Abstract
Purpose
In some countries with high smoking prevalence, smoke-free legislation has only been implemented in specific public places, as opposed to a comprehensive ban on smoking in all public places. The purpose of this study was to provide valid data on second-hand smoke (SHS) exposure that reflect the consequences of incomplete smoke-free legislation, and provide a rationale for expanding this legislation.
Materials and Methods
Indoor and outdoor environmental exposure (fine particulate matter [PM2.5], air nicotine, and dust 4-(methylnitrosamino)-1-(3-pyridyl)-1-butanone [NNK]) was monitored in 35 public places where smoking is prohibited by law in Goyang, Republic of Korea. Biomarkers of SHS exposure (urinary cotinine, hair nicotine, and urinary 4-(methylnitrosamino)-1-(3-pyridyl)-1-butanol) were measured in 37 non-smoking employees. Geometric means and standard deviations were used in comparison of each measure.
Results
Considerable exposure of SHS was detected at all indoor monitoring sites (PM2.5, 95.5 μg/m3 in private educational institutions; air nicotine, 0.77 μg/m3 in large buildings; and dust NNK, 160.3 pg/mg in large buildings); environmental measures were higher in private or closed locations, such as restrooms. Outdoor measures of SHS exposure were lowest in nurseries and highest in government buildings. Biochemical measures revealed a pattern of SHS exposure by monitoring site, and were highest in private educational institutions.
Conclusion
The evidence of SHS exposure in legislative smoke-free places in Korea suggests that incomplete smoke free legislation and lack of enforcement of it might not protect people from exposure to smoke. Therefore, active steps should be taken toward a comprehensive ban on smoking in all public places and its enforcement.
“Forced smoking” or second-hand smoke (SHS) is considered a critical issue in the demand for tobacco control measures. SHS exposure is also considered to confer a high risk of adverse health consequences among those who have consciously chosen not to smoke. For this reason, countries on the forefront of successful smoke-free legislation have launched unprecedented public awareness campaigns, and mobilized to advocate a comprehensive smoking ban in public places. Such measures lead to a substantial reduction in smoking prevalence and SHS exposure [1]. However, the total number of smokers worldwide continues to increase due to population growth; 40% of children, 33% of non-smoking men, and 35% of non-smoking women are still exposed to SHS [2]. The Western Pacific region has the highest rate of SHS exposure, though many countries in the region show a downward trajectory in smoking prevalence due to recent tobacco control efforts.
Tobacco control policies in the Republic of Korea have been continuously strengthened since the adoption of the National Health Promotion Act in 1995 [3]. These policies have been accelerated by smoke-free legislation that prohibits smoking in specific public places, and two increases in the tax on tobacco products in 2002 and 2004, respectively. This has led to a rapid decline in smoking prevalence among males over the last two decades (from over 70% in the 1990s to 39% in 2011) [4]. Nevertheless, Korea still has the highest smoking prevalence among male adults worldwide, and reports have shown a relatively high rate of SHS exposure among non-smokers in Korea, which is gradually increasing (42.1% in males and 31.6% in females, in 2007; 45.9% in males and 33.8% in females in 2010) [5,6]. Interestingly, these results come from studies conducted after the implementation of smoke-free policies in Western Pacific countries where tobacco control efforts, including smoke-free legislation, are in the same initial stage as in Korea [2]. This has sparked debate on the effectiveness of smoke-free legislation, and laid the foundation for arguments against mandates that prohibit smoking in specific public places. Therefore comprehensive, valid data on SHS exposure are needed in order to evaluate the effect of smoke-free legislation, and to provide a rationale for their expansion.
Recently, various environmental measures of tobacco constituents have become available for assessment of SHS exposure, including vapor-phase nicotine and fine particulate matter (PM2.5) levels, as well as biochemical measures such as urinary cotinine concentration and hair nicotine concentration. In addition, it has been suggested that levels of tobacco-specific nitrosamines such as 4-(methylnitrosamino)-1-(3-pyridyl)-1-butanone (NNK) concentration in environmental dust, and 4-(methylnitrosamino)-1-(3-pyridyl)-1-butanol (NNAL) concentration in urine are excellent markers of SHS exposure, and show good specificity for tobacco-related health risks [7].
In the current study environmental (PM2.5 levels, air nicotine concentration, and dust NNK concentration) and biochemical (e.g., hair nicotine concentration, urinary cotinine concentration, and NNAL concentration) measures were used to evaluate SHS exposure in public places where incomplete smoke-free legislation has been applied in Korea.
From June to September 2011, PM2.5 levels, air nicotine concentration, and dust NNK concentration were measured in 35 public places where smoking is prohibited by law, which were used as monitoring sites: government buildings (10), large buildings (11), nurseries (6), and private educational institutions (8). There were 115 monitoring locations across the 35 monitoring sites, including lobbies, working offices, elevators, restrooms (male and female), and indoor parking lots. Measurements were also taken from outdoor areas within 1 m of the entrance of each monitoring site. Monitoring sites were randomly sampled from Goyang, a residential satellite city of the capital Seoul, by location and population. Monitoring equipment was concealed, and therefore monitoring was carried out unbeknown to those within the buildings (employees, visitors, etc.). During the monitoring period, one air nicotine sampler was lost in a government building, and three were lost in private educational institutions. Dust NNK concentration was under the limit of detection (LOD) in one nursery and one private educational institution.
At each monitoring site, field researchers completed an observational questionnaire to document active smoking evidence (smell of cigarette smoke, presence of cigarette butts, and witnessing people smoking). They also administered a building questionnaire regarding building characteristics and smoking policy to either the building manager or other appropriate personnel. A reference monitoring site was also chosen. Smoking was banned throughout the grounds of the reference monitoring site, and all employers there declared they were non-smokers.
Informed consent was obtained from 37 non-smokers who worked in the different monitoring sites (15 from government buildings, four from large buildings, 15 from nurseries, and three from private educational institutions). Relevant biological samples were obtained for measurement of biochemical markers of SHS exposure (urinary cotinine, hair nicotine, and urinary NNAL concentrations) in non-smoking employees. Cross-sectional comparison of environmental and biochemical measures by monitoring sites and locations was performed. The current study was approved by the Institutional Review Board of National Cancer Center, Korea.
PM2.5 levels were measured using a MetOne Acrocet 531 Aerosol Particulate Profiler (Grants Pass, OR). Indoor and outdoor PM2.5 monitoring was carried out for a minimum of 30 minutes at each monitoring site, as well as for 10 minutes before entering and after exiting the monitoring site. PM2.5 data were multiplied by 8.33 to adjust for the underestimation of the machine to monitor gravimetric PM2.5 concentration [8].
Air nicotine concentration was measured by a passive sampler: a 37-mm polystyrene sampling cassette holding a Teflon-coated glass fiber filter treated with 4% sodium bisulfate and 5% ethanol. To avoid air circulation (i.e., “dead spots”), the passive samplers were hung in the middle of the monitoring location, 1-2 m from the floor, and at least 1 m away from an open window or a ventilation system, and were not visible or accessible to people. They were hung under the eaves of the entrance, or of windows for outdoor air monitoring. The sampling rate was 24 mL/min and the calculated limit of detection was 0.05 μg/m3 over the 7-day sampling period [9]. The exposed filters were extracted and nicotine was analyzed by gas chromatography using a nitrogen phosphorous detector (7820A, Agilent Technologies, Santa Clara, CA). For quality control purposes, 10% of the samples were duplicates. Final air nicotine concentrations were determined by subtraction of background levels from the blank samples.
Dust NNK concentration was measured using dust samples collected from interior ledges and exterior frames of doors and windows. Filter paper soaked in 50% MeOH and left to dry was used to collect the dust samples. After collection, 4 mL of 100 mM ammonium acetate was added to extract samples from the paper. Samples were analyzed using an Agilent 1260 rapid resolution liquid chromatography (Agilent Technologies) coupled with a triple quadruple mass spectrometer equipped with a Turbo Ion Spray TM source (AB SCIEX, Framingham, MA). Standard curves were made from a blank dust, which was originated by spiking a blank matrix with 100 mM ammonium acetate. The LOD and limit of quantification (LOQ) for NNK concentration were 5 pg/mg (S/N 3.2), 25 pg/mg (S/N 7.2), respectively. Precision (both within- and between-day) of the method was found to be acceptable (coefficient variance < 10%).
A sample of 1 mg of hair was finely cut for measurement of nicotine level, and washed for 1 hour with 1 mL dichloromethane. The high-performance liquid chromatography system and tandem mass spectrometer used were an HP 1100 series (Agilent Technologies) and an API 4000 (Applied Biosystems/MDS Sciex, Toronto, Canada) equipped with an atmospheric pressure chemical ionization interface. The LOD and LOQ for hair nicotine concentration were 0.16 and 0.28 ng/mg, respectively.
Urinary cotinine concentration was measured using a liquid chromatography–tandem mass spectrometry system on an API 4000 using the TurboIonSpray interface and multiple reaction monitoring (Applied Biosystems/MDS Sciex). The LOD and LOQ for urinary cotinine concentration were 1 ng/mL and 2 ng/mL, respectively.
A highly sensitive liquid chromatography–tandem mass spectrometry method was developed to determine urinary NNAL concentration. The method involves liquid-liquid extraction followed by conversion to hexanoate ester derivative, which facilitates separation from interfering urinary constituents by extraction and chromatography, and enhances detection with electrospray ionization mass spectrometry. The LOD and LOQ for urinary NNAL concentration were 0.1 pg/mL and 0.2 pg/mL, respectively. The precision of all three of these methods was found to be statistically acceptable.
Descriptive statistics including the geometric mean and standard deviation were produced for PM2.5 level, air nicotine concentration, and dust NNK concentration across each monitoring site, including indoor and outdoor locations, and then subdivided by monitoring location. Statistical analyses were performed using SAS ver. 9.2 (SAS Inc., Cary, NC).
Considerable PM2.5 levels (95.5 μg/m3 in private educational institutes), air nicotine concentrations (0.77 μg/m3 in large buildings), and dust NNK concentration (160.3 pg/mg in large buildings) were detected, and biochemical measures showed evidence of SHS exposure. All considered environmental measures of SHS exposure were lowest in nurseries and private educational institutions, whereas the highest measures were observed in large buildings (Table 1). Air nicotine and dust NNK concentrations in large buildings were 2.9-fold that in private educational institutions, and 17.6-fold that in nurseries. PM2.5 levels in private educational institutions were 6-fold that in nurseries, and more than approximately 4-fold that of World Health Organization guidelines (25 μg/m3 for 24 hours). In the reference monitoring site, even air nicotine and NNK should not be detected in places where there is no exposure to smoke, air nicotine concentration was not detected, and the PM2.5 level was relatively low, while, unexpectedly, the dust NNK concentration was slightly higher than that in nurseries (Table 1).
Air nicotine concentration did not differ markedly across the open monitoring locations of the different monitoring sites. However, it was quite high in private or closed locations, such as restrooms, elevators, and indoor parking lots. Similar patterns were observed for dust NNK concentration. The higher dust NNK concentration in the reference monitoring site was caused by the high concentration in some of the monitoring locations, such as restrooms and elevators (Table 2).
PM2.5, air nicotine concentration, and dust NNK concentration were detected outdoors at all monitoring sites. Outdoor measures of SHS exposure were lowest in nurseries and highest in government buildings. Air nicotine concentration was higher in outdoor than in indoor areas of government buildings, and a similar pattern was observed in PM2.5 levels. Dust NNK concentration was higher in outdoor areas of nurseries and private educational institutions (Table 3).
Of all monitoring sites, the non-smoking policy was strictly enforced in 16.7% (6 sites), cigarettes could be purchased at 19.4% (7 sites), and evidence of active smoking was found in 25% (9 sites). None of the environmental measures differed when these factors were taken into account, nor was any consistency found among the three environmental measures (data not shown).
The hair nicotine concentrations, urinary cotinine concentrations, and urinary NNAL concentrations observed in the current study suggested the existence of SHS exposure. The results of these biochemical measures were the highest among those who worked in private educational institutions and the lowest in those who worked in nurseries (Fig. 1).
In many countries where smoking prevalence is still high and smoke-free legislation is in its initial stages, governments may consider passing less strict smoke-free legislation, or only partial smoking bans, with a promise to strengthen these policies gradually. This approach is sometimes used to minimize negative societal reaction, including that of smokers or tobacco companies [10]. The current study provides evidence of continued SHS exposure in public places where smoking is prohibited by law in Korea, a country that has passed the kind of incomplete smoke-free legislation described above. In addition, the monitoring results reported in this study showed that there are negative consequences to incomplete smoke-free legislation, and to lack of enforcement of such legislation.
According to the National Health Promotion Act of Korea, smoking in central and local government buildings (since 2003), large buildings (since 1995), and private educational institutions (since 1995) is prohibited by law. The entirety of nurseries and their grounds are non-smoking areas (since 2003). This smoke-free legislation was established according to the articles of the World Health Organization Framework Convention on Tobacco Control, and applied at the national level [3,6,11]. However, in our study the environmental and biochemical measures of SHS exposure indicated the presence of SHS exposure in all of the sites investigated. In particular, the fact that private educational institutions and nurseries showed a significant level of SHS exposure should be considered for stricter enforcement of the smoke free law, because these places are intended for children and adolescents who should be protected from SHS exposure.
We monitored indoor and outdoor PM2.5 levels, air nicotine concentration and dust NNK concentration, and the results showed similar patterns by monitoring site and monitoring location, particularly for air nicotine concentration and dust NNK concentration. The mean PM2.5 level, which is widely used when monitoring for SHS exposure, was higher than the guidelines set by the World Health Organization for outdoor air quality, and higher than results from a previous study of sites where smoking is prohibited by law in Scotland [12,13]. Air nicotine concentrations were lower than those found in Argentina (2.60 [1.43-2.99] μg/m3), but similar or higher than those found in the same types of sites in other countries in Latin America (i.e., < LOD [< LOD–0.004] μg/m3 in Panama, and 0.01 [< LOD–0.05] μg/m3 in Guatemala) and China (0.48 [0.21–1.23] μg/m3) [14-16]. In particular, a considerable dust NNK concentration was detected at all monitoring sites including the reference monitoring site.
Worse, no strict enforcement of the smoking prohibition was identified in some monitoring sites. The environmental measures were much higher in relatively private or closed locations such as restrooms, elevators, and indoor parking lots. At large buildings, nurseries, and private educational institutions, some environmental measures were higher outdoors than indoors. Incomplete smoke-free legislation, which designates specific public places as smoke-free as opposed to a comprehensive ban on smoking in all public places, could lead smokers working or visiting in smoke-free buildings to smoke more frequently at any locations inside of the buildings to avoid eyes of others or outside of buildings which are not included in the legislation but close to legislative smoke-free buildings. It might be a kind of ‘balloon effect’ caused by incomplete smoking-free legislation, and the lack of enforcement of this legislation. It leaves people without protection from SHS exposure, and might also explain why the rate of SHS exposure has been gradually increasing in Korea in spite of smoke-free legislation.
As a meaningful finding of the current study, measure of dust NNK, which is the strongest of the nitrosamine carcinogens, suggested the potential for indirect SHS exposure (third-hand smoke) due to cumulative smoking rather than current smoking as well as more frequent illegal smoking [17,18]. At the reference site, where smoking had been banned by institutional rule for over a decade, thus, it is unlikely that any active smoking took place, higher dust NNK concentration was found in restrooms and elevators, where employees and visitors likely stop after smoking outside the building, implicating the possibility of third-hand smoke exposure. On the other hand, higher dust NNK concentration in more private or closed locations at each site could be evidence of more frequent illegal smoking in legislative smoke free buildings.
In this study smoking-specific biochemical measures, such as hair nicotine concentration, urinary cotinine concentration and urinary NNAL concentration were detected in non-smoking employees, although the concentrations were not high. The concentrations of these biochemical measures among non-smokers are useful for evaluation of overall personal exposure [19]. While each marker has a different half-life, and it is possible that subjects had other sources of SHS exposure, the concentrations of hair nicotine, urinary cotinine, and NNAL showed similar patterns by monitoring site, which suggests possible long- and short-term SHS exposure in these public places where smoking is prohibited by law.
Despite these meaningful implications, there are a few limitations in this study. First, because the current study has a cross-sectional design, the results cannot compare SHS exposure reduction before and after smoke-free legislation came into effect. However, rather than identify the reduction in SHS exposure after the introduction of smoke-free legislation, the current study focused on whether considerable SHS or potential thirdhand smoke exposure exists in the areas where smoking is now prohibited by law. To do this we compared our data with that of a reference site where smoke-free legislation has been strictly enforced since its launch, in addition to collecting various standardized environmental and biochemical monitoring data [7]. Second, we could not identify a meaningful difference in monitoring results according to enforcement of smoke-free legislation or active smoking evidence, although evaluation of how smoke-free legislation is implemented and its effect on SHS exposure is important. This lack of meaningful difference might be caused by inaccurate information on the implementation and enforcement of smoke-free legislation due to masking the lack of implementation, which is illegal, or to how questions on how smoking policy was to be implemented or enforced were asked. Evidence of active smoking was also observed in our study, but in normally non-smoking areas it is rare to find cigarette butts or smokers; therefore, most active smoking evidence was based on the presence of cigarette odor. However, this limitation would not affect the study results and implications, which suggest the necessity of a comprehensive smoking ban and strict enforcement of smoke-free policies. Third, the environmental and biochemical measures did not exactly correspond by monitoring site, which created difficulties in explaining the results, even if overall the results showed similar patterns across monitoring sites. This lack of correspondence could be explained by differences in monitoring duration, i.e., PM2.5 level was measured for 30 minutes with active absorption of air; air nicotine concentration for 7 days with a passive sampler; and dust NNK was a one-time measure, though it consisted of duplicate samples [7]. The different particles or substances targeted by each environmental measure could also be involved in this lack of correspondence; PM2.5, for example, is not a tobacco-specific measure and can be significantly affected by any other particles of similar size and outdoor air pollutants, therefore, assessing the impact of SHS directly may be difficult, whereas the other measures such as air nicotine and dust NNK are tobacco-specific measures and cannot be affected by other factors [7]. Last, the number of participants who provided samples for biochemical measures varied by monitoring site, depending on the total number of employees, the number of non-smokers among employees, and the number of people who agreed to participate and signed informed consent forms. However, refusal to participate is very rare and should not have affected the study results. Indeed, biochemical measures and environmental measures showed corresponding patterns.
The evidence of SHS exposure in legislative smoke free places in Korea, suggests that incomplete smoke free legislation and lack of enforcement might not protect people from exposure to smoke. Therefore, active steps should be taken toward a comprehensive ban on smoking in all public places and its enforcement.
Furthermore, to the best of our knowledge, this study represents the first comprehensive field monitoring study to provide comprehensive scientific evidence for continuous SHS exposure and potential third-hand smoke exposure in the Western Pacific region, where the rate of smoking is the highest in the world and the population is accustomed to a smoking environment. In addition, the negative consequences of incomplete smoke-free legislation and less strict enforcement of that legislation were introduced. Therefore, the current study results might provide a considerable reference to understanding what type of legislation and policies are effective in creating smoke-free environments, and which policies can have a real health benefit for the populations of countries in situation similar to that of Korea.
ACKNOWLEDGMENTS
This study was supported by the National Cancer Center (grant nos. NCC-1010250, NCC-1310060) and Regional Government of Goyang (1131110-1).
References
1. Callinan JE, Clarke A, Doherty K, Kelleher C, et al. Legislative smoking bans for reducing secondhand smoke exposure, smoking prevalence and tobacco consumption. Cochrane Database Syst Rev. 2010; (4):CD005992.


2. Eriksen M, Mackay J, Ross H. The tobacco atlas. 4th ed. Atlanta, GA: American Cancer Society;2012.
3. Korea Ministry of Government Legislation. National Health Promotion Act of Korea [Internet]. Sejong: Korea Ministry of Government Legislation;[cited 2013 May 1]. Available from: http://www.moleg.go.kr/main.html.
4. Korean Ministry of Health and Welfare. Korea Ministry of Health and Welfare website [Internet]. Sejong: Korean Ministry of Health and Welfare;[cited 2013 Sep 18]. Available from: http://www.mw.go.kr/front_new/al/sal0301vw.jsp?PAR_MENU_ID=04&MENU_ID=0403&page=199&CONT_SEQ=257768.
5. Organization for Economic Co-operation and Development. OECD Health Statistics 2014: Frequently Requested Data [Internet]. OECD;2014. [cited 2015 Mar 30]. Available from: http://www.oecd.org/els/health-systems/oecd-health-statistics-2014-frequently-requested-data.htm.
6. Korean National Health and Nutrition Examination Survey (KNHANES) [Internet]. Cheongju: Korea Centers for Disease Control and Prevention;[cited 2013 May 1]. Available from: http://knhanes.cdc.go.kr/knhanes/index.do.
7. Apelberg BJ, Hepp LM, Avila-Tang E, Gundel L, Hammond SK, Hovell MF, et al. Environmental monitoring of secondhand smoke exposure. Tob Control. 2013; 22:147–55.


8. Lee K, Hahn EJ, Pieper N, Okoli CT, Repace J, Troutman A. Differential impacts of smoke-free laws on indoor air quality. J Environ Health. 2008; 70:24–30. 54.
9. Hammond SK, Leaderer BP. A diffusion monitor to measure exposure to passive smoking. Environ Sci Technol. 1987; 21:494–7.


10. World Health Organization. World report on the global tobacco epidemic, 2013: enforcing bans on tobacco advertising, promotion and sponsorship. Geneva: World Health Organization;2013.
11. World Health Organization. WHO Framework Convention on Tobacco Control [Internet]. Geneva: World Health Organization;[cited 2013 Sep 18]. Available from: http://www.who.int/fctc/en/.
12. World Health Organization. WHO Air quality guidelines: global update 2005. Copenhagen: World Health Organization Regional Office for Europe;2006.
13. Semple S, Creely KS, Naji A, Miller BG, Ayres JG. Secondhand smoke levels in Scottish pubs: the effect of smoke-free legislation. Tob Control. 2007; 16:127–32.


14. Navas-Acien A, Peruga A, Breysse P, Zavaleta A, Blanco-Marquizo A, Pitarque R, et al. Secondhand tobacco smoke in public places in Latin America, 2002-2003. JAMA. 2004; 291:2741–5.


15. Stillman F, Navas-Acien A, Ma J, Ma S, Avila-Tang E, Breysse P, et al. Second-hand tobacco smoke in public places in urban and rural China. Tob Control. 2007; 16:229–34.


16. Barnoya J, Mendoza-Montano C, Navas-Acien A. Secondhand smoke exposure in public places in Guatemala: comparison with other Latin American countries. Cancer Epidemiol Biomarkers Prev. 2007; 16:2730–5.


17. Schick SF, Farraro KF, Perrino C, Sleiman M, van de Vossenberg G, Trinh MP, et al. Thirdhand cigarette smoke in an experimental chamber: evidence of surface deposition of nicotine, nitrosamines and polycyclic aromatic hydrocarbons and de novo formation of NNK. Tob Control. 2014; 23:152–9.


Fig. 1.
Geometric mean of hair nicotine concentration, urinary cotinine concentration, and urinary NNAL concentration in non-smoking full-time employees. NNAL, 4-(methylnitrosamino)-1-(3-pyridyl)-1-butanol.
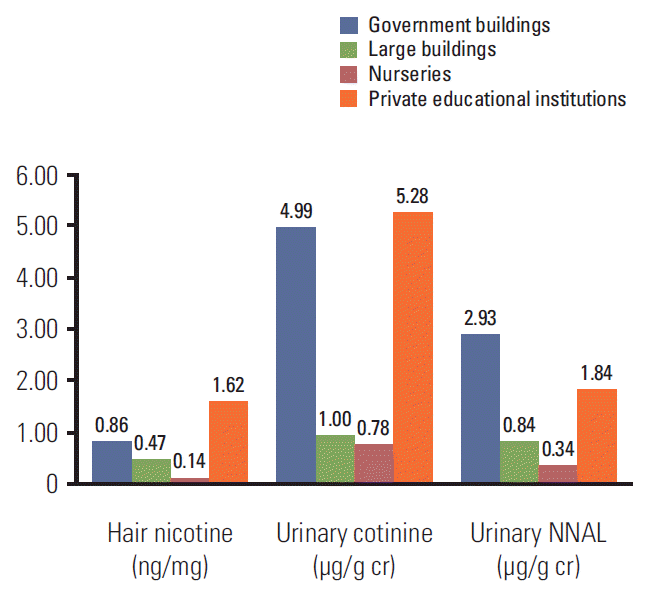
Table 1.
PM2.5 level, air nicotine concentration, and dust NNK by type of monitoring site
Table 2.
PM2.5 level, air nicotine concentration, and dust NNK by monitoring location at different monitoring sites
Table 3.
Comparison of PM2.5 level, air nicotine concentration, and dust NNK in indoor and outdoor locations