Abstract
Purpose
This study was undertaken to evaluate the significance of cyclooxygenase-2 (COX2) overexpression and the expression of somatostatin receptor (SSTR) subtypes in gastroenteropancreatic neuroendocrine tumors (GEP-NETs).
Materials and Methods
Two hundred and forty-seven cases of GEP-NET, comprising 86 foregut and 156 hindgut primary NETs, and 5 metastatic NETs in the liver, were studied retrospectively with immunohistochemistry for COX2, chromogranin A, Ki-67, SSTR1, SSTR2, and SSTR5.
Results
COX2 overexpression was observed in 54%(126 of 234), and SSTR1, SSTR2, and SSTR5 positivity in 84%(196 of 233), 72%(168 of 233), and 55%(128 of 232), respectively. COX2 overexpression was found to be positively correlated with Ki-67 labeling index and inversely correlated with the expression of SSTR subtypes. In addition, the expression of SSTR subtypes was tightly correlated in any comparative pairs. A significant inverse correlation was found between COX2 and SSTR2 expression in the foregut, but not hindgut NETs. Kaplan-Meier analyses showed that COX2 overexpression (p=0.003) and high Ki-67 labeling index (p<0.001) were associated with poor overall survival (OS), whereas expression of SSTR2 (p<0.001) was associated with better OS of GEP-NET patients. Multivariate analysis revealed negative SSTR2 expression as an independent prognostic marker in GEP-NET patients (p<0.001).
Gastroenteropancreatic neuroendocrine tumors (GEP-NETs) constitute a heterogeneous group of tumors that originate from the neuroendocrine cell system, have widely divergent clinical presentations and are relatively infrequent, constituting only approximately 2% of all neoplasms; they are typically indolent, slow-growing tumors [1]. GEP-NETs occur in approximately 1.95-2.50 per 100,000 population and pancreatic endocrine tumors occur in approximately 1 per 100,000 population and represent 1-2% of all pancreatic neoplasms [2].
Cyclooxygenase-2 (COX2) is expressed in neuroendocrine cells of human colonic mucosa [3], and in cell lines derived from NETs, including metastatic carcinoids of the pancreas. The neuroendocrine tumor cells express COX2, and furthermore, their growth is inhibited by non-steroidal anti-inflammatory drugs (non-steroidal anti-inflammatory drugs or COX2 selective inhibitors) [4]. A few reports have found that COX2 expression was associated with poor prognosis of midgut carcinoid tumors or reported high COX2 expression in the advancing edge of gastrointestinal carcinoid tumors [5]. Ohike and Morohoshi [6] reported that the distribution of COX2-positive cells tended to be similar to the distribution of Ki-67-positive cells in pancreatic NETs, and that there was a close relationship between COX2 expression and tumor proliferative activity.
The small cyclic peptide hormone somatostatin is present in the human body in 2 molecular forms, i.e., somatostatin-14 (consisting of 14 amino acids) and somatostatin-28 (28 amino acids). It exerts diverse biologic effects through interaction with specific somatostatin receptors (SSTRs) located in target tissues. SSTRs are a family of 5 widely distributed G protein-coupled receptors, including subtypes SSTR1, SSTR2, SSTR3, SSTR4, and SSTR5, which characteristically consist of a single polypeptide chain with 7 transmembrane-spanning domains, extracellular domains with ligand binding sites and intracellular domains with sites linked to the activation of second messengers. SSTRs mediate different intracellular signaling pathways involved in cell proliferation, differentiation, and angiogenesis [7].
The inhibitory effects of either somatostatin or its analogs, mediated via SSTRs, are linked with several intracellular systems, i.e., inhibition of adenylyl cyclase, resulting in a decrease in intracellular cyclic AMP levels; reduction of Ca2+ influxes, resulting in reduced intracellular Ca2+ levels; and, in a number of tissues, stimulation of tyrosine phosphatase activity. Long-acting somatostatin analogs have been used for more than 10 years to treat endocrine tumors. Their high receptor-binding affinity is the basis for both diagnostic uses, like scintigraphy or positron emission tomography scans, and therapeutic procedures [8]. A large variety of primary tumors and their metastases can express a high density of SSTRs. Pituitary tumors, endocrine pancreatic tumors and carcinoids express multiple SSTR subtypes, but SSTR2 predominance was found in 90% of carcinoids and 80% of endocrine pancreatic tumors. It has been recently shown that treatment of non-functioning pancreatic endocrine tumor with the somatostatin analog octreotide can stabilize the disease in approximately 40% of cases [9,10].
To study the clinical significance of the expression of COX2 and SSTR subtypes in GEP-NETs, we analyzed immunostaining findings for COX2 and SSTR subtypes in 247 patients with GEP-NET.
The formalin-fixed and paraffin-embedded tumor tissues of patients treated from 1989 to 2008 were retrieved from the archives at the Department of Pathology at Seoul National University Hospitals in Seoul and Bundang, Korea. The inclusion criteria were primary or metastatic GEP-NET diagnosed by endoscopic biopsy and surgical resection, and the exclusion criteria were neuroendocrine tumor of appendiceal origin or metastatic neuroendocrine tumor of unknown primary origin.
Two hundred and forty-seven patients (mean age, 53 years; range, 21 to 88 years) were included in this study. Among them, follow-up and survival information was obtained for 194 patients. The mean follow-up period was 44 months (range, 1 to 140 months), as of September 2010. The study protocol was approved by the Institutional Review Board committee of Seoul National University Hospital (C-1012-027-343). Patient survival data, including dates and causes of death, were obtained from the Korean Central Cancer Registry at the Ministry of Health and Welfare. Standard histopathological examinations included assessment of the pathological tumor stage, according to the criteria described in the 6th edition of the American Joint Committee on Cancer staging manual [11]. Histologic classifications were performed as recommended by the World Health Organization (WHO) guidelines [12].
Tissues obtained from patients were routinely fixed in 10% buffered formalin and embedded in paraffin blocks. After screening the available slides for each case, we selected a paraffin block that was well fixed and contained a representative section of the tumor. One tissue column (2.0 mm in diameter) was obtained from each selected paraffin block and arranged in separate new paraffin blocks with 60 holes, using a trephine apparatus (Superbiochips Laboratories, Seoul, Korea). Thus, tissue array blocks containing tumor tissues (2 mm in diameter) were produced from all samples. Microarray blocks were then sectioned at 4 µm and processed for immunohistochemical staining. After removing paraffin with xylene, the sections were rehydrated with graded ethanol and immersed in Tris-buffered saline.
Immunostainings for COX2, Ki-67, chromogranin A (CgA), SSTR1, SSTR2, and SSTR5 were performed using Bond Polymer Refine Detection kits (Leica Microsytems, Newcastle Upon Tyne, UK) and Leica BOND-MAX autostainer (Leica Microsystems) with the following antibodies: COX2 (1 : 100, CX229, mouse monoclonal, Cayman Chemical, Ann Arbor, MI), Ki-67 (1 : 100, MIB-1, mouse monoclonal, DAKO, Carpentaria, CA), CgA (1 : 100, M0869, mouse monoclonal, DAKO), SSTR1 (1 : 100, ab2366, rabbit polyclonal, Abcam, Cambridge, UK), SSTR2 (1 : 100, MAB4224, mouse monoclonal, R&D systems, Minneapolis, MN), and SSTR5 (1 : 100, PAB0299, rabbit polyclonal, Abnova, Jhongli, Taoyuan, Taiwan), respectively.
Immunoreactivity for COX2 was evaluated in tumor cells using a semi-quantitative system, based on the sum of staining intensity (0=negative staining, 1=weak, 2=intermediate, 3=strong staining) and positive cell percentage scores (0=0%, 1=1-25%, 2=26-50%, 3≥50%). Thus, scores from 0-3 assigned for staining intensity and percentage scores were added to obtain an overall score (maximum 6). With regard to COX2 staining, scores of 0-4 (negative or low expression) were considered negative and scores of 5-6 (high expression) as positive [5,13]. For CgA, samples displaying any nuclear staining in tumor cells were scored as positive; for SSTR1, samples showing any cytoplasm or nuclear staining were scored as positive; for SSTR2, samples showing membranous staining in >50% of tumor cells with moderate staining were scored as positive; finally, for SSTR5, samples displaying cytoplasmic staining in >50% tumor cells were scored as positive. Ki-67 staining was performed in all 247 patients on tissue array and grade was obtained in 232 patients. The number of cells that stained for Ki-67 were expressed as percentages; thus, the Ki-67 labeling index was graded as G1 if the number of positive cells was ≤2%, G2 when 3-20%, and G3 when >20%.
Spearman's rank correlation was performed for analyses of 6 protein expressions in GEP-NETs. Survival rates were calculated using the Kaplan-Meier method, and groups were compared using the log rank test. Kaplan-Meier curves were plotted using overall survival (OS) data. p-values <0.05 were considered statistically significant. Multivariate analyses were conducted using the Cox proportional hazard regression model. Factors included in the multivariate analysis for OS were pathologic stage (confined within organ vs. advanced stage), histology (well-differentiated NET or well-differentiated neuroendocrine carcinoma vs. poorly differentiated neuroendocrine carcinoma or mixed type), lymphatic invasion (negative vs. positive), Ki-67 labeling index (G1 vs. G2 or G3), COX2 (no overexpression vs. overexpression), and SSTR2 (positive vs. negative). Statistical analysis was performed using the SPSS ver. 15.0 (SPSS Inc., Chicago, IL).
Patient characteristics by tumor site are summarized in Table 1. Tumor sites of NET in those patients were, as follows: 86 foregut (49 stomach, 29 duodenum, 8 pancreas), 156 hindgut (18 colon, 138 rectum), and 5 metastatic tumors in the liver (3 colon primary, 2 pancreas primary). Two hundred and twenty-five (91%) of 247 tumors were confined within an organ, and 21 (9%) tumors were in an advanced stage: 9 foregut, 7 hindgut primary tumors, and 5 liver metastatic tumors. Synchronous cancer was associated in 19 (22%) foregut and 5 (3%) hindgut primary tumors. Histologically poor differentiation was observed in 20 (23%) foregut tumors, 14 (9%) hindgut primary tumors, and 1 (20%) metastatic liver tumor. The proportion of patient death was significantly higher in foregut patients than in hindgut patients (33% [22 of 66] vs. 5% [8 of 123], p<0.001).
Intracellular localization of proteins was shown, as follows: COX2 and SSTR5 in the cytoplasm, CgA, Ki-67, and SSTR1 in the nuclei, and SSTR2 in the membrane of tumor cells (Fig. 1). Overall, COX2 overexpression was observed in 54% (126 of 234), CgA positivity in 35% (82 of 232), SSTR1 expression in 84% (196 of 233), SSTR2 expression in 72% (168 of 233), and SSTR5 expression in 55% (128 of 232). Ki-67 labeling index was obtainable in 232 cases and scored as G1 in 65% (150 cases), G2 in 27% (63 cases), and G3 in 8% (19 cases). COX2 overexpression showed significant association with poor differentiation, higher stage, and lymphatic invasion. Ki-67 labeling index was higher in foregut NETs, poorly differentiated NETs, and NETs with lymphatic invasion. In terms of primary tumor site, rates of CgA positivity and G2 or G3 grade Ki-67 were higher in foregut tumors; in contrast, expression of all SSTR subtypes was higher in hindgut tumors (Table 2).
All of SSTR1, SSTR2, and SSTR5 expression was significantly associated with better differentiation, lower pathologic stage, and absence of lymphatic invasion. Among the 3 SSTR subtypes, primary site-specific difference was noted for SSTR5, which was higher in hindgut NETs than in foregut NETs (Table 2).
Correlation analysis in the expression of 6 proteins used in this study was performed. Strong correlations were noted in any comparative pairs of SSTR1, SSTR2, or SSTR5 expression (ρ>0.3, p<0.001). An inverse correlation between COX2 and SSTR2 expression was noted in foregut NETs (ρ=-0.3, p=0.002).
Kaplan-Meier analyses showed decreased mean OS for patients with COX2 overexpression (92 months vs. 127 months, p=0.003) and patients with G2 or G3 grades Ki-67 labeling indices (82 months vs. 126 months, p<0.001). In contrast, SSTR2 expression was found to be significantly associated with better mean OS (127 months vs. 66 months, p<0.001) (Fig. 2). SSTR1 expression (55 months vs. 120 months, p<0.001) and SSTR5 expression (101 months vs. 118 months, p=0.003) were also associated with better mean OS.
Multivariate regression analysis with variables including pathologic stage, histology, Ki-67 labeling index, lymphatic invasion, COX2 overexpression, and SSTR2 expression was performed. Stage (hazard ratio, 4.836; 95% confidence interval [CI], 1.997 to 11.710; p<0.001), Ki-67 labeling index (hazard ratio, 2.863; 95% CI, 1.096 to 7.476; p=0.032), and negative SSTR2 expression were independent prognostic markers in GEP-NETs (hazard ratio, 6.565; 95% CI, 2.412 to 17.872; p<0.001); however, histologic type, presence of lymphatic invasion, or COX2 overexpression were not independent prognostic markers (Table 3).
The present series consisted of 86 (35%) foregut primary, 156 (63%) hindgut primary, and 5 (2%) liver metastatic NETs. In 225 (91%) cases, tumors were confined to an organ, and in 21 (9%) cases, tumors were either locally advanced or metastatic. Midgut carcinoid tumor was not included in the present study, which reflects the low prevalence of midgut carcinoid tumors in Asia [14].
The presence of COX2-immunoreactive neuroendocrine cells was observed not only in the large intestine, but also in the stomach, duodenum, and small intestine [3]. A few authors have reported that elevated COX2 expression was involved in the tumorigenesis of pancreatic endocrine [6,15] and gastrointestinal carcinoid tumors [5], and that COX inhibitors are currently being developed for chemopreventive purposes and as adjuvant drugs for the treatment of several human malignancies. NET appear to be an attractive target for such therapies, because of their low proliferative capacity and relative resistance to both chemotherapy and bio-therapeutic modalities, such as interferon-alpha or somatostatin therapy. In a cell culture model of COX2-expressing neuroendocrine neoplasia, they found that COX2 up-regulated the expression and bioactivity of CgA. They also showed that the COX inhibitor sulindac had direct antiproliferative effects on GEP-NET cell lines, as well as colon cancer cell lines [4].
Some links between COX2 and somatostatin, such as extracellular signal-regulated protein kinase, a downstream molecule in the mitogen-activated protein kinase pathway induced by SSTR binding, have been reported [16]. Furthermore, rofecoxib and octreotide were found to have synergic antineoplastic effects in vitro in SGC-7901 cells (a gastric cancer cell line) and in xenografts in nude mice [17], and human gastric adenocarcinoma growth was found to be inhibited preoperatively by a combination of celecoxib and octreotide [18].
The placebo-controlled, prospective randomized study group reported that the long-acting octreotide showed substantial antitumor effect and longer progression-free survival than placebo in patients with well-differentiated NETs and a low tumor burden [19]. The antineoplastic activity of somatostatin analogs has also been demonstrated in several experimental models, both in vivo and in vitro. Mitosis inhibition was mediated by SSTRs 2 and 5 and resulted in cell cycle arrest [20]. Similarly, the loss of the SSTR2 expression in some human adenocarcinomas seemed to be responsible for losing the regulation of cell proliferation [21].
There are a few reports that suggest the need for indications for somatostatin analog treatment and for predictive markers of treatment response and somatostatin analog resistance. Slaby et al. [22] examined the gene expression of SSTR subtypes by quantitative reverse transcriptase-polymerase chain reaction (RT-PCR) and the correlations between those expression and the outcome of GEP-NET treatment with somatostatin analogs, and found that only SSTR4 was significantly associated with the stabilization of disease during somatostatin analog therapy. In contrast, Toboada et al. [23] showed that elevated tumor expression of SSTR1, SSTR2, and dopamine receptor 2 may help improve responsiveness to somatostatin analogs.
SSTR expression in GEP endocrine tumors is heterogeneous. The types most commonly detected are SSTR1 and 2, followed by SSTR3 and 5, and then by SSTR4, which is poorly represented in GEP-NETs [24]. A correlative immunohistochemical and RT-PCR analysis showed that SSTRs 1-5 were heterogeneously expressed in GEP endocrine tumors and that immunohistochemistry was a reliable tool to detect SSTRs 2, 3, and 5 in both surgical and biopsy specimens [24].
In our series, the overall positive rates of SSTRs in GEP-NETs were 84% for SSTR1, 72% for SSTR2, and 55% for SSTR5. The expression of all 3 subtypes was more frequently observed in well-differentiated NETs, hindgut primary NETs, and NETs with a low pathologic stage. Further stratified analysis in a larger series is required to examine the clinical utilization of the expression of SSTRs in the treatment of GEP-NETs as a predictive marker for response to somatostatin analog treatment.
The present study showed that COX2 overexpression in GEP-NETs is associated with aggressive pathologic variables and poor OS. In contrast, the expression of SSTR subtypes is shown to be associated with better OS. Furthermore, inverse correlations were noted between the expression of COX2 and SSTRs in GEP-NETs. Our results also suggest that COX2 could be a therapeutic target in a selected subset of GEP-NETs.
Acknowledgments
The authors thank Hye Jung Lee and Seung Hee Jung for their technical assistance with the tissue array construction and immunohistochemical studies.
The research was supported by the Korean Cancer Association Novartis Research Grant.
References
1. Oberg K, Eriksson B. Endocrine tumours of the pancreas. Best Pract Res Clin Gastroenterol. 2005; 19:753–781. PMID: 16253899.
2. Plöckinger U, Rindi G, Arnold R, Eriksson B, Krenning EP, de Herder WW, et al. Guidelines for the diagnosis and treatment of neuroendocrine gastrointestinal tumours: a consensus statement on behalf of the European Neuroendocrine Tumour Society (ENETS). Neuroendocrinology. 2004; 80:394–424. PMID: 15838182.
3. Nakajima T, Hamanaka K, Fukuda T, Oyama T, Kashiwabara K, Sano T. Why is cyclooxygenase-2 expressed in neuroendocrine cells of the human alimentary tract? Pathol Int. 1997; 47:889–891. PMID: 9503473.


4. Detjen KM, Welzel M, Wiedenmann B, Rosewicz S. Nonsteroidal anti-inflammatory drugs inhibit growth of human neuroendocrine tumor cells via G1 cell-cycle arrest. Int J Cancer. 2003; 107:844–853. PMID: 14566837.


5. Cadden I, Johnston BT, Turner G, McCance D, Ardill J, McGinty A. An evaluation of cyclooxygenase-2 as a prognostic biomarker in mid-gut carcinoid tumours. Neuroendocrinology. 2007; 86:104–111. PMID: 17700013.


6. Ohike N, Morohoshi T. Immunohistochemical analysis of cyclooxygenase (COX)-2 expression in pancreatic endocrine tumors: association with tumor progression and proliferation. Pathol Int. 2001; 51:770–777. PMID: 11881729.


7. Volante M, Bozzalla-Cassione F, Papotti M. Somatostatin receptors and their interest in diagnostic pathology. Endocr Pathol. 2004; 15:275–291. PMID: 15681851.


8. Eriksson B, Oberg K. Summing up 15 years of somatostatin analog therapy in neuroendocrine tumors: future outlook. Ann Oncol. 1999; 10(Suppl 2):S31–S38. PMID: 10399030.


9. Missiaglia E, Dalai I, Barbi S, Beghelli S, Falconi M, della Peruta M, et al. Pancreatic endocrine tumors: expression profiling evidences a role for AKT-mTOR pathway. J Clin Oncol. 2010; 28:245–255. PMID: 19917848.


10. Butturini G, Bettini R, Missiaglia E, Mantovani W, Dalai I, Capelli P, et al. Predictive factors of efficacy of the somatostatin analogue octreotide as first line therapy for advanced pancreatic endocrine carcinoma. Endocr Relat Cancer. 2006; 13:1213–1221. PMID: 17158766.


11. Greene FL, Page DL, Fleming ID, Fritz A, Balch CM, Haller DG, et al. AJCC cancer staging manual. 2002. 6th ed. New York, NY: Springer-Verlag.
12. Solcia E, Klöppel G, Sobin LH. International histological classification of tumours. 2000. 2nd ed. Berlin: Springer-Verlag;p. 38–74.
13. Kostopoulos I, Arapantoni-Dadioti P, Gogas H, Papadopoulos S, Malamou-Mitsi V, Scopa CD, et al. Evaluation of the prognostic value of HER-2 and VEGF in breast cancer patients participating in a randomized study with dose-dense sequential adjuvant chemotherapy. Breast Cancer Res Treat. 2006; 96:251–261. PMID: 16538542.


14. Ito T, Sasano H, Tanaka M, Osamura RY, Sasaki I, Kimura W, et al. Epidemiological study of gastroenteropancreatic neuroendocrine tumors in Japan. J Gastroenterol. 2010; 45:234–243. PMID: 20058030.


15. Okami J, Nakamori S, Yamamoto H, Sakon M, Tsujie M, Hayashi N, et al. An immunohistochemical study of cyclooxygenase (COX)-2 expression in endocrine tumors of the pancreas. J Exp Clin Cancer Res. 2002; 21:569–576. PMID: 12636104.
16. Kwon KS, Chae HJ. Sodium salicylate inhibits expression of COX-2 through suppression of ERK and subsequent NF-kappaB activation in rat ventricular cardiomyocytes. Arch Pharm Res. 2003; 26:545–553. PMID: 12934647.
17. Tang C, Liu C, Zhou X, Wang C. Enhanced inhibitive effects of combination of rofecoxib and octreotide on the growth of human gastric cancer. Int J Cancer. 2004; 112:470–474. PMID: 15382074.


18. Huang MT, Chen ZX, Wei B, Zhang B, Wang CH, Huang MH, et al. Preoperative growth inhibition of human gastric adenocarcinoma treated with a combination of celecoxib and octreotide. Acta Pharmacol Sin. 2007; 28:1842–1850. PMID: 17959037.


19. Rinke A, Müller HH, Schade-Brittinger C, Klose KJ, Barth P, Wied M, et al. Placebo-controlled, double-blind, prospective, randomized study on the effect of octreotide LAR in the control of tumor growth in patients with metastatic neuroendocrine midgut tumors: a report from the PROMID Study Group. J Clin Oncol. 2009; 27:4656–4663. PMID: 19704057.


20. Schally AV, Nagy A. Chemotherapy targeted to cancers through tumoral hormone receptors. Trends Endocrinol Metab. 2004; 15:300–310. PMID: 15350601.


21. Buscail L, Saint-Laurent N, Chastre E, Vaillant JC, Gespach C, Capella G, et al. Loss of sst2 somatostatin receptor gene expression in human pancreatic and colorectal cancer. Cancer Res. 1996; 56:1823–1827. PMID: 8620499.
22. Slaby O, Sachlova M, Bednarikova M, Fabian P, Svoboda M, Vytopilova S, et al. Gene expression of somatostatin receptor 4 predicts clinical outcome of patients with metastatic neuroendocrine tumors treated with somatostatin analogs. Cancer Biother Radiopharm. 2010; 25:237–243. PMID: 20423238.


23. Taboada GF, Neto LV, Luque RM, Córdoba-Chacón J, de Oliveira Machado E, de Carvalho DP, et al. Impact of gsp oncogene on the mRNA content for somatostatin and dopamine receptors in human somatotropinomas. Neuroendocrinology. 2011; 93:40–47. PMID: 21079388.
24. Papotti M, Bongiovanni M, Volante M, Allìa E, Landolfi S, Helboe L, et al. Expression of somatostatin receptor types 1-5 in 81 cases of gastrointestinal and pancreatic endocrine tumors. A correlative immunohistochemical and reverse-transcriptase polymerase chain reaction analysis. Virchows Arch. 2002; 440:461–475. PMID: 12021920.
Fig. 1
Immunostaining results of gastroenteropancreatic neuroendocrine tumors for cyclooxygenase-2 (COX2), Ki-67, and somatostatin receptors (SSTRs) 1, 2, and 5. In neuroendocrine tumors, COX2 and SSTR5 are localized in the cytoplasm, Ki-67 and SSTR1 are in the nuclei, and SSTR2 is in the membrane. First column, negative; second column, moderate positive; third column, strong positive; fourth column, high power field of strong positive (immunohistochemical staining with DAB, first three columns,×200; fourth column,×400).
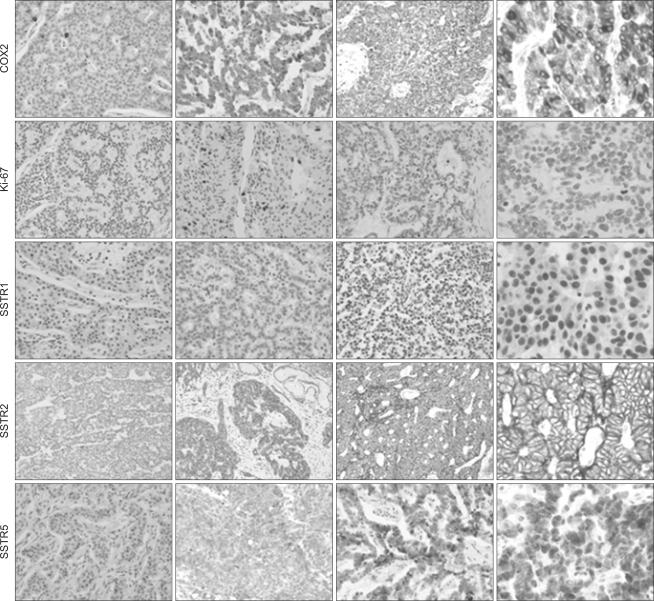
Fig. 2
Kaplan-Meier analyses of overall survival in 194 patients with gastroenteropancreatic neuroendocrine tumors. (A) Cyclooxygenase-2: solid line, no overexpression; dotted line, overexpression. (B) Ki-67: solid line, G1; dotted line, G2 or G3. (C) Somatostatin receptor 2: solid line, positive; dotted line, negative.
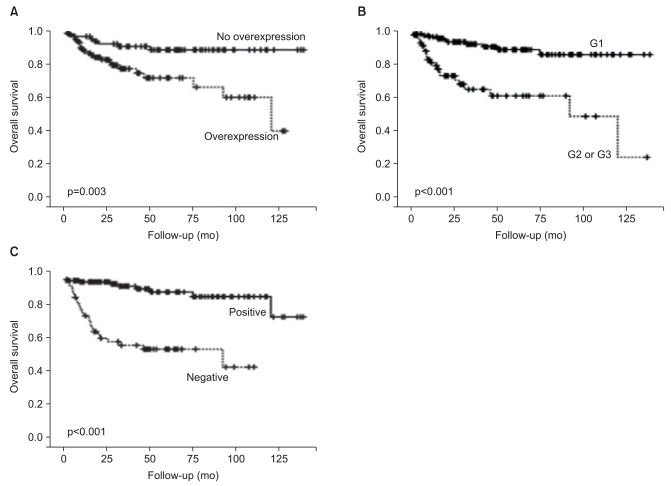
Table 1
Clinicopathologic features of gastroenteropancreatic neuroendocrine tumors
Table 2
Correlations between protein expression and pathologic variables in gastroenteropancreatic neuroendocrine tumors
Values are presented as number (%). WDNET, well-differentiated neuroendocrine tumor; WDNEC, well-differentiated neuroendocrine carcinoma; PDNEC, poorly differentiated neuroendocrine carcinoma; Mixed, mixed exo- and endocrine carcinoma; COX2, cyclooxygenase-2; CgA, chromogranin A; SSTR, somatostatin receptor.
Table 3
Multivariate analysis for overall survival in gastroenteropancreatic neuroendocrine tumors