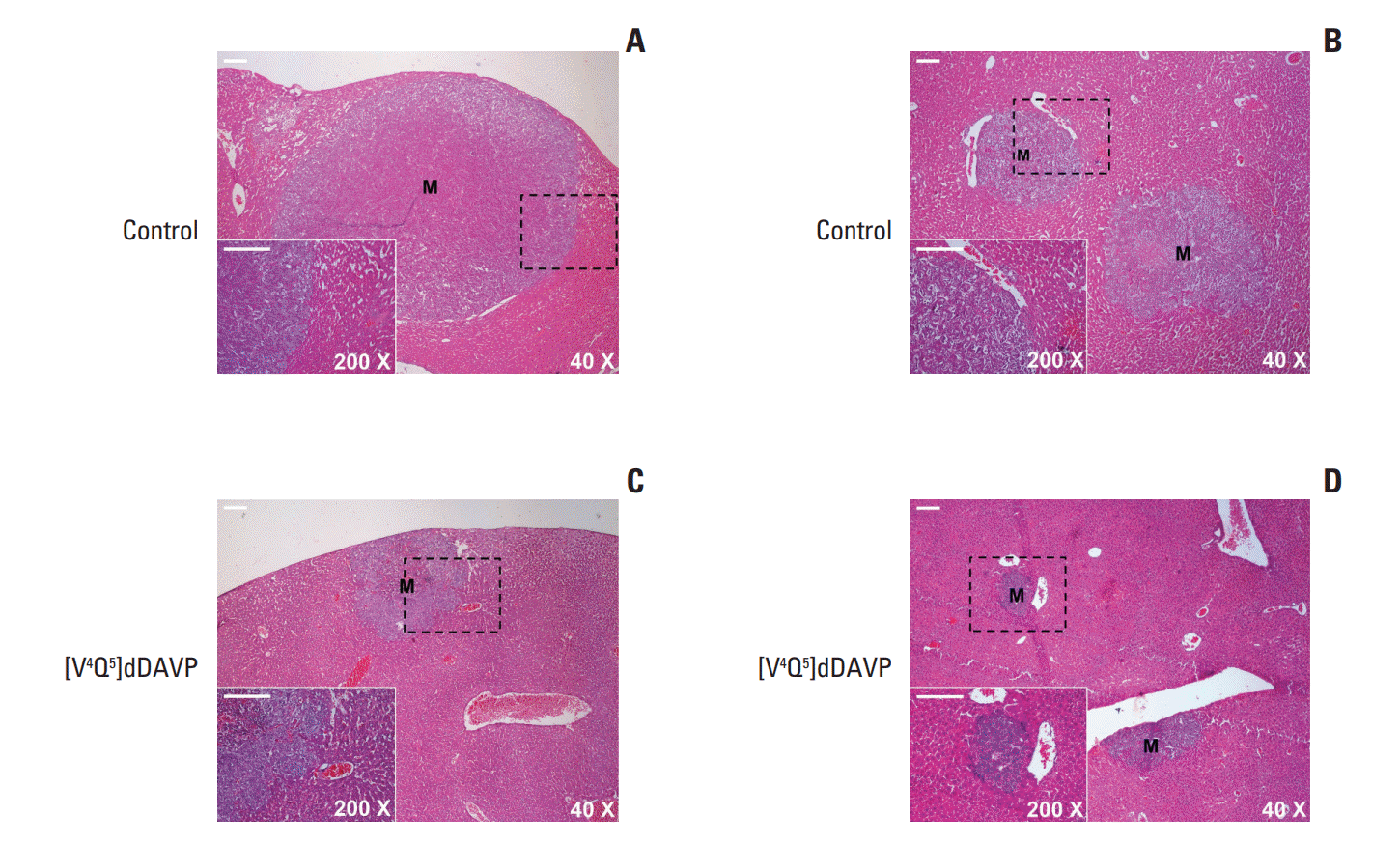
Fig. 3.
Reduction of pulmonary experimental metastatic spread of colorectal cancer cells by intravenous administration of [V4Q5]dDAVP. Mice were inoculated with 2×105 CT-26 colorectal carcinoma cells in the lateral tail vein, and after 21-day pulmonary metastases were evaluated. [V4Q5]dDAVP peptide was injected at a dose of 0.3 μg/kg intravenous 30 minutes before tumor cell injection and 24 hours after. (A) Representative left lung lobes (five most colonized lobes from each group) are depicted. Bouin dye. (B) Weight of colonized lungs from different experimental groups. Lung weights are expressed as median with range (box and whiskers). *p < 0.05, unpaired t test. n=12 animals per experimental group. (C) Representative sections of lungs from control (top) and treated (bottom) animals are depicted (H&E staining, ×40). Scale bars=500 μm.
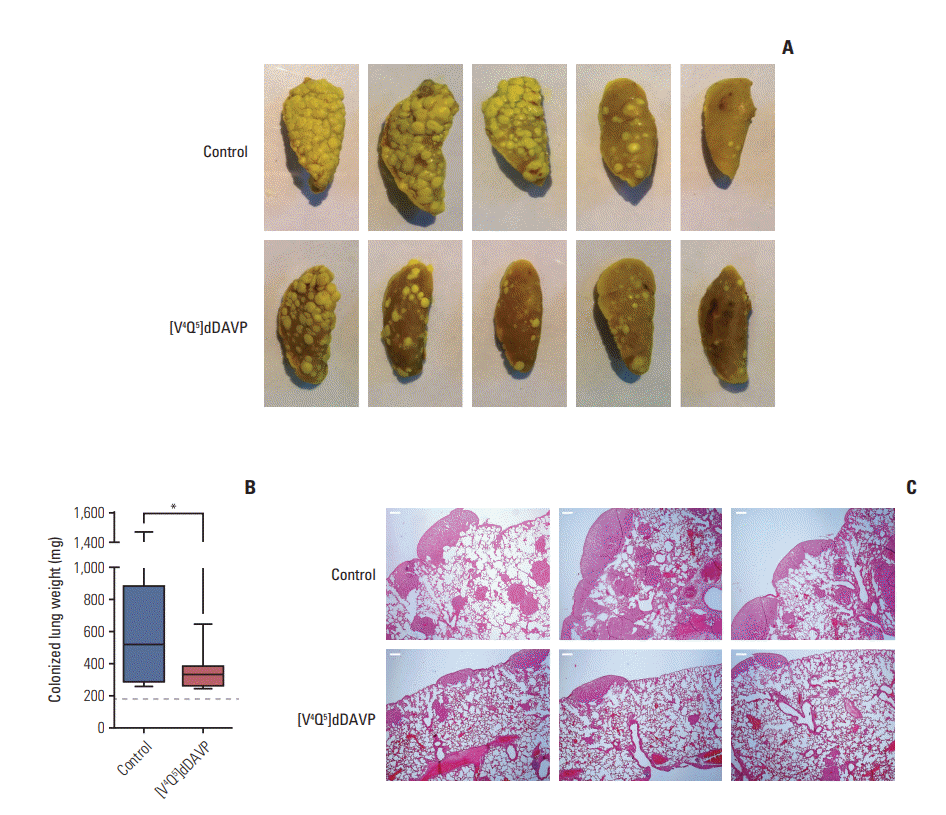
Fig. 4.
Effect of [V4Q5]dDAVP on colorectal tumor-induced angiogenesis and microvascular cell migration and morphogenesis. (A) Colo-205 cell-induced angiogenesis evaluated by a modified-Matrigel plug assay. Representative images of plugs recovered from vehicle or [V4Q5]dDAVP (0.3 μg/kg intravenously, thrice-weekly for 2 weeks) treated mice are depicted. (B) Quantitative assessment of angiogenesis was achieved by the determination of relative hemoglobin content in Matrigel plugs using the Drabkin's method. n=10 animals per experimental group. (C) Quantification of in vitro migration and tube formation by arginine vasopressin type 2 membrane receptor‒expressing microvascular HMVEC-L cells incubated overnight with [V4Q5]dDAVP (1 μM) in comparison to saline vehicle. (D) Crystal violet staining of microvascular endothelial cells that crossed the polycarbonate membrane in the Transwell migration assay (left) and representative images of vasculature-like structures formed by HMVEC-L cells in the morphogenesis assay (right, ×100). Data are presented as mean±standard error of mean and are representative of at least two independent experiments. *p < 0.05, **p < 0.01, ***p < 0.001 vs. control, unpaired t test.
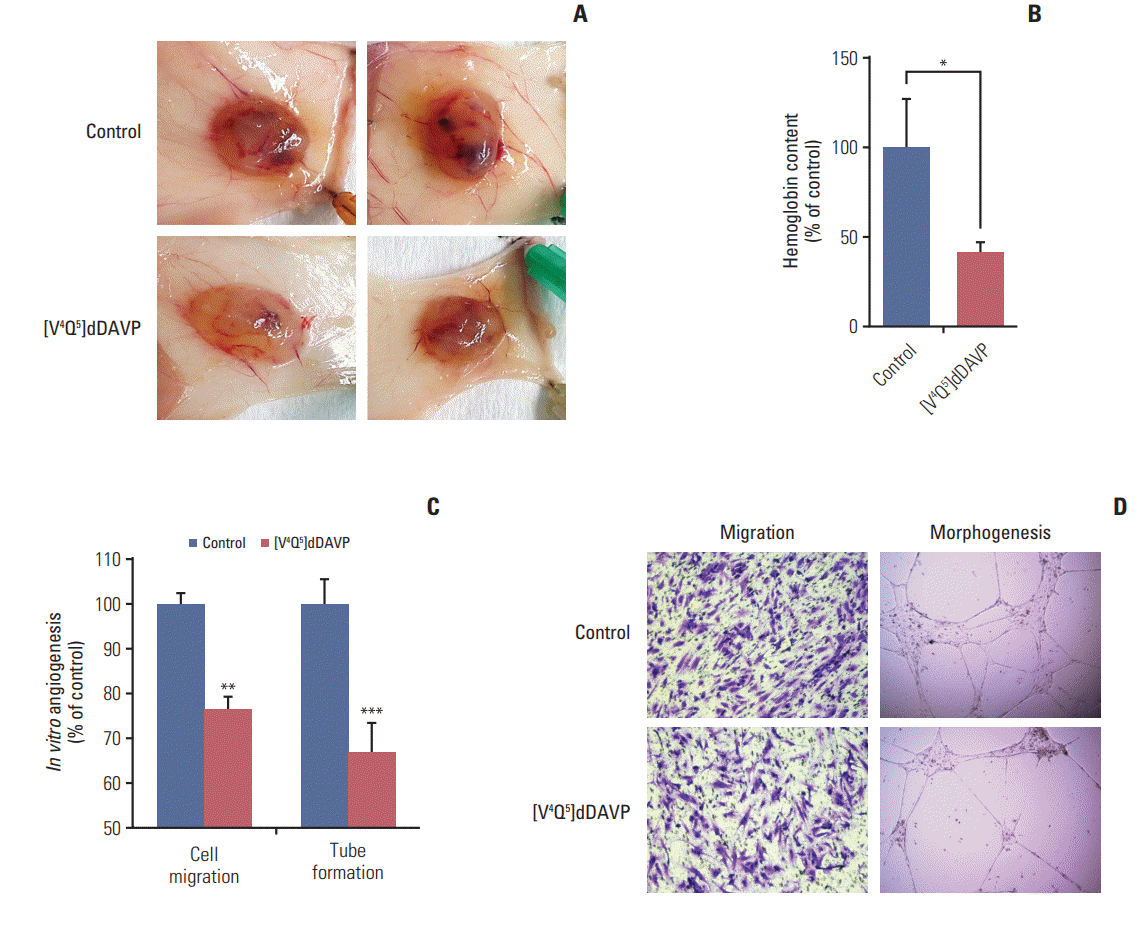
Fig. 5.
In vitro direct cytostatic effects of [V4Q5]dDAVP on colorectal cancer (CRC) cells alone or in combination with standard-of-care chemotherapy. (A) IC50 calculation and reduction of in vitro CT-26 cell colony formation by [V4Q5]dDAVP (right). Dotted line indicates 50% inhibition. Statistical significance (*p < 0.05) in IC50 calculation studies for [V4Q5]dDAVP was achieved with drug concentrations ≥ 1 μM. Representative photographs of vehicle- or [V4Q5]dDAVP-treated low density CT-26 cultures are depicted (left). (B) Reversal of [V4Q5]dDAVP cytostatic effect by addition of arginine vasopressin type 2 membrane receptor selective chemical antagonist tolvaptan. ***p < 0.001, [V4Q5]dDAVP vs. control or [V4Q5]dDAVP plus tolvaptan. (C) IC50 calculation of cytotoxic agent 5-fluorouracil (5-FU) on exponentially growing CT-26 and Colo-205 CRC cells. Statistical significance (*p < 0.05) in IC50 calculation studies for 5-FU in CT-26 and Colo-205 was achieved with drug concentrations ≥ 0.5 or 5 μM, respectively. PBS, phosphate buffered saline. (D) Addition of [V4Q5]dDAVP to sub-IC50 concentrations of 5-FU enhances in vitro cytostatic effect on high density AVPR2-expressing CRC cell cultures. *p < 0.05, **p < 0.01, ***p < 0.001 vs. control. ##p < 0.01 and ###p < 0.001, [V4Q5]dDAVP plus 5-FU vs. [V4Q5]dDAVP or 5-FU individual therapies. Results are representative of at least three independent experiments. Data are presented as mean±standard error of mean. ANOVA plus Dunnet´s test.
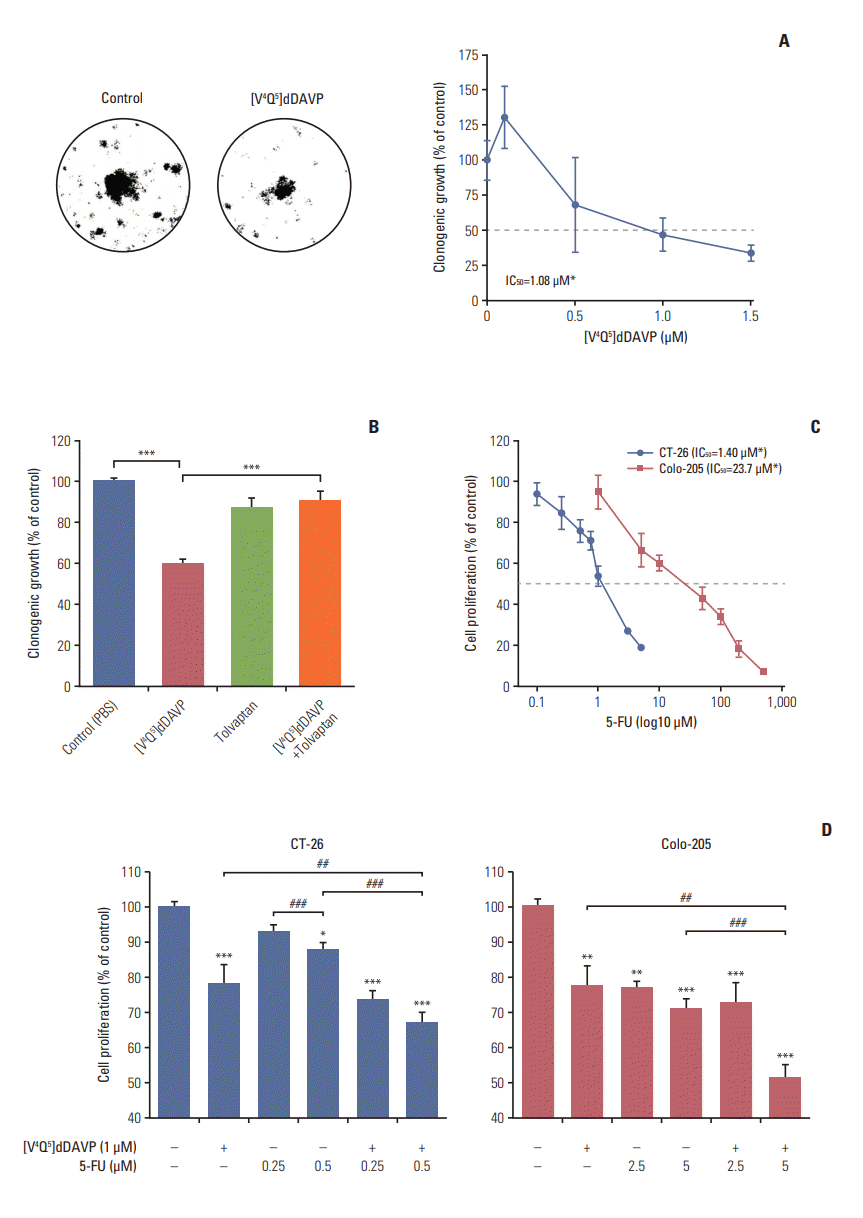