Abstract
Purpose
The purpose of this study was to determine the optimal biologically equivalent dose (BED) for stereotactic body radiotherapy (SBRT) by comparing local control rates in proportion to various total doses and fractionation schedules.
Materials and Methods
Thirty-four patients with early non-small-cell lung cancer and a single metastatic lung tumor were included in this study. Differences in local control rates were evaluated according to gender, primary tumor site, response, tumor size, and BED. For comparison of BEDs, the prescribed dose for SBRT was stratified according to three groups: high (BED > 146 Gy), medium to high (BED, 106 to 146 Gy), and low to medium (BED < 106 Gy).
Results
For all patients, the overall local control rate was 85.3% at two years after treatment. Five local recurrences were observed, and, notably, all of them were observed in the low to medium BED group. Significantly higher local control rates were observed for patients with a complete response than for those with a partial response or stable disease (p < 0.001). Twenty-six patients with a tumor size of < 3 cm showed no dose-response relationship in the low to medium, medium to high, and high BED groups, whereas eight patients with a tumor size of ≥ 3 cm showed a significant dose-response relationship. The observed 2-year local recurrence-free survival rates in patients with a tumor size of < 3 cm and in those with a tumor size of ≥ 3 cm were 96.2% and 50.0%, respectively, which were significantly different (p=0.007).
Conclusion
BED > 100 Gy is required in order to achieve a > 85% local control rate regardless of tumor size. The optimal dose for small tumors of < 3 cm appears to be within a range below 150 Gy BED. Escalation of BED to high levels (> 150 Gy) may be required for patients with a tumor size larger than 3 cm.
Stereotactic body radiotherapy (SBRT) plays an important role in treatment of patients with early primary and metastatic lung tumors because its clinical outcomes have been proven to be equivalent to those of surgical resection [1-3]. In addition, recent technological advances in image guidance and treatment delivery techniques have enabled more precise delivery of high dose radiation to tumors [4].
Previously, the recommended dose for SBRT was 48-60 Gy in 3-5 fractions within a short time interval [5,6]. For improvement of local tumor control, dose escalation for SBRT has become an important issue. However, published studies on SBRT vary considerably with regard to the fractionation schedules and total dose to target volume [5,6]. According to the Elekta Collaborative Lung Research Group, the biologically equivalent dose (BED) is the most significant predictor of local control as calculated using an α/β of 10 Gy in the linear quadratic model [7]. The large multi-institutional retrospective review conducted by Onishi et al. [8] demonstrated that a BED of 100 Gy or more resulted in superior local control and survival rates than did BEDs of less than 100 Gy. In a phase II trial of SBRT for T1 lung cancer of Radiation Therapy Oncology Group (RTOG) 0236, delivery of 151 Gy BED resulted in a 3-year local control rate of 100% [9]. Although the consensus on the dose for SBRT is shifting in favor of a high BED of > 100 Gy, Zhang et al. [10] reported that a high BED of > 146 Gy (20 Gy×3) for ablation of small metastatic or primary lung nodules might exceed the optimal dose. Thus, the reported optimal doses and fractionation schedules of SBRT for treatment of early primary and metastatic lung tumors are still inconsistent. Therefore, the purpose of this study was to determine the optimal BED for SBRT by comparing local control rates in proportion to various total doses and fractionation schedules.
Thirty-four patients with early non-small-cell lung cancer or a single metastatic lung tumor who were treated between July 2010 and January 2013, were included in this study after institutional review board approval of our institutional protocol.
The patient's body was immobilized with a vacuum. Multislice respiration-correlated computed tomography (4DCT) scans were acquired for SBRT planning using the Varian Real-time Position Management (RPM) system (Varian Medical Systems Inc., Palo Alto, CA) to monitor patient breathing without administration of intravenous contrast medium.
The acquired images were sorted using the Treatment Planning System (TPS; Eclipse v.8.6, Varian Medical Systems Inc.) according to respiratory phases that were determined using the RPM, to generate the 10-breathing phase (0-90%) 4DCT image dataset. Gross tumor volume (GTV) was delineated as the visible tumor in each phase of the lung window on the 4DCT images, which accounted for all tumor positions in the four-dimensional dataset. The clinical target volume was identical to the GTV on the assumption that microscopic disease is controlled by doses delivered along the 30-40% isodose line. The summation of tumor positions during all phases of respiration was used to generate an individualized internal target volume for each tumor, to which a margin of 2 mm was uniformly expanded to create the planning target volume (PTV) to account for setup uncertainties. Positioning of the immobilized patient and target localization were verified before every treatment session using onboard cone beam computed tomography (CT) (Varian iX, Varian Medical Systems Inc.). SBRT planning was performed using TPS software.
SBRT was delivered with total doses of 36-60 Gy in 3-5 fractions within a total treatment time of 8-15 days. Total doses and fractionation schedules were chosen according to the size and location of the tumors adjacent to normal tissues. These doses were prescribed to the 95% isodose line covering the PTV.
Differences in local control rates were evaluated according to gender, primary tumor site, response, tumor size, and BED. For comparison of BEDs, the prescribed doses were administered according to various fractionation schedules for SBRT and were stratified into three groups according to the corresponding BED: high (BED > 146 Gy), medium to high (BED, 106 to 146 Gy), and low to medium (BED < 106 Gy). The efficacy of different fractionation and total doses for local control of the tumor was then analyzed. We applied the BED calculation formula nd (1+d/α/β) to each of our dose schedules, where n=the fractionation number, d=the daily dose, and α/β was assumed to be 10 Gy for tumors. For example, the BED for 20 Gy×3 treatments is 150 Gy and places the patient in the group in which patients received a high BED. The median BED for SBRT was 93.5 Gy (range, 66 to 150 Gy). The response was first evaluated by chest CT taken at 1 to 2 months after SBRT. Thereafter, patients were followed up with either chest CT or positron emission tomography (PET)-CT at 3 to 4 month intervals.
Local response was categorized according to three types: 1) complete response (CR): complete disappearance of the tumor on a CT or PET scan, 2) partial response (PR): reduction of at least 30% in the sum of the longest diameter of the tumor, and 3) stable disease (SD): a response of less than 30% decrease in the sum of the longest diameter of the tumor [1]. Distinguishing between tumor progression and radiation-induced lung consolidation was often difficult; therefore, local recurrence was assigned when the tumor size had increased on two consecutive CT scans [11].
Categorical variables were compared using the chi-square test or Fisher's exact test. Continuous variables were compared using the Mann-Whitney test, Student t-test, Kruskal-Wallis test, and one-way analysis of variance. Median overall local control rate and local recurrence-free survival curves were calculated using the Kaplan-Meier method. For comparison of the local control rates among groups, the log-rank test was used for calculation of p-value. SPSS ver. 20.0 (IBM Corp., Armonk, NY) was used in performance of statistical analyses. All tests were two-tailed and a p-value of < 0.05 was considered statistically significant.
Patient characteristics are shown in Table 1. The primary organs involved were the lung (n=19), head and neck (n=6), breast (n=5), kidney (n=2), rectal (n=1), and bone (n=1). The median tumor size was 1.6 cm (range, 0.7 to 5.0 cm). Twenty-six patients (76.5%) had a tumor size of < 3 cm, whereas eight patients (23.5%) had a tumor size of ≥ 3 cm. All tumors were peripherally located in the lung. In 16 cases, lesions were located in the upper lobe of the lungs; in 16 cases in the lower lobe; and in two cases in the right middle lobe. Of the 34 patients, nine received high doses of radiation for SBRT (BED > 146 Gy), four received medium to high doses (BED, 106 to 146 Gy), and 21 received low to medium doses (BED < 106 Gy).
At two years after treatment, the overall local control rate for all patients was 85.3%. Five local recurrences were observed with an average time to local recurrence of 14.6 months (range, 6.1 to 34.4 months). Of the five local recurrences, four were noted in cases of primary lung tumors and one was noted in a head and neck primary tumor (Table 2).
Differences in local control were evaluated according to response, tumor size, BED, gender, and primary tumor site. CR was noted in 28 patients, PR was noted in five patients, and SD was noted in one patient. Significantly greater local control rate was observed for patients with CR than for those with PR or SD (p < 0.001). In contrast to the four of the eight patients (50%) with a tumor size of ≥ 3 cm who showed local recurrence, only one of 26 patients (3.9%) with a tumor size of < 3 cm showed local recurrence (p=0.007). Local control rates at two years for the high and medium to high BED groups were 100%, whereas the rate was 76.2% for low to medium BED group (p=0.076). The incidence of local recurrence did not vary significantly according to primary site (p=0.536) and gender (p=0.150) (Table 2).
Table 3 shows the characteristics of the three BED groups. The median follow-up periods were 17.4 months (range, 5.2 to 27.5 months), 15.2 months (range, 6.3 to 28.1 months), and 14.6 months (range, 6.1 to 34.4 months) in the high, medium to high, and low to medium BED groups, respectively. All five local recurrences were noted in the low to medium BED group (p=0.076).
BEDs in our data set were analyzed for possible association between local recurrence and tumor size. No dose-response relationship for 26 patients with a tumor size of < 3 cm showed in the low to medium, medium to high, and high BED groups, whereas a significant dose response relationship was observed for patients with a tumor size of ≥ 3 cm in all 3 BED groups. BED > 100 Gy is necessary in order to achieve a > 85% local control rate regardless of tumor size (Table 4). Individual characteristics of patients who manifested local recurrence are shown in Table 5.
Fig. 1 shows the Kaplan-Meier curves of local recurrence-free survival for each of the three BED groups. The 2-year local recurrence-free survival rates in the high, medium to high, and low to medium BED groups were 100%, 100%, and 76.2%, respectively. Results of the log rank test did not show a statistically significant difference among the three groups (p=0.583). In stratification according to tumor size, patients with tumors < 3 cm, and those with tumors ≥ 3 cm had 2-year local recurrence-free survival rates of 96.2% and 50.0%, respectively (p=0.099) (Fig. 2). Fig. 3 shows the local recurrence-free survival among different response groups. The 2-year estimated median time to recurrence was 29.4 months for patients in the SD group and 27.8 months for those in the PR group (p=0.255). Univariate and multivariate analyses were performed to determine prognostic factors for local recurrence, however, no significant factors were identified (Table 6).
The applicability of BED employing a large dose per fraction is criticized by the likelihood of overestimating the BED [12]. Nevertheless, we used the linear-quadratic model with an α/β of 10 Gy for calculation of BED and then compared it to the existing reports that used a variety of SBRT doses and fractionation schedules.
Several groups have investigated the dose-response relationship for local tumor control. Two large series of multi-institutional reviews by Onishi et al. [8] and Baumann et al. [13] demonstrated that using higher BED on local tumors resulted in significantly better outcomes. Onishi et al. [8], who used BED calculations for comparison of a wide variety of SBRT dose and fractionation schedules, found improved treatment efficacy for a BED of > 100 Gy. The local recurrence rate was just 8.4% in patients who received a BED of ≥ 100 Gy, which was lower than the 42.9% local recurrence rate in patients receiving a BED of < 100 Gy. Onimaru et al. [14] compared two dose regimens of 40 Gy and 48 Gy in four fractions administered to patients with stage I non-small cell lung carcinoma (NSCLC). In patients with stage IB disease, significantly better local control and cause-specific survival was observed with the 48-Gy regimen than with the 40 Gy regimen. In a recent study, Mehta et al. [15] also confirmed that achieving an acceptable local control of > 90% would require a BED that was substantially above 100 Gy. In our study, all five local recurrences were observed in patients who received doses below a BED of 100 Gy, which corresponds to 45 Gy at 15 Gy per fraction. The group treated with low to medium BED (66 to 106 Gy) had an increased risk of local recurrence, whereas patients in both the medium to high (106 to 146 Gy) and high (> 146 Gy) BED groups showed equal local control rates of 100% at two years after treatment. Although, no significant increase of local recurrence was observed in the low to medium BED group (p=0.076) compared to the medium to high and high BED groups, our results are consistent with previous reports of improved outcomes for a BED of > 100 Gy [8,13,15,16].
While a minimal BED necessary for SBRT in order to achieve a > 90% local control rate is generally agreed to be > 100 Gy, an optimal maximum BED, based on dose-response relationships and tumor size, has not been identified. Zhang et al. [10] reported that using a high BED of > 146 Gy (20 Gy×3) for ablation of small sized metastatic or primary lung nodules might have exceeded the optimal dose. In this study, the medium (83.2 to 106 Gy) or medium to high (106 to 146 Gy) BED groups had a higher overall survival than those treated with low (< 83.2 Gy) or high (> 146 Gy) BED. Subgroup analysis showed that medium BED might be reasonable for stage I NSCLC. According to van Baardwijk et al. [5], published results in the literature do not support the hypothesis of a positive dose-response relationship for tumor control within the applied dose range. They suggested that doses should be reduced to a level that still achieves tumor control rates in excess of 90% for stage I NSCLC. Mehta et al. [15] also reported that dose-escalation beyond a BED of 159 Gy, as calculated using a linear quadratic model, likely translates to a clinically insignificant gain in tumor control probability but may result in clinically significant toxicity. Therefore, a dose of 60 to 66 Gy delivered in three fractions (BED, 150 to 180 Gy) for T1 lung cancer used by initial studies from North America [9] including RTOG 0236 [17] may be unnecessarily high. In our subgroup analysis on the relationship between BED and tumor size, no significant dose-response relationship in local recurrence was observed among the low to medium, medium to high, and high BED groups in patients with a tumor size of < 3 cm (Table 4). Consequently, we suggest that the medium to high and high BED regimens for ablation of tumors < 3 cm shows equivalent local control and that the optimal dose for small tumors < 3 cm may be within a range below 150 Gy BED, although these findings should be interpreted with caution due to the small sample size and few local recurrences in our data set.
Tumor size is a significant prognostic factor in treatment of lung cancer [18]. In the Nordic SBRT trial [19], the recurrence rate among T1a patients was 0% at three years, which was significantly better than the rate of 40.8% for patients with T2a disease. Matsuo et al. [20] also reported that with dosage of 48 Gy in four fractions, a tumor size of 20 mm was a more optimal threshold than a tumor size of 30 mm for prognostic assessment after SBRT. They demonstrated that tumor diameter is one of the most significant factors affecting outcome after SBRT. In our analysis, the local control rate decreased significantly with an increase in tumor size (p=0.007) (Table 2). Patients with a tumor size of < 3 cm had a local control rate of 96.2% at two years whereas those with a tumor size ≥ 3 cm had an inferior 2-year local control rate of 50%.
Ohri et al. [18] reported that the effect of tumor size on predicted outcome diminishes as BED increases. For example, 50 Gy in five fractions on tumors of sizes 1 cm, 3 cm, and 5 cm results in predicted 2-year local control rates of 93%, 90%, and 83% respectively. When a more aggressive regimen is used, such as 54 Gy in three fractions, those predicted control rates increase to 99%, 98%, and 96% respectively. Similarly, a review of published experiences suggests that the influence of tumor size on local control seems to decrease with the intensity of the SBRT regimen used [7,9,21,22]. In a study where 45 Gy in three fractions was delivered to the isocenter (PTV BED, 95 Gy), the 3-year rate of local control was 78% for T1 lesions and only 40% for T2 lesions [21]. In RTOG 0236 where a BED of 151 Gy was delivered to the PTV, the 3-year local control rate was 91% for T2 lesions [17]. Our study demonstrated significantly improved local control in patients with tumors larger than 3 cm who were administered a higher BED (p=0.049) (Table 4). Based on previous reports and current study, escalation of the local BED to high levels (> 150 Gy) may be required for patients with a tumor size of ≥ 3 cm. The ideal treatment regimen for large sized tumors remains to be determined in future studies.
In our study, the inclusion of diverse clinical disease entities of primary lung lesions and metastatic lung lesions from various origins in our analysis of dose response and local control is based on known outcomes in which local control rates do not vary with tumor origin if the appropriate dose for SBRT is employed [1,2]. Some authors, however, reported that local control rates after SBRT for metastatic lung tumors differed according to the origin of the primary tumor [23,24]. Local control rates for metastatic lung tumors from colorectal cancer are significantly worse than those for primary lung cancers or metastasis from cancers of other origins. However, the causes for worse outcomes appear to be attributed to sub-optimal dose prescription rather than primary tumor origin. This is because the dose for previously reported metastatic lung tumors from colorectal cancer was lower than 100 Gy of BED, which is the sub-optimal dose for a local control rate of > 90%. Norihisa et al. [1] reported that no local progression was observed in nine cases of oligometastatic lung tumors of colorectal origin that were irradiated with 60 Gy in 4 or 5 fractions (BED, 110 to 125 Gy).
Late local recurrence after SBRT has been reported [25]. However, in our study we were unable to determine local control at time points beyond two years because of the short follow-up time. Thus, conduct of additional studies of long-term outcomes is warranted.
BED >100 Gy is required in order to achieve a > 85% local control rate regardless of tumor size. The optimal dose for small tumors of < 3 cm appears to be in a range below 150 Gy BED. Escalation of BED to high levels (> 150 Gy) may be required for patients with a tumor size larger than 3 cm. Conduct of future studies will be needed in order to determine the ideal BED under these circumstances in addition to the impact of tumor size on optimal BED so that treatment may be tailored for the individual patient.
References
1. Norihisa Y, Nagata Y, Takayama K, Matsuo Y, Sakamoto T, Sakamoto M, et al. Stereotactic body radiotherapy for oligometastatic lung tumors. Int J Radiat Oncol Biol Phys. 2008; 72:398–403.


2. Wulf J, Haedinger U, Oppitz U, Thiele W, Mueller G, Flentje M. Stereotactic radiotherapy for primary lung cancer and pulmonary metastases: a noninvasive treatment approach in medically inoperable patients. Int J Radiat Oncol Biol Phys. 2004; 60:186–96.


3. Chi A, Liao Z, Nguyen NP, Xu J, Stea B, Komaki R. Systemic review of the patterns of failure following stereotactic body radiation therapy in early-stage non-small-cell lung cancer: clinical implications. Radiother Oncol. 2010; 94:1–11.


4. Underberg RW, Lagerwaard FJ, Cuijpers JP, Slotman BJ, van Sornsen de Koste JR, Senan S. Four-dimensional CT scans for treatment planning in stereotactic radiotherapy for stage I lung cancer. Int J Radiat Oncol Biol Phys. 2004; 60:1283–90.


5. Van Baardwijk A, Tome WA, van Elmpt W, Bentzen SM, Reymen B, Wanders R, et al. Is high-dose stereotactic body radiotherapy (SBRT) for stage I non-small cell lung cancer (NSCLC) overkill? A systematic review. Radiother Oncol. 2012; 105:145–9.


6. Olsen JR, Robinson CG, El Naqa I, Creach KM, Drzymala RE, Bloch C, et al. Dose-response for stereotactic body radiotherapy in early-stage non-small-cell lung cancer. Int J Radiat Oncol Biol Phys. 2011; 81:e299–303.


7. Kestin LL, Grills IS, Guckenberger M, Belderbos J, Hope AJ, Werner-Wasik M, et al. Substantial dose-response relationship with clinical outcome for lung stereotactic body radiotherapy (SBRT) delivered via online image guidance. Int J Radiat Oncol Biol Phys. 2010; 78 Suppl:S14.


8. Onishi H, Shirato H, Nagata Y, Hiraoka M, Fujino M, Gomi K, et al. Hypofractionated stereotactic radiotherapy (Hypo-FXSRT) for stage I non-small cell lung cancer: updated results of 257 patients in a Japanese multi-institutional study. J Thorac Oncol. 2007; 2(7 Suppl 3):S94–100.


9. Timmerman R, Paulus R, Galvin J, Michalski J, Straube W, Bradley J, et al. Stereotactic body radiation therapy for inoperable early stage lung cancer. JAMA. 2010; 303:1070–6.


10. Zhang J, Yang F, Li B, Li H, Liu J, Huang W, et al. Which is the optimal biologically effective dose of stereotactic body radiotherapy for Stage I non-small-cell lung cancer? A meta-analysis. Int J Radiat Oncol Biol Phys. 2011; 81:e305–16.


11. Aoki T, Nagata Y, Negoro Y, Takayama K, Mizowaki T, Kokubo M, et al. Evaluation of lung injury after threedimensional conformal stereotactic radiation therapy for solitary lung tumors: CT appearance. Radiology. 2004; 230:101–8.


12. Park C, Papiez L, Zhang S, Story M, Timmerman RD. Universal survival curve and single fraction equivalent dose: useful tools in understanding potency of ablative radiotherapy. Int J Radiat Oncol Biol Phys. 2008; 70:847–52.


13. Baumann P, Nyman J, Lax I, Friesland S, Hoyer M, Rehn Ericsson S, et al. Factors important for efficacy of stereotactic body radiotherapy of medically inoperable stage I lung cancer: a retrospective analysis of patients treated in the Nordic countries. Acta Oncol. 2006; 45:787–95.


14. Onimaru R, Fujino M, Yamazaki K, Onodera Y, Taguchi H, Katoh N, et al. Steep dose-response relationship for stage I non-small-cell lung cancer using hypofractionated high-dose irradiation by real-time tumor-tracking radiotherapy. Int J Radiat Oncol Biol Phys. 2008; 70:374–81.


15. Mehta N, King CR, Agazaryan N, Steinberg M, Hua A, Lee P. Stereotactic body radiation therapy and 3-dimensional conformal radiotherapy for stage I non-small cell lung cancer: a pooled analysis of biological equivalent dose and local control. Pract Radiat Oncol. 2012; 2:288–95.


16. Guckenberger M, Wulf J, Mueller G, Krieger T, Baier K, Gabor M, et al. Dose-response relationship for image-guided stereotactic body radiotherapy of pulmonary tumors: relevance of 4D dose calculation. Int J Radiat Oncol Biol Phys. 2009; 74:47–54.


17. Dunlap NE, Larner JM, Read PW, Kozower BD, Lau CL, Sheng K, et al. Size matters: a comparison of T1 and T2 peripheral non-small-cell lung cancers treated with stereotactic body radiation therapy (SBRT). J Thorac Cardiovasc Surg. 2010; 140:583–9.


18. Ohri N, Werner-Wasik M, Grills IS, Belderbos J, Hope A, Yan D, et al. Modeling local control after hypofractionated stereotactic body radiation therapy for stage I non-small cell lung cancer: a report from the elekta collaborative lung research group. Int J Radiat Oncol Biol Phys. 2012; 84:e379–84.


19. Baumann P, Nyman J, Hoyer M, Wennberg B, Gagliardi G, Lax I, et al. Outcome in a prospective phase II trial of medically inoperable stage I non-small-cell lung cancer patients treated with stereotactic body radiotherapy. J Clin Oncol. 2009; 27:3290–6.


20. Matsuo Y, Shibuya K, Nagata Y, Takayama K, Norihisa Y, Mizowaki T, et al. Prognostic factors in stereotactic body radiotherapy for non-small-cell lung cancer. Int J Radiat Oncol Biol Phys. 2011; 79:1104–11.


21. Koto M, Takai Y, Ogawa Y, Matsushita H, Takeda K, Takahashi C, et al. A phase II study on stereotactic body radiotherapy for stage I non-small cell lung cancer. Radiother Oncol. 2007; 85:429–34.


22. Baba F, Shibamoto Y, Ogino H, Murata R, Sugie C, Iwata H, et al. Clinical outcomes of stereotactic body radiotherapy for stage I non-small cell lung cancer using different doses depending on tumor size. Radiat Oncol. 2010; 5:81.


23. Takeda A, Kunieda E, Ohashi T, Aoki Y, Koike N, Takeda T. Stereotactic body radiotherapy (SBRT) for oligometastatic lung tumors from colorectal cancer and other primary cancers in comparison with primary lung cancer. Radiother Oncol. 2011; 101:255–9.


Fig. 1.
Kaplan-Meier local recurrence-free survival curves according to biologically equivalent dose groups (p=0.583).
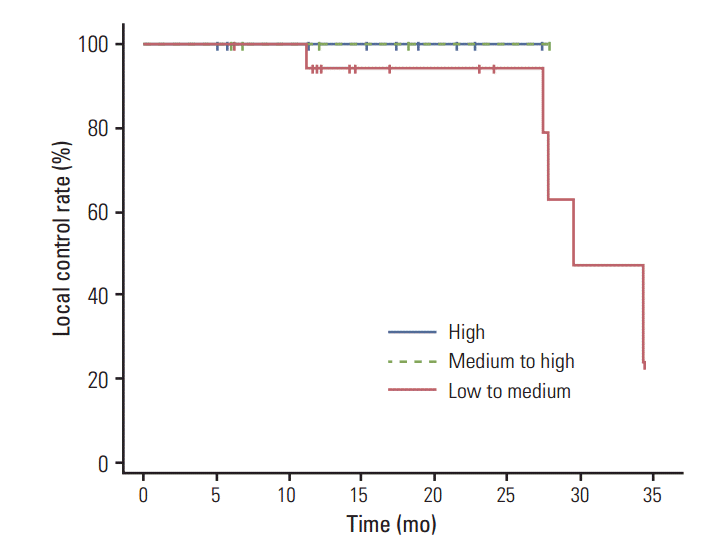
Table 1.
Patient and treatment characteristics
Table 2.
Analysis of factors affecting local control rate
Variable | Local recurrence | Local control | Crude 2-year local control rate (%) | p-value |
---|---|---|---|---|
Gender | 0.150a) | |||
Male | 5 | 18 | 78.3 | |
Female | 0 | 11 | 100 | |
Primary tumor | 0.536 | |||
Lung | 4 | 15 | 79.0 | |
Head and neck | 1 | 5 | 83.3 | |
Breast | 0 | 5 | 100 | |
Others | 0 | 4 | 100 | |
Response | < 0.001a) | |||
CR | 1 | 27 | 96.4 | |
PR | 3 | 2 | 40.0 | |
SD | 1 | 0 | 0 | |
Size of tumor (cm) | 0.007a) | |||
< 3 | 1 | 25 | 96.2 | |
≥ 3 | 4 | 4 | 50.0 | |
BED | 0.076a) | |||
High | 0 | 9 | 100 | |
Medium to high | 0 | 4 | 100 | |
Low to medium | 5 | 16 | 76.2 |
Table 3.
Characteristics of three BED groups
Table 4.
Relationship of local recurrence between BEDs and tumor sizes
Table 5.
Characteristics of local recurrent patients
Table 6.
Prognostic factors related to local recurrence
Prognostic factor | Univariate p-value | Multivariate p-valuea) |
---|---|---|
Gender | 0.271 | 0.749 |
Age | 0.072 | 0.824 |
Primary site | 0.264 | 0.722 |
Response | 0.135 | 0.983 |
Tumor size | 0.135 | 0.983 |
BED | 0.082 | 0.987 |