Abstract
Purpose
We examined clinical and dosimetric factors as predictors of symptomatic radiation pneumonitis (RP) in lung cancer patients and evaluated the relationship between interstitial lung changes in the pre-radiotherapy (RT) computed tomography (CT) and symptomatic RP.
Materials and Methods
Medical records and dose volume histogram data of 60 lung cancer patients from August 2005 to July 2006 were analyzed. All patients were treated with three dimensional (3D) conformal RT of median 56.9 Gy. We assessed the association of symptomatic RP with clinical and dosimetric factors.
Results
With a median follow-up of 15.5 months (range, 6.1 to 40.9 months), Radiation Therapy Oncology Group grade ≥ 2 RP was observed in 14 patients (23.3%). Five patients (8.3%) died from RP. The interstitial changes in the pre-RT chest CT, mean lung dose (MLD), and V30 significantly predicted RP in multivariable analysis (p=0.009, p < 0.001, and p < 0.001, respectively). MLD, V20, V30, and normal tissue complication probability normal tissue complication probability (NTCP) were associated with the RP grade but less so for grade 5 RP. The risk of RP grade ≥ 2, ≥ 3, or ≥ 4 was higher in the patients with interstitial lung change (grade 2, 15.6% to 46.7%, p=0.03; grade 3, 4.4% to 40%, p=0.002; grade 4, 4.4% to 33.3%, p=0.008). Four of the grade 5 RP patients had diffuse interstitial change in pre-RT CT and received chemoradiotherapy.
Thoracic radiation therapy (RT) is an important component of treatment for locally advanced non-small cell lung cancer and limited stage small cell lung cancer [1-4]. The fundamental goal of RT is to deliver an effective dose for local control and keep toxicity to an acceptable level in the surrounding normal tissues. However, irradiation of the thorax is frequently accompanied by harmful side effects. One major concern is radiation pneumonitis (RP), which can lead to severe respiratory dysfunction, including chronic respiratory insufficiency caused by the development of pulmonary fibrosis, and even death [5,6].
Clinical risk factors for the development of RP include older age, disease location in the mid-lower lung, chemotherapy-schedule, and the presence of comorbidity [7,8]. Additionally, many studies suggest dosimetric factors relate to RP [9-12].
Several studies reported that patients with interstitial lung disease (ILD) developed severe RP after thoracic stereotactic body radiotherapy (SBRT) [13,14]. Acute exacerbation of ILD can cause respiratory failure and death. Severe ILD was regarded as a relative contraindication in the clinical guidelines for SBRT published by the Japanese Society for Therapeutic Radiation and Oncology [15]. There is limited data showing an association of subclinical ILD with RP [16,17], and whether subclinical ILD is a risk factor for thoracic RT is a matter of speculation.
We examined clinical and dosimetric factors as predictors of symptomatic RP in lung cancer patients and further evaluated the relationship of pre-radiation interstitial changes in the computed tomography (CT) and symptomatic RP.
The medical records and dose volume histogram (DVH) data of 60 lung cancer patients, treated with three dimensional (3D) conformal RT from August 2005 to July 2006 at Seoul St. Mary's Hospital, were retrospectively collected and analyzed. At least 6 months of follow-up was required for inclusion in this study. Patients who died within 6 months after completion of RT, were lost to follow-up, or did not complete treatment, were excluded from the analysis.
Patient characteristics are summarized in Table 1. All 60 patients were treated with curative intent, using 3D conformal RT. Forty-eight patients (80%) received chemotherapy as a part of their definitive treatment. Twenty-seven patients (45%) received chemotherapy prior to RT and 21 patients (35%) received concurrent chemoradiotherapy. The regimens used for concurrent chemotherapy were etoposide and cisplatin (8 patients), docetaxel and cisplatin (8 patients), taxol (4 patients), and cisplatin (1 patient). Fourteen patients (23.3%) received RT or concurrent chemoradiotherapy as definitive salvage treatment following recurrent disease after surgical intervention (lobectomy eight patients, wedge resection two patients, pneumonectomy one patient, and others three patients). Fifty-two patients (86.7%) showed a good performance status of more than Eastern Cooperative Oncology Group (ECOG) 2. Each patient’s pre-RT chest CT scans were reviewed by two radiation oncologists and one diagnostic radiologist who were not informed of which patients developed symptomatic RP. Fifteen patients (25%) had interstitial changes on their chest CT scans. Interstitial changes were recorded if any of the following criteria were met: subpleural ground glass opacity (GGO), fibrous reticulation, traction bronchiectasis, micronodules, honey-comb appearance, or volume loss, especially in both lower lobes [18]. Thoracic RT was not performed in patients with clinical ILD, which was defined as a status of symptomatic disease or post-treatment with high probability of acute exacerbation. The patients showing interstitial change on pre-RT CT without related symptom or history of a treatment were included in this study as subclinical ILD. This retrospective study was approved by the hospital’s institutional review board.
A planning CT scan with contrast enhancement of the entire lung volume was acquired with a vac-lock immobilization device at shallow normal breathing. Four dimensional CT (4D-CT) scans were also acquired in all patients to evaluate respiratory motion. All patients underwent virtual simulation and treatment planning with the 3D treatment planning system (Pinnacle, ADAC Laboratories, Milpitas, CA). The calculation was performed with lung heterogeneity corrections by radiological path algorithm. The gross tumor volume (GTV) included the tumor and the clinically involved nodes. The GTV node was determined by chest CT and/or positron emission tomography–computed tomography for the majority of patients; the node was determined by mediastinoscopy in a small number of patients. The clinical target volume included the GTV with an additional uniform 5-mm expansion and high risk, uninvolved mediastinal lymph nodes as well as the ipsilateral hilar lymph nodes. The high risk, involved mediastinal lymph nodes were defined as those lymph nodes adjacent to the GTV node with a high probability of microscopic involvement. We added a different panning target volume (PTV) margin based on an assessment of each tumor’s motion using the Leonardo system (Siemens, Concord, CA) in 4D-CT. The lung, esophagus, spinal cord, and heart were contoured. The lung volume was contoured and defined excluding the GTV.
Most patients were treated with conventional beam arrangements (i.e., initial AP/PA fields to include the PTV) for the first 30-40 Gy, followed by an off-cord oblique beam to the PTV, usually composed of three to five beams. Some patients with small PTVs were treated with multiple beam arrangements from the beginning. The median radiation dose was 56.9 Gy (range, 45.0 to 64.8 Gy), generally delivered at 1.8-2 Gy/fraction, five fractions per week. RT was delivered with 10 MV X-ray from a LINAC (Siemens). Generally, the radiation dose was prescribed such that 95% of PTV was covered by 95% of the prescription dose. The lung DVH was calculated directly from the physical dose distribution (i.e., adjustments were not made for fraction size or overall treatment time). The following dosimetric factors were extracted from the lung DVH: V10, V20, V30, V40, mean lung dose (MLD), and normal tissue complication probability (NTCP) as derived from the Lyman and Kutcher models.
A diagnosis of RP was made by the clinical symptoms of nonproductive cough, fever, exertional dyspnea and characteristic chest radiographs and CT findings. Patients were evaluated by radiation oncologists 3 to 6 weeks after completion of RT and at 1- to 3-month intervals thereafter. RP grading was recorded using Radiation Therapy Oncology Group (RTOG) scale, based on the severity of clinical symptoms and radiographic changes. Two radiation oncologists and one diagnostic radiologist who were blinded to RP status reviewed the medical records, chest X-ray and/or chest CT scan of all patients with suspected RP, and ruled out other causes of pneumonia that were confirmed as infectious pneumonia or disease progression.
All statistical analysis in this study was performed with the SPSS ver. 18.0 (SPSS Inc., Chicago, IL). The chi-squared test and Fisher exact test were used to compare RP risk in subgroups and Student’s t test was used for normally distributed continuous variables. To identify independent predictors of RP, all factors were included in a multivariable linear regression model. Using a stepwise procedure, variables with a p-value of 0.05 or higher were dropped from the model so that only significant predictors remained. All statistical tests were two sided and a p-value of less than 0.05 was considered statistically significant.
With a median follow-up of 15.5 months (range, 6.1 to 40.9 months), 22 patients (36.7%) developed RP: eight (13.3%) with grade 1, six (10.0%) with grade 2, one (1.6%) with grade 3, 2 (3.3%) with grade 4, and five (8.3%) with grade 5. RTOG grade 2 or higher RP was observed in 14 patients (23.3%). Five patients (8.3%) died from RP. The median time to the onset of symptoms was 33 days (range, 6 to 160 days) from the completion of RT.
Table 2 shows the relationship between clinical factors and RP. There was a trend of increased risk of RP in patients treated with combined chemoradiation compared with patients treated with RT alone (41.7% vs. 16.7%, p=0.108). However, this difference was not statistically significant. Interstitial change in the pre-RT chest CT was the only clinical factor associated with RP (p=0.033). In multivariable analysis, interstitial changes in the pre-RT chest CT were significantly associated with RP (p=0.009; odds ratio, 10.24; 95% confidence interval, 1.787 to 58.679).
The statistically significant dosimetric factors for predicting RP are shown in Table 3. In the univariable analysis, the radiation field size, MLD, V10, V20, V30, V40, and NTCP were significantly associated with RP (all p < 0.05). From the multivariable analysis, the MLD (p < 0.001) and V30 (p < 0.001 were significant independent predictors for RP. Cumulative curves of the development of RP stratified by MLD, V20, V30, and NTCP are illustrated in Fig. 1. Our data showed that maintaining an MLD less than 15 Gy, V20 ≤ 25 %, and V30 ≤ 20 % could reduce the incidence of RP. Fig. 2 illustrates the distribution of RP grades by MLD, V20, V30, and NTCP. As shown in Fig. 2, five patients with grade 5 RP did not have higher dosimetric parameters compared to non-fatal RP patients.
Fifteen patients (25%) had interstitial changes on their chest CT scans. There was a difference in RP incidence between patients with interstitial lung change in pre-RT CT and without interstitial change (Table 4). RP was detected in nine (60.0%) of the 15 patients with diffuse interstitial change in their pre-RT CT compared to 13 (28.9%) of 45 patients without interstitial changes (p=0.030). Risk of RP grade ≥ 2 was significantly different depending on presence of interstitial change in the patient’s pre-RT CT (p=0.030). In addition, interstitial change in pre-RT CT raised the risk of RP grade ≥ 3 and ≥ 4 from 4.4% to 40% (p=0.002) and 4.4% to 33.3% (p=0.008). Interstitial change in pre-RT chest CT was the only clinical factor associated with RP in the multivariable analysis (p=0.009).
We analyzed the pre-RT chest CT of the RP patients, by focusing on the imaging findings. Of these patients, the characteristic findings of the nine RP patients with diffuse interstitial changes in their pre-RT chest CT scans are summarized in Table 5. These patients presented mixed and various interstitial changes such as honeycombing, reticular opacity, traction brochiectasis, focal subpleural GGO, and micronodular density. However, in the higher grade RP patients, diffuse honeycombing and diffuse nodular density patterns were frequently observed involving the entirety of both lungs diffusely, or occasionally involving both lower lung fields. Fig. 3 shows a typical representative case of a patient who progressed to fatal RP. Four of the grade 5 RP patients had interstitial changes in their pre-RT chest CT and also received chemotherapy: concurrent chemoradiotherapy in two patients, and sequential chemotherapy followed by RT in two patients.
Table 6 gives a detailed description of five patients with grade 5 RP. Three patients initially developed focal consolidation corresponding to the radiation fields during RT or within one month after RT completion, and the lesions progressed to the entire lung beyond radiation fields. The other two patients presented with bilateral lung infiltration 4 to 6 weeks after RT completion and died following rapid progression. No pathogens were identified and steroid and antibiotic treatments were not effective.
In this study, we examined clinical and dosimetric factors as predictors of symptomatic RP in lung cancer patients and further evaluated the relationship of pre-radiation interstitial changes in the CT with symptomatic RP, as there are limited data for interstitial change in pre-RT CT regarding RP. The RP risk differed significantly by the presence of interstitial lung change in the pre-RT CT. The risk of RP grade ≥ 2 or ≥ 3 was elevated in patients with interstitial change in their pre-RT CT scans. Interstitial change in the pre-RT chest CT scan was the only clinical factor associated with RP in the multivariable analysis.
Patient performance status, age, sex, smoking status, chemotherapy exposure, tumor site, and pre-RT pulmonary function test have been implicated in RP [7,8]. Several studies [12,19] showed that the chemotherapy schedule predicts RP, although the studies were limited by varied drug regimens and schedules. Similar results were obtained from this study. The percentage of patients who developed RP in our study was higher in patients with combined chemoradiation compared with RT alone (41.7% vs. 16.7%, respectively), but this difference did not reach statistical significance (p=0.108), perhaps due to small sample size.
We noted that MLD and V30 significantly predicted RP. In our studies, all RP grades were associated with dosimetric factors, such as MLD V20, V30, V40, and NTCP, similar to other reports [9-12], except in the cases of grade 5 fatal RP. The five patients with grade 5 RP in our study did not have worse dosimetric parameters than those with non-fatal RP. RP is usually limited to the irradiation field; however, in grade 5 RP patients of our study, their chest CT scans were characterized by diffuse interstitial infiltration and consolidation even outside the radiation field in the absence of an identifiable infectious organism. This suggests that other biologic factors might have contributed to RP deaths.
We analyzed the pre-RT chest CT scans of the RP patients and frequently observed diffuse honeycombing and diffuse nodular density patterns in high grade RP. Patients whose pre-RT chest CT scans revealed diffuse interstitial changes in either the entirety of both lungs or both lower lung fields had a greater risk of high grade RP. These patients must be carefully watched during chemoradiation and closely monitored following RT due to the increased risk. This is the essential point: the physician must monitor carefully even patients who are within the acceptable range results for the pulmonary function test prior to RT, even those with no history of symptomatic ILDs. Several Japanese studies have suggested that subclinical ILD found in CT scans before thoracic RT is related to fatal RP, and those patients have to be carefully evaluated [16,17,20]. Our study found that patients with interstitial lung change in CT have a significantly higher risk of severe RP, and that the interstitial change is a statistically significant prognostic factor for severe RP. Yamaguchi et al. [16] suggested that extensive interstitial change in pre-RT CT is a contraindication for thoracic RT. In our study, we obtained similar results. In addition we showed that five patients with grade 5 RP did not have higher dosimetric parameters compared to the non-fatal RP patients. Even if patients have reasonable dosimetric results in their radiotherapy planning, it is necessary to carefully evaluate and follow up with the patient due to their high risk of severe RP.
Several studies suggest that certain biological or physiological responses to radiation could explain RP and that these are not well predicted by the empiric dose-volume relationship. Further research that takes into consideration biologic markers may be necessary [21]. Tumor growth factor β1, interleukins 1, 6, 8, and 10, Krebs von den Lungen-6 (KL-6) and surfactant proteins (SP) are important biologic markers in the development of symptomatic radiation-induced lung injury. Interstitial change on the CT and high levels of biologic markers (KL-6, SP-D) were associated with an increased risk of severe RP [22]. Further study with a higher number of patients and several biologic factors is necessary to determine the relationships of interstitial change in pre-RT CT, biologic markers, and severe RP. It is hoped that further research will identify biomarkers that will allow us to tailor our treatment for lung cancer patients to avoid the development of severe RP.
There are several limitations in this study. As a retrospective study, this study has the possibility of selection bias. A small number of patients with short inclusion were analyzed in this study, because we explored this study as the extension of another study for RP. Patient characteristics and radiation treatment were heterogeneous. A prospective study with a larger number of patients will improve the result of this study. Despite these limitations, our findings are helpful to identify and follow the high risk patients of severe RP.
Our study identified diffuse interstitial disease as a significant clinical factor for the occurrence of RP and also showed the usefulness of dosimetric factors such as MLD, V20, V30, and NTCP in predicting the incidence and severity of RP. Four of our five grade 5 RP patients had interstitial changes in their pre-RT chest CTs. When image findings were analyzed in RP patients, diffuse honeycombing and diffuse nodular patterns were frequently observed in high grade RP. The patients with interstitial change in pre-RT CT have to be carefully evaluated and managed for severe RP after thoracic RT.
ACKNOWLEDGMENTS
The authors would like to acknowledge the statistical assistance of Catholic Medical Center Clinical Research Coordinating Center.
References
1. Choy H, Akerley W, Safran H, Graziano S, Chung C, Williams T, et al. Multiinstitutional phase II trial of paclitaxel, carboplatin, and concurrent radiation therapy for locally advanced non-small-cell lung cancer. J Clin Oncol. 1998; 16:3316–22.


2. Toschi L, Cappuzzo F, Janne PA. Evolution and future perspectives in the treatment of locally advanced non-small cell lung cancer. Ann Oncol. 2007; 18 Suppl 9:ix150–5.


3. Pignon JP, Arriagada R, Ihde DC, Johnson DH, Perry MC, Souhami RL, et al. A meta-analysis of thoracic radiotherapy for small-cell lung cancer. N Engl J Med. 1992; 327:1618–24.


4. De Ruysscher D, Pijls-Johannesma M, Vansteenkiste J, Kester A, Rutten I, Lambin P. Systematic review and meta-analysis of randomised, controlled trials of the timing of chest radiotherapy in patients with limited-stage, small-cell lung cancer. Ann Oncol. 2006; 17:543–52.


5. Morgan GW, Breit SN. Radiation and the lung: a reevaluation of the mechanisms mediating pulmonary injury. Int J Radiat Oncol Biol Phys. 1995; 31:361–9.


6. McDonald S, Rubin P, Phillips TL, Marks LB. Injury to the lung from cancer therapy: clinical syndromes, measurable endpoints, and potential scoring systems. Int J Radiat Oncol Biol Phys. 1995; 31:1187–203.


7. Vogelius IR, Bentzen SM. A literature-based meta-analysis of clinical risk factors for development of radiation induced pneumonitis. Acta Oncol. 2012; 51:975–83.


8. Palma DA, Senan S, Tsujino K, Barriger RB, Rengan R, Moreno M, et al. Predicting radiation pneumonitis after chemoradiation therapy for lung cancer: an international individual patient data meta-analysis. Int J Radiat Oncol Biol Phys. 2013; 85:444–50.


9. Jin H, Tucker SL, Liu HH, Wei X, Yom SS, Wang S, et al. Dose-volume thresholds and smoking status for the risk of treatment-related pneumonitis in inoperable non-small cell lung cancer treated with definitive radiotherapy. Radiother Oncol. 2009; 91:427–32.


10. Baker R, Han G, Sarangkasiri S, DeMarco M, Turke C, Stevens CW, et al. Clinical and dosimetric predictors of radiation pneumonitis in a large series of patients treated with stereotactic body radiation therapy to the lung. Int J Radiat Oncol Biol Phys. 2013; 85:190–5.


11. Dang J, Li G, Ma L, Diao R, Zang S, Han C, et al. Predictors of grade ≥ 2 and grade ≥ 3 radiation pneumonitis in patients with locally advanced non-small cell lung cancer treated with three-dimensional conformal radiotherapy. Acta Oncol. 2013; 52:1175–80.


12. Park YH, Kim JS. Predictors of radiation pneumonitis and pulmonary function changes after concurrent chemoradiotherapy of non-small cell lung cancer. Radiat Oncol J. 2013; 31:34.


13. Togashi Y, Masago K, Handa T, Tanizawa K, Okuda C, Sakamori Y, et al. Prognostic significance of preexisting interstitial lung disease in Japanese patients with small-cell lung cancer. Clin Lung Cancer. 2012; 13:304–11.


14. Yamaguchi S, Ohguri T, Ide S, Aoki T, Imada H, Yahara K, et al. Stereotactic body radiotherapy for lung tumors in patients with subclinical interstitial lung disease: the potential risk of extensive radiation pneumonitis. Lung Cancer. 2013; 82:260–5.


15. Nagata Y, Hiraoka M, Mizowaki T, Narita Y, Matsuo Y, Norihisa Y, et al. Survey of stereotactic body radiation therapy in Japan by the Japan 3-D Conformal External Beam Radiotherapy Group. Int J Radiat Oncol Biol Phys. 2009; 75:343–7.


16. Yamaguchi S, Ohguri T, Matsuki Y, Yahara K, Oki H, Imada H, et al. Radiotherapy for thoracic tumors: association between subclinical interstitial lung disease and fatal radiation pneumonitis. Int J Clin Oncol. 2015; 20:45–52.


17. Sanuki N, Ono A, Komatsu E, Kamei N, Akamine S, Yamazaki T, et al. Association of computed tomography-detected pulmonary interstitial changes with severe radiation pneumonitis for patients treated with thoracic radiotherapy. J Radiat Res. 2012; 53:110–6.


18. Mueller-Mang C, Grosse C, Schmid K, Stiebellehner L, Bankier AA. What every radiologist should know about idiopathic interstitial pneumonias. Radiographics. 2007; 27:595–615.


19. Das SK, Zhou S, Zhang J, Yin FF, Dewhirst MW, Marks LB. Predicting lung radiotherapy-induced pneumonitis using a model combining parametric Lyman probit with nonparametric decision trees. Int J Radiat Oncol Biol Phys. 2007; 68:1212–21.


20. Makimoto T, Tsuchiya S, Hayakawa K, Saitoh R, Mori M. Risk factors for severe radiation pneumonitis in lung cancer. Jpn J Clin Oncol. 1999; 29:192–7.


Fig. 1.
Cumulative curves of the development of RP, which were stratified by MLD (A), V20 (B), V30 (C), and NTCP (D). There were consistencies in that the higher RP rates were associated with higher MLD, V20, V30, and NTCP. Maintaining a MLD less than 15 Gy, V20 < 25%, and V30 < 20% could reduce the incidence of RP. RP, radiation pneumonitis; MLD, mean lung dose; V20 and V30, percent lung volume receiving more than 20 Gy and 30 Gy; NTCP, normal tissue complication probability.
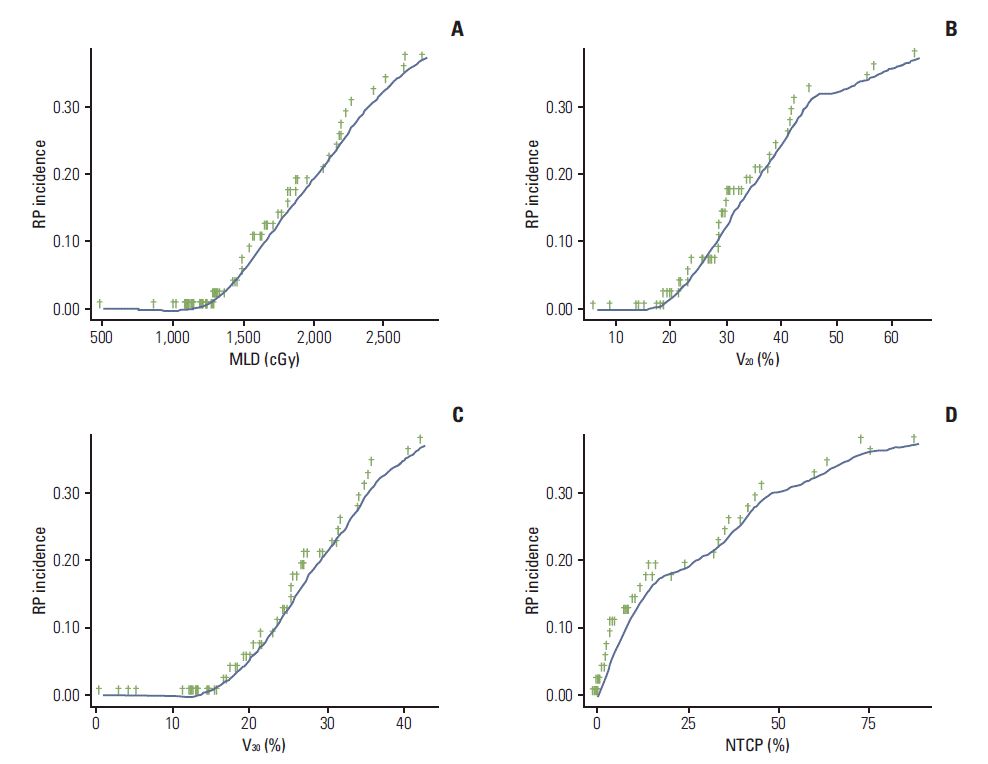
Fig. 2.
The distribution of RP grade stratified by MLD (A), V20 (B), V30 (C), and NTCP (D). A MLD, V20, V30, V40, and NTCP also were strongly associated with RP grade. However, all dosimetric parameters were even lower in grade 5 fatal RP. RP, radiation pneumonitis; MLD, mean lung dose; V20 and V30, percent lung volume receiving more than 20 Gy and 30 Gy; NTCP, normal tissue complication probability.
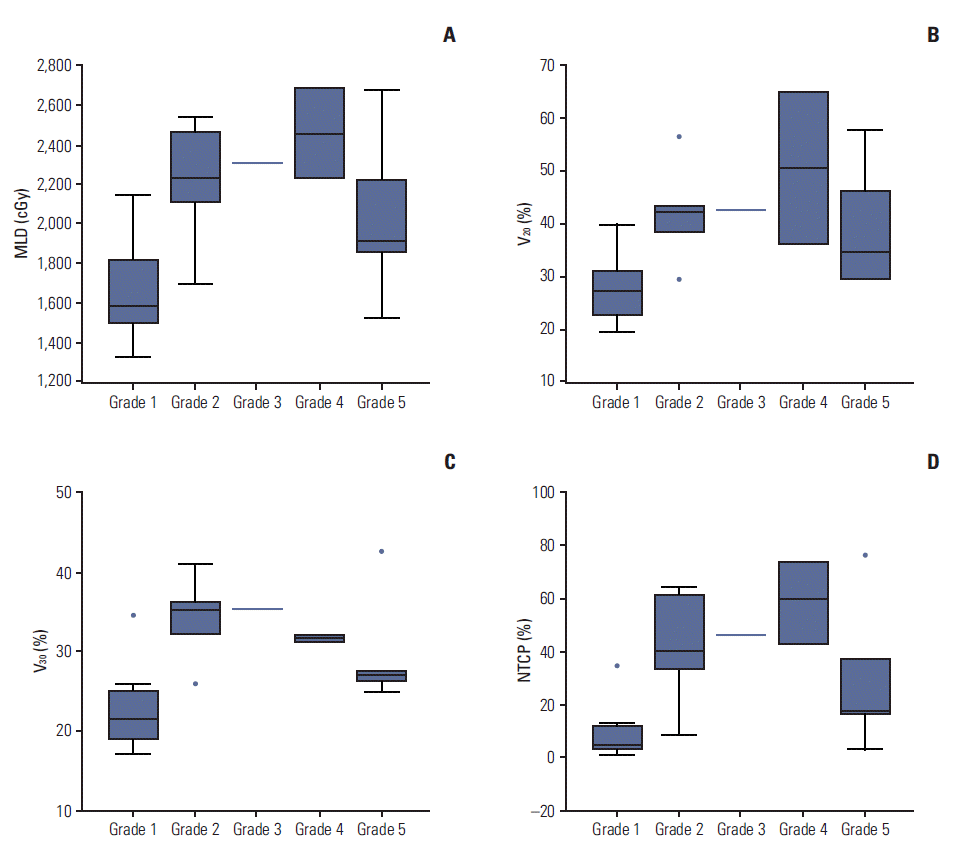
Fig. 3.
Representative of grade 5 fatal radiation pneumonitis patient. This patient was a 65 year-old male with non-small cell lung cancer, T2N2M0 (2.2-cm-sized fluorodeoxyglucose avid mass in right lower lobe (arrow) with metastatic lymphadenopathies in right interlobar and subcarina/right paraesophageal area) (A) treated with weekly docetaxel (20 mg/m2)+cisplatin (20 mg/m2) concurrent chemoradiotherapy (CCRT). Although, he had a history of interstitial lung disease diagnosed 2 years ago, he was in a clinically stable condition with acceptable pulmonary function (forced expiratory volume in 1 second, 2.2 L; carbon monoxide lung diffusion capacity, 74%). Initial chest computed tomography showed diffuse involvement of reticular opacity, subpleural ground glass opacity (arrows) (B), traction bronchiectasis (arrow) (C), and honeycombing (arrow) (D). One month after completing CCRT (59.4 Gy/33 fractions) (E), he achieved excellent partial response. The malignant tumor in right upper lobe showed partial metabolic response (arrow) (F) and minimal haziness was noted in the computed tomography (arrow) (G). However, he developed coughing, progressive dyspnea, and fever with newly developed diffuse interstitial infiltration and consolidation extending beyond radiation field 3 months after completion of radiation therapy (H, I). No specific infectious cause was identified. He died despite 2 weeks of intensive care.
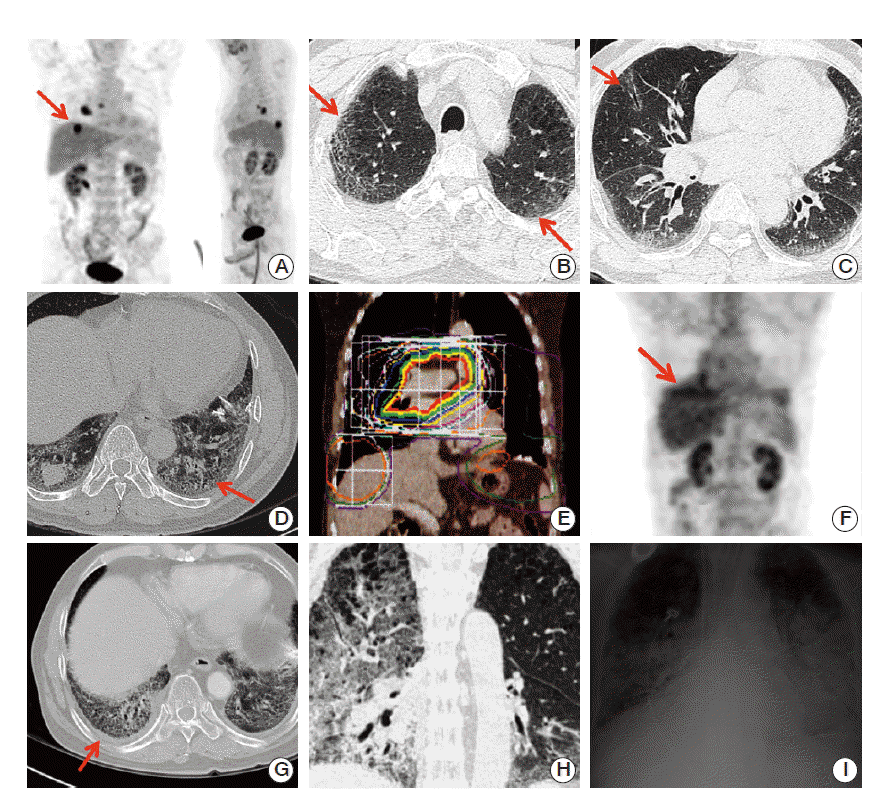
Table 1.
Patient characteristics (n=60)
Table 2.
Association between clinical factors and RP by univariable analysis
Variable | No. of RPs/Total (%) | p-valuea) | |
---|---|---|---|
Gender | Male | 19/48 (39.6) | 0.507 |
Female | 3/12 (25.0) | ||
ECOG PS | 0 | 2/4 (50) | 0.476 |
1 | 13/33 (39.4) | ||
2 | 6/15 (40.0) | ||
3 | 1/8 (12.5) | ||
Smoking | Yes | 19/46 (41.3) | 0.300 |
No | 3/12 (25.0) | ||
Weight loss | Yes | 7/21 (33.3) | 0.783 |
No | 15/39 (38.5) | ||
COPD | Yes | 8/24 (33.3) | 0.662 |
No | 14/36 (38.9) | ||
Interstitial change in CT | Yes | 9/15 (60.0) | 0.033 |
No | 13/45 (28.9) | ||
Histology | NSCLC | 14/39 (35.9) | 0.969 |
SCLC | 7/18 (38.9) | ||
Stage | I | 0/1 (0) | 0.757 |
II | 1/2 (50) | ||
III | 13/33 (39.4) | ||
IV | 0/3 (0) | ||
Tumor location | Central | 20/53 (37.7) | 0.636 |
Periphery | 2/7 (28.6) | ||
Upper | 13/39 (33.3) | 0.465 | |
Lower | 9/21 (42.9) | ||
Surgery | Yes | 5/14 (35.7) | 0.933 |
No | 17/46 (37.0) | ||
Chemotherapy | Yes | 20/48 (41.7) | 0.108 |
No | 2/12 (16.7) | ||
Sequential | 12/27 (44.4) | 0.425 | |
Concurrent | 8/21 (38.1) |
Table 3.
Association between dosimetric factors and RP by univariable analysis
Variable | Without RP | With RP | p-valuea) |
---|---|---|---|
Mean lung dose | 1,452±419 | 1,996±407 | < 0.001 |
V10 | 35.2±10.2 | 48.5±13.8 | < 0.001 |
V20 | 24.2±7.4 | 37.0±11.8 | < 0.001 |
V30 | 18.7±7.5 | 28.9±7.1 | < 0.001 |
V40 | 11.9±6.5 | 18.9±4.6 | < 0.001 |
NTCP | 7.7±16.0 | 28.9±24.6 | 0.001 |
Table 4.
Risk of RP by interstitial lung disease in pre-RT CT
Pre-RT CT finding | All patients | RP Gr ≥ 1 | RP Gr ≥ 2 | RP Gr ≥ 3 | RP Gr ≥ 4 |
---|---|---|---|---|---|
Interstitial change in CT | 15 | 9 (60) | 7 (46.7) | 6 (40) | 5 (33.3) |
No interstitial change in CT | 45 | 13 (28.9) | 7 (15.6) | 2 (4.4) | 2 (4.4) |
p-valuea) | 0.03 | 0.03 | 0.002 | 0.008 |
Table 5.
Characteristic findings in pre-RT chest computed tomography of 22 patients with RP
Table 6.
Characteristics of the five patients with grade 5 RP
RP, radiation pneumonitis; COPD, chronic obstructive pulmonary disease; RT, radiation therapy; CT, computed tomography; MLD, mean lung dose; V20 and V30, percent lung volume receiving more than 20 Gy and 30 Gy; NSCLC, non-small cell lung cancer; CCRT, concurrent chemoradiotherapy; TB, traction bronchiectasis; GGO, ground glass opacity; Chemo-RT, chemotherapy followed by radiation therapy.