Introduction
Epidermal growth factor (EGF) is a single polypeptide of 53 amino acid residues that is involved in cell growth, proliferation, and differentiation [
1]. EGF stimulates target cells by binding to epidermal growth factor receptor (EGFR), which is a transmembrane receptor with intrinsic tyrosine kinase activity. The interaction of EGF-EGFR induces dimerization and autophosphorylation of the receptor, while regulating the expression level of a variety of transcription factors through multiple signaling pathways [
2].
Contrary to the conventional concept of EGF action, treatment with EGF is sometimes associated with increased cell death. Cao et al. [
3] demonstrated that the nanomole level of EGF decreased cell adhesion and induced apoptosis in the A431 (human squamous cell carcinoma) cell line, and EGF was shown to induce apoptosis related to cell cycle arrest at the G1 check point with alterations in expression levels of regulatory proteins. In addition, EGF binding leads to the internalization of EGFR, and this structural modification is known to be a specified process of EGF-induced apoptosis [
4,
5]. More recently, studies have discussed the endocytoplasmic regulation of the EGF-EGFR complex and its association with signal transducer and activator of transcription-1 (STAT-1) and p38 mitogen-activated protein kinase (MAPK) [
6-
8].
Targeting agents of the EGFR-related signaling pathway have been proposed as a novel treatment modality [
9]. The inhibition of EGFR signaling pathways was shown to induce cytostatic and cytotoxic effects and decrease repopulation of tumor cells [
10]. Moreover, preclinical and clinical studies of the combined use of EGFR inhibitors and radiotherapy (RT) have indicated that the inhibition of EGFR enhanced therapeutic efficacy of fractionated irradiation [
11,
12]. Based on these principles, the present study focused on contradictory concepts, EGF-induced anti-tumor effect and radiosensitization. Although previous investigations reported
in vitro results of enhanced radioresponse by EGF, few studies have evaluated this
in vivo [
13-
15].
Therefore, this study was conducted to evaluate the tumor growth suppression and radiation-sensitizing effects of EGF in A431 xenograft tumor models. Potential adverse systemic effects of EGF on major normal organs were also examined.
Go to :

Materials and Methods
1. Animals
This experiment was approved by the Institutional Animal Care and Use Committee (IACUC) of Seoul National University Hospital (IACUC approval No. 12-0179). The National Research Council guidelines for the care and use of laboratory animals were observed. Male 5-week-old BALB/c-nu mice were used in this study. The animals were maintained at the Biomedical Research Institute of Seoul National University Hospital, which was approved by the Korean Food and Drug Administration and complies with the regulations and standards of the IACUC of Seoul National University Hospital. The mice were housed under pathogen-free conditions with controlled humidity (40%-60%) and temperature (20°C-24°C). Animals were housed under a 12-hour light/dark cycle, with lights on from 8 AM to 8 PM The mice were kept in an individual ventilated cage system on sawdust bedding. Standard mouse diet and filtered city tap water from standard Perspex drinking bottles were provided ad libitum.
2. Cell line
We used A431 cells that originated from human vulvar epidermoid carcinoma. The cell line was purchased from the American Type Culture Collection (Manassas, VA), and cells were maintained in Dulbecco's modified Eagle's medium (Gibco BRL, Grand Island, NY) supplemented with 10% fetal bovine serum (Gibco BRL). Cells were grown in an incubator with a humidified atmosphere of 95% air/5% CO2 at 37.5°C.
3. Study reagent
Recombinant human EGF was provided by Daewoong Pharmaceutical Company (Seoul, Korea) in the form of powder. The agent was diluted in phosphate-buffered saline at a concentration of 1 mg/mL and stored at –70°C.
4. In vivo tumor models
A total of 5×10
5 A431 cells were injected subcutaneously into the right hind leg of the mice. When the tumors reached a volume of 200 mm3 at about 7-9 days after the inoculation, mice were divided into five groups (n=8 for each group). An additional two mice (n=2) that were not inoculated with tumor cells received intraperitoneal EGF for 20 days in each experiment. The animals were monitored for 6 months without other interventions to evaluate potential adverse effects of EGF on major organs.
Fig. 1 illustrates the treatment design of the five experimental groups. The control group (group I) received no treatment, while the others received EGF for 6 days, EGF for 20 days, RT (30 Gy/6 fractions [fx], daily), and RT (30 Gy/6 fx, daily) plus concomitant EGF (for 6 days) (groups II, III, IV, and V, respectively) (
Fig. 1). EGF was administered by intraperitoneal injection (5 mg/kg) once a day. The injection dose was determined by considering the half-life of the drug, and the feasibility was examined in the preliminary experiments. RT was delivered using 6-MV photon energy (Clinac 6/100, Varian Medical Systems, Palo Alto, CA). The fraction size was 5 Gy/fx and the total RT dose was 30 Gy. A custom-made acrylic device was employed to immobilize the body and the leg tumors. In the RT plus EGF group (group V), EGF was injected several minutes before irradiation. A time interval existed due to positioning of mice on the treatment couch, opening/closing the door of the treatment room, and the beam-on time with manipulation of the treatment machine. Day 1 was defined as the start date of each treatment.
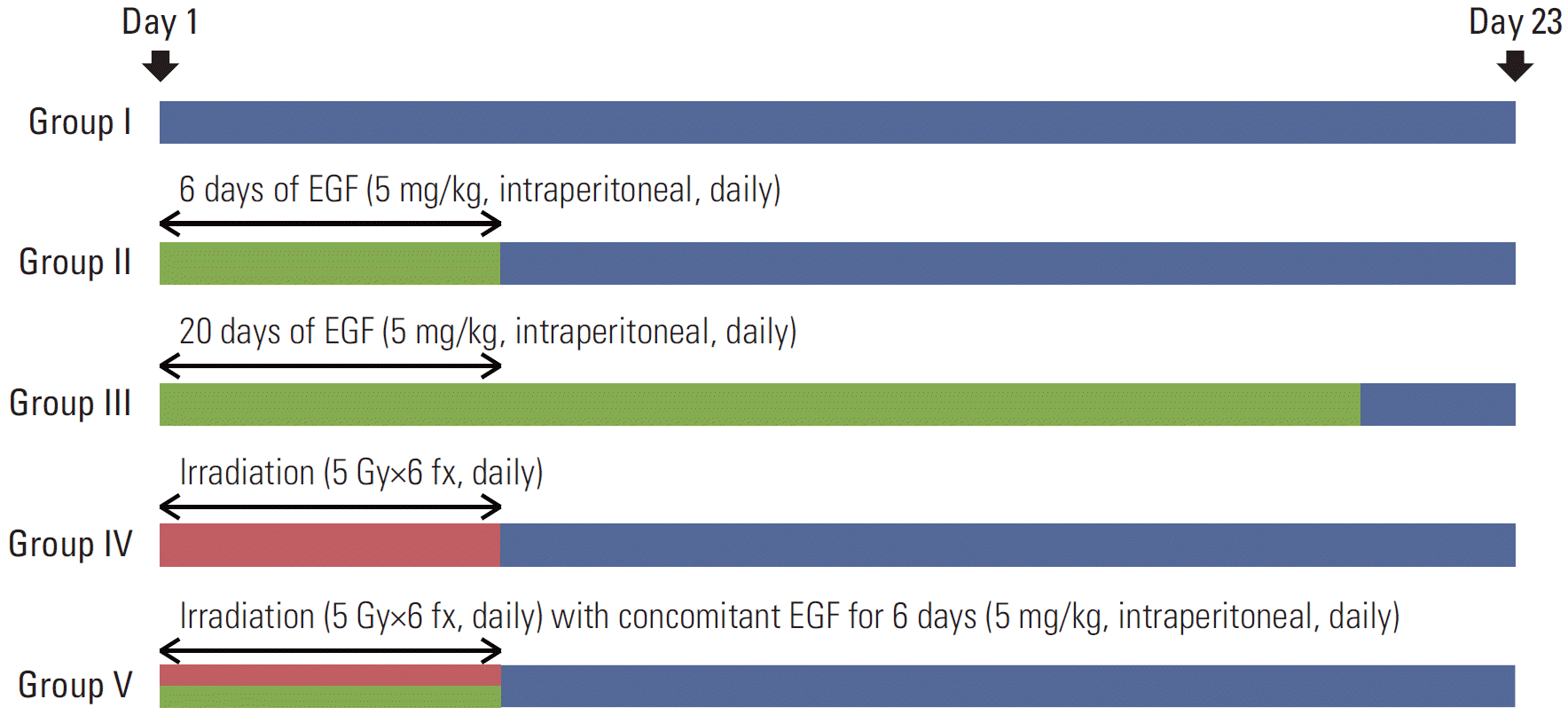 | Fig. 1.Treatment groups, dose and schedules in A431 xenograft models of nude mice. EGF, epidermal growth factor; fx, fractions. Group I, no treatment; group II, EGF for 6 days; group III, EGF for 20 days; group IV, radiotherapy; group V, radiotherapy plus concomitant EGF. 
|
5. Measurement of tumor volume
Tumor size was measured every other day using a Vernier caliper by two independent researchers (Y.J.L. and S.-R.J.) until day 23. To determine a humane endpoint, the entire period of observation was stopped before the maximum diameter of a single tumor exceeded 2 cm. Tumor volume was calculated according to the formula 1/2×length×width2 (mm3). Mice were sacrificed on days 0, 12, and 23 to obtain paraffin blocks of tumor tissues and major organs, such as liver, lung, and kidney. The relative tumor volume was defined as the ratio between the final volume and the initial volume. The experiments were independently repeated three times.
6. Hematoxylin and eosin staining and immunohistochemistry of formalin-fixed, paraffin-embedded sections
Five-micrometer-thick, paraffin-embedded tumor sections were cut and deparaffinized in Dako PT Link (Dako North America Inc., Carpinteria, CA) and then stained with hematoxylin and eosin (H&E). For immunohistochemical staining, the antigen retrieval process was performed at 97°C using target retrieval solution. Slides were rinsed with Envision FLEX Wash Buffer (Dako North America Inc.) and washed with diluted water. Endogenous blocking with 3% H2O2 was performed for 5 minutes. The primary antibodies, anti-EGFR (1:50, #4267, Cell Signaling, Danvers, MA) and anti-cleaved caspase-3 (1:50, #9661, Cell Signaling) were diluted with antibody diluents (Invitrogen Life Technologies, Carlsbad, CA). Buffer solution was used again, and secondary antibodies of horseradish peroxidase-labeled polymer anti-rabbit were applied. The samples were developed with Dako REAL 3,3'-diaminobenzidine+chromogen (Dako North America Inc.) and treated with Mayer’s hematoxylin. After multi-step dehydration with 95% and 100% alcohol, xylene was used to remove the alcohol.
7. Histologic examination
H&E-stained slides of tumor and major organ tissues of groups I-V were examined. The morphological findings were then analyzed to assess the systemic impact of exogenous EGF. Upon immunohistochemistry analysis, the expression level of EGFR in A431 cells was evaluated, and cleaved caspase-3 was used to analyze EGF-induced apoptosis in tumor tissues. A single pathologist (J.M.K.) reviewed the immunohistochemistry results without prior knowledge of treatment outcome. The intensity of expression was reported in a semi-quantitative manner.
8. Statistical analysis
Data were presented as the mean±standard deviation of the relative tumor volume. The mean data of three independent experiments were included. Since the tumor volumes were characterized as longitudinal data with repeated measurements, the differences among the experimental groups were analyzed by linear mixed model analysis. Groups I-III were analyzed together to evaluate the EGF-induced tumor growth suppression, and groups IV and V were compared to investigate enhancement of the radioresponse. The results were considered to be statistically significant if the p-value was less than 0.05. All analyses were performed using SPSS ver. 20.0 (IBM Co., Armonk, NY).
Go to :

Discussion
The anti-tumor effects of exogenous EGF have been known for decades. However, most previous investigations of these effects were based on in vitro experiments. Therefore, this study was conducted to establish EGF-induced cell death in mouse xenograft models using A431 cells and its potential as a radiation sensitizer in the design of fractionated irradiation.
The growth curve of the control group was steeper than that of groups II (EGF×6) and III (EGF×20), and the final tumor volume of untreated mice was significantly higher at the end of follow-up. However, the tumors of groups II (EGF×6) and III (EGF×20) also continued to grow, and no absolute decrease in tumor volume was observed over the entire follow-up period. The injection of exogenous EGF suppressed tumor growth, but was not clearly associated with tumor shrinkage.
Choi et al. [
17] evaluated the anti-tumor effects of EGF in xenograft mouse models. Their study compared potential cytotoxic effects of EGF (intraperitoneal, 1 mg/kg, every other day, three times) and cisplatin (intraperitoneal, 5 mg/kg, once) using subcutaneously inoculated human cancer cell lines, including A431 cells. Although the study design differed from that of the present study, the patterns of growth suppression were similar to those in the present study. The authors also demonstrated the possibility of EGF as a cytotoxic agent in certain types of tumors.
Intraperitoneal injection of EGF for 6 days did not lead to a statistically significant difference in tumor size compared to the control group, indicating that the treatment did not exert an anti-tumor effect. However, the concomitant EGF (for 6 days) with fractionated RT (30 Gy in 6 fx) resulted in more prolongation of the therapeutic effect and slower tumor re-growth relative to the RT-only group. These findings suggest that the concomitant use of EGF enhanced the radioresponse during treatment and decreased tumor repopulation after completion of RT.
The current findings are in agreement with previous
in vitro results. Kwok and Sutherland [
13] initially reported that an exogenous EGF after or continuously before, during, and after irradiation enhanced the radiosensitivity of human squamous cell carcinoma cell lines
in vitro. They demonstrated that EGF enhanced radiosensitivity with reduction in the shoulder region of the cell survival curves, and that the maximum effect was observed in response to treatment with 10 ng/mL of EGF. In addition, the EGF-related enhancement of irradiation was higher in the G1 phase with a wider shoulder region than other phases [
14,
18]. However, few studies have reported the impact of EGF on radiosensitivity to date.
Kwon et al. [
19] recently designed
in vitro and
in vivo studies to evaluate the effects of EGF on cell proliferation and radiation survival. In their study, a clonogenic assay revealed that EGF suppressed tumor growth in human cancer cell lines with high expression of EGFR, and that the inhibitory effect was more evident at higher concentrations of EGF (1 nM or more). When EGF was combined with irradiation
in vitro, EGF enhanced the cell killing effect in cancer cell lines, but not in normal fibroblast cells. In an
in vivo study of EMT-6 (mouse mammary sarcoma), treatment with EGF (1 mg/kg for 7 consecutive days, three times a day) decreased tumor volume, but this decrease was not statistically significant. The authors concluded that further studies were needed to investigate such effects in human cancer cell lines.
It should also be noted that overall growth inhibition was more evident in group III (EGF×20) than in group II (EGF×6). Although the tumor volume of mice treated with EGF for 6 days eventually approached the level of the control group, the anti-tumor effect of EGF for 20 days was maintained. Given that a longer duration of daily EGF injections induced more tumor suppression, we suggest that adequate exposure to EGF is needed to obtain an observable level of EGFinduced tumor suppression. However, since the mice treated with EGF for 20 days were sacrificed on the third day following completion of the treatment, there is a possibility of delayed tumor growth. Accordingly, further studies are needed to evaluate the differential kinetics based on total duration of EGF treatment in vivo.
Caspase-3 is one of the key molecules of the apoptosis pathway. This study provided histologic evidence of increased apoptotic cells in mice treated with RT+EGF, which was correlated with increased radioresponse and smaller tumor volumes. Song et al. [
4] performed an
in vitro study with A431 cells and found that sustained activation of EGFR by EGF was related to activation of caspase-3 and a time-dependent cleavage of caspase-8 from protease assay and western blot analysis. They concluded that EGF-mediated apoptosis could be induced by both mitochondrial and non-mitochondrial pathways. In addition, Chiu et al. [
20] conducted an
in vitro study using neuroblastoma cell lines and demonstrated that the maximum level of total caspase-3 expression could be obtained from incubation with EGF (5 ng/mL).
In the present study, the impact of EGF on major normal organs was evaluated, and the intraperitoneal injection of EGF did not induce adverse histological changes in the liver, lung, or kidney. Furthermore, extra mice treated with EGF for 20 days without tumor inoculation survived for 6 months without abnormal histological findings. Therefore, it was suggested that intraperitoneal EGF induced little systemic toxicity in the major organs studied. However, the absence of a histologic reaction does not guarantee clinical safety of the treatment because this preclinical study is based on immunosuppressed mice. Careful consideration should be given to different host factors, such as innate immunity and pharmacokinetics of intraperitoneal EGF. Potential systemic impact on other organs, including the intestine and the brain, must also be evaluated.
Several mechanisms of EGF-induced apoptosis have been proposed. In previous studies, p21-dependent arrest in the G1 phase was considered an important principle [
21,
22]. Cao et al. [
3] also reported that EGF treatment increased p21, but suppressed cyclin A and D1, cyclin-dependent kinase 2, and phosphorylated retinoblastoma protein, which induced cell cycle arrest and decline in cell adhesion. In addition, the role of STAT-1, a downstream molecule in the EGFR pathway, was substantiated [
8,
23]. More recently, several studies demonstrated that p38 MAPK mediates STAT-1 tyrosine phosphorylation, which leads to the apoptotic response of an exogenous EGF [
4,
7]. However, little is known about the potential mode of action of the radiosensitizing effect of EGF. In earlier
in vitro studies, the enhancement of radiation sensitivity was more prominent in cancer cells with higher EGFR expression [
14]. Furthermore, cell-cycle dependency was observed, and the G1 phase was found to be most responsive [
18].
Go to :
