Abstract
Purpose
We investigated the mechanism by which some types of cancer cells grow faster in the presence of ascorbic acid supplementation.
Materials and Methods
Adj.PC-5, a mouse plasmacytoma cell, is known to show ascorbic acid-dependent growth and was chosen as a test system. The growth of cancer cells was measured by the colony number on soft agar or the cellular proliferation in suspension culture. The ascorbate level was measured by a high performance liquid chromatography system with an electrochemical detector. Glucose 6-phosphate dehydrogenase was analyzed both on the specific enzyme activity level and on the transcription level by performing Northern blot analysis.
Results
Ascorbyl 2-phosphate among the ascorbate derivatives was the most efficient in stimulating cell growth. The intracellular and extracellular ascorbate concentrations following treatment with either ascorbate or ascorbyl 2-phosphate suggest that the superiority of ascorbyl 2-phosphate for stimulating cell growth may be due to its slow conversion to ascorbate in the culture medium. The steady transformation to ascorbate ensures sustained levels of ascorbate in the culture medium and thereby maximizes the growth stimulatory effect of ascorbate. Ascorbyl 2-phosphate markedly enhanced, in a concentration-and time-dependent manner, mRNA synthesis as well as the enzymatic activity of glucose 6-phosphate dehydrogenase, which is known to be a rate-limiting enzyme in cell growth. On the other hand, simultaneous addition of dehydroisoandrosterone, a well- known inhibitor of glucose 6-phosphate dehydrogenase, to the culture medium abrogated the growth stimulation by ascorbyl 2-phosphate, and it also reduced the glucose 6-phosphate dehydrogenase activity proportionately.
There is controversy about the positive effect of supplementing cancer patients with ascorbic acid. The carcinogenesis and the inhibition of carcinogenesis by ascorbic acid and other ascorbate derivatives, including the effects on cell proliferation, tumor promotion and tumor growth, have been recently reviewed by the Cosmetic Ingredient Review Expert Panel (1). The panel concluded that ascorbic acid is not carcinogenic, but sodium ascorbate can promote the development of urinary carcinomas in two-stage carcinogenesis studies, in which ascorbate was fed to animals that were pre-exposed to carcinogens. Although ascorbate at high concentrations has been shown to suppress tumor cell growth in various cell culture systems (2,3), lower physiological concentrations (<100µM) have been demonstrated to induce growth enhancement (4~6). Although the growth-stimulatory effects of ascorbate varied from one tumor cell type to another, it was most apparent for the cultures of primary cells that had been kept in culture medium containing ascorbate (7). Rather than generating oxidative stress in cells, ascorbate is one of the most efficient intracellular antioxidants, eliminating the reactive oxygen species produced both inside and outside the cell (8,9). These previous studies suggested to us that extracellular ascorbate above a threshold concentration might be cytotoxic, while intracellular ascorbate might have positive effects on cell growth.
Cell growth is directly correlated with glucose metabolism as well as the expression of many cell-cycle regulatory proteins. Among the enzymes involved in glucose metabolism, glucose 6-phosphate dehydrogenase (G6PD) is the first and key enzyme of the pentose-phosphate pathway that produces NADPH and ribose-5-phosphate. In primary cultures of liver cells, EGF and insulin were reported to stimulate growth of liver cells and also to increase G6PD activity (10). Moreover, a wide variety of tumors have been reported to express increased levels of G6PD activity, ranging from 3 to 20 times higher than the control levels (i.e., the adjacent normal tissues) (11~13). G6PD was demonstrated to be a rate-limiting enzyme for cell growth; increased intracellular G6PD activity due to its overexpression led to enhanced cell growth, while cultures treated with dehydroepiandrosterone, a G6PD-specific inhibitor (14), showed attenuation of cell growth (15). In particular, NADPH was proven to be the critical product of G6PD that is required for cell growth.
In this study we investigated the possibility that ascorbate stimulates cancer cell growth by augmenting G6PD activity. We used Adj.PC-5 cells that have been passaged in vivo (in the spleen) to maintain their ascorbate dependency and supplemented the culture medium with ascorbyl 2-phosphate magnesium salt (APM), which is a stable derivative of ascorbate.
L-Ascorbic acid, L-sodium ascorbate, glutathione, ascorbate oxidase, 2-mercaptoethanol and dehydroisoandrosterone were purchased from Sigma Chemical (St. Louis, MO). Dehydroascorbic acid and metaphosphoric acid were from Aldrich Chemical (Milwaukee, WI), and APM was from Wako Pure Chemical (Osaka, Japan). All the chemicals were of analytical grade.
Female BALB/c mice (20~25 g) were used. The mice were given Jeil Laboratory Chow (Seoul, Korea) and water ad libitum. The animals were kept at 21~24℃ and 40~60% relative humidity with a 12-hr light/dark cycle.
A mouse plasmacytoma cell line, Adj-PC5 (5), was maintained and transplanted by the intravenous injection of 1 to 5×106 cells to the BALB/c mice. The Adj.PC-5 cells mainly infiltrated into the spleen following intravenous injection. The tumor cell suspensions for the experiments in this study were prepared from the removed tumor-bearing spleens by grinding the with a syringe plunger on a fine wire mesh screen. The cells were cultured in complete RPMI 1640 medium (Gibco, Grand Island, NY) containing 5% fetal bovine serum (FBS, Gibco), 2 mM L-glutamine (Sigma), antibiotics (100 units penicillin and 100 mg streptomycin per ml) (Gibco) at 37℃ in a humidified 5% CO2 incubator.
Colony forming assay was performed according to a previously reported method (16), with minor modifications. Briefly, the cells (3×105 nucleated cells/dish) from the plasmacytoma-infiltrated spleens and normal spleens of the BALB/c mice were diluted and cultured on soft-agar (0.3%) plates. The agar culture was contained in a 35-mm tissue culture dish (Falcon, Lincoln Park, NJ) with 5 small holes made at the bottom and it was stored within a 60-mm culture dish (Falcon) for sterility. The cultures were incubated at 37℃ in a humid atmosphere that was continuously flushed with 5% CO2 and the cultures were fed daily with 0.5 ml of fresh RPMI 1640 medium containing 5% FBS, 2 mM L-glutamine, 100 units/ml penicillin and 100 g/ml streptomycin, plus the compounds to be assessed. The fed medium percolated through the agar layer and drained out through the holes made at the bottom of the culture dish. Colonies containing 50 or more cells were counted with an inverted microscope when the cultures were 2 weeks old.
The cells were adjusted to 3×105 nucleated cells per ml in RPMI 1640 medium supplemented with 5% FBS, 2 mM L-glutamine and antibiotics. Chemicals were directly added to the cell suspensions, and the suspensions were then transferred (200 µl aliquots) and cultured on 96-well microtiter plates (Costar, Cambridge, MA) at 37℃ and 5% CO2 for 48 h. The cells were pulsed with 0.5µCi/well of [3H]-thymidine (NEN, Boston, MA) for the last 6 h of the culture period. The thymidine incorporated into DNA was measured by scintillation counting.
Ascorbate was measured by a previously described method (3), with minor modifications. The high performance liquid chromatography (HPLC) system (Shimadzu Corp., Tokyo, Japan) consisted of a CBM-10A communication module, a L-ECD-6A electrochemical detector, a LC-10AD pump system and a CTO-10A column oven. The stationary phase was a Shim-pack CLC-ODS column (packed with octadesyl coated silica particles of 5µm diameter and a 10 nm pore diameter, the column was 6 mm×15 cm) (Shimadzu) connected to a Shim-pack G-ODS guard column (4 mm×1 cm) (Shimadzu). The mobile phase consisted of 50 mM potassium phosphate and 100µM ethylenediaminetetraacetic acid and it was adjusted to pH 2.5 with phosphoric acid. The mobile phase was filtered through 0.45µm JH hydrophilic filters (Millipore, Yonezawa, Japan) prior to use. Column equilibration was performed at a flow rate of 1.0 ml/min and this flow rate was used for all the subsequent analyses. The detector was set at 0.75 V versus an Ag/AgCl reference. After the indicated incubation periods, the cultures were centrifuged to separately collect the cells and supernatants. The cells were washed with phosphate-buffered saline and extracted in 5% metaphosphoric acid solution for 10 min. In the case of the culture supernatants, an equal volume of metaphosphoric acid (10%) was added to extract the ascorbic acid. The extract was filtered (0.22µm) and then applied to the HPLC column.
G6PD activity was measured by a previously described method (17). The cells were washed twice in phosphate-buffered saline and then homogenized in ice-cold 50 mM Tris·HCl buffer (pH 7.4) that contained 10 mM MgCl2, 0.5 mM phenylmethylsulfonyl fluoride, 1µg leupeptin per ml, and 1µg apro tinin per ml. The supernatants were collected following centrifugation (12,000 rpm) for 5 min at 4℃ and then they were used for the enzyme assay. A cuvette was loaded with 0.95 ml of the reaction mixture (50 mM Tris·HCl buffer; pH 7.4, 13.3 mM MgCl2, 1 mM NADP+, 1 mM glucose 6-phosphate and 1 mM maleimide) and stored at 30℃ for 5 min. A cell lysate volume of of 50µl was added to the cuvette and mixed well. The change in absorbance at 340 nm for the first 5 min following a 5-min lag period was monitored and normalized against the protein concentration to calculate the specific enzyme activity.
Total RNA was isolated from the Adj.PC-5 cells using RN-Agents® (Promega, Madison, MI). The RNA samples (30µg) were denatured with formaldehyde, electrophoresed on a 1.2% agarose gel that contained 4% formaldehyde and then they were transferred to a nylon membrane (Amersham, Arlington Heights, IL). The transferred RNA was crosslinked to the nylon membrane by ultraviolet irradiation. Hybridization was performed overnight at 42℃ in 50% formamide, 5X SSC, 100µg yeast tRNA per ml, 7.5% dextran sulfate, 10X Denhardt's solution and 2% sodium dodecyl sulphate (SDS) with a 32P-labeled probe that was the 0.5 kb PCR fragment of mouse G6PD cDNA. The nylon membrane was washed three times for 30 min at 40℃ each time with 2X SSPE (0.1% SDS), 1X SSPE (0.1% SDS) and 0.1X SSPE, and then it was exposed for 24 h at -70℃ for autoradiography with using an intensifying screen.
The mean±SD was determined for each treatment. The treatment groups were compared with the control groups by using Dunnett's two-tailed t-test (18), with statistical significance defined as p<0.05. All the experiments shown in this report were repeated at least three times to confirm their reproducibility.
Consistent with our previous reports (5,16), ascorbate added daily enhanced colony formation in the Adj.PC-5 cell cultures in a concentration-dependent manner at up to 100µM, but then there was a slight decrease at 200µM (Fig. 1A). However, ascorbate did not increase colony formation in the presence of ascorbate oxidase (0.5 units/ml). The oxidation and degradation of ascorbate that were catalyzed by ascorbate oxidase abrogated its stimulatory effect on colony growth, and this result suggests that the reduced form is essential for ascorbate to express its growth-stimulatory effect. Compared with colony growth, the incorporation of [3H]-thymidine into the cells growing in suspension culture represents the growth rate of the total cells. The spleen cells from the mice that were intravenously transplanted with Adj.PC-5 cells were adjusted to 5×105 nucleated cells per ml and these were cultured on a 96-well plate in the presence of ascorbate derivative. Fig. 1B shows the increased growth of Adj.PC-5 cells by the addition of ascorbate. Ascorbic acid, sodium ascorbate and APM all stimulated growth at up to 100µM. APM was more efficient at enhancing cell growth than either ascorbic acid or sodium ascorbate. The addition of only 25µM APM was enough to induce a significant increase in cell proliferation. In addition, enhancement of proliferation was observed even with a quite high concentration of APM, e.g., 400µM, which is a concentration that was cytotoxic in the case of either ascorbic acid or sodium ascorbate (data not shown). Meanwhile, the cells from normal spleens showed only background levels of proliferation (<1,000 dpm) regardless of the cell type and the amounts of added ascorbate derivative (data not shown).
Although the reduced state is important for ascorbic acid to enhance cell growth, as well as to act as an antioxidant, there may also be other factors that determine the efficiency of growth stimulation, such as the cellular transport efficiency and stability in culture medium. To further characterize the factors that determine the stimulatory efficiency for cell growth, we daily fed four different derivatives of ascorbate to Adj.PC-5 cell cultures (Fig. 2). The sodium salt form of ascorbate enhanced colony formation at the same level as was observed in the ascorbic acid-treated cultures. Thus, the ascorbic acid-induced pH change does not appear to be a major factor for the modulation of cell growth by ascorbic acid. Dehydroascorbate, an oxidized form of ascorbate, was not effective at stimulating cell growth, as was anticipated from the ascorbate oxidase experiments. The highest colony forming response was observed in the cultures treated with APM, showing an approximately 10-fold increase at 100µM over the untreated control. APM is a stable derivative of ascorbic acid and slowly but steadily converted to ascorbic acid by phosphatases in both the medium and the cell cytoplasm. However, it is not yet certain which phosphatase is responsible for the dephosphorylation. This result implies that not only the redox state, but also the consistency of the ascorbic acid concentration may be important in order for it to stimulate cell growth.
APM was better than ascorbate in terms of both low cytotoxicity and growth stimulation. To elucidate that the superiority of APM on cell growth was due to its slow transformation to ascorbate as well as the resultant steady concentration of extracellular ascorbate, we examined the intracellular and extracellular ascorbate concentration kinetics following the treatment with either ascorbate or APM (200µM). As shown in Fig. 3A, the ascorbate level in the medium decreased in a time-dependent manner, leaving 38% and 4% of the initial concentration at 24 and 48 h, respectively. Meanwhile, the intracellular ascorbate increased, reaching a maximum at 12 h, and then gradually decreased. In contrast to the culture treated with ascorbate, both the extra- and intracellular ascorbate concentration in the APM-treated culture started with 0µM (Fig. 3). The ascorbate concentration in the medium increased over time until 12 h following APM treatment and then it showed a plateau for 50µM between 12~24 h. The intracellular level of ascorbate also increased to approximately 1.4 nmoles/106 cells by 12 h after treatment and then it decreased thereafter. Although the level was quite low, ascorbate was detectable in both the cells and the medium of the cultures at 72 h following the start of APM treatment. However, no remaining ascorbate was detected at 72 h in either the cells or supernatants of the ascorbate-treated cultures.
We measured the effects of ascorbate on the enzyme activities involved in the regulation of cellular reduction-oxidation conditions, including superoxide dismutase, catalase, glutathione reductase and G6PD. G6PD was the only enzyme that showed a significantly increased level of activity after a 72-h incubation with ascorbate (data not shown). Since G6PD produces NADPH and ribose-5-phosphate and it is known to be a rate-limiting enzyme for cell growth, we further investigated the concentration-dependent and time-dependent effects of ascorbate on G6PD activity. The G6PD activity was increased in a concentration-dependent fashion in the Adj.PC-5 cell cultures treated with APM for 72 h (Fig. 4A). Approximately 50% enhancement was observed in the culture treated with 400µM of ascorbate. As shown in Fig. 4B, the G6PD activity was slowly increased in a time-dependent manner. This 50% increase of G6PD activity was quite consistent with the growth stimulation level by APM, suggesting the possibility that the enhanced G6PD activity is an underlying mechanism for the ascorbate-induced stimulation of cell growth. To determine whether this increase of G6PD activity was accompanied with an increase of G6PD mRNA, we performed Northern blot analysis (Fig. 4C). The level of G6PD mRNA was enhanced by the APM (200µM) treatment in a time-dependent manner and this was consistent with the results of the change of enzyme activity.
In order to determine if hydrogen peroxide produced from ascorbate has a specific role in the enhancement of growth and the G6PD activity induced by APM, catalase (10 units/ml) was exogenously added to the culture medium. While the addition of catalase to the Adj.PC-5 cell cultures increased colony growth regardless of the existence of APM, the G6PD activity in the Adj.PC-5 cells was not altered by APM (Fig. 5). These results suggest that growth enhancement by catalase was mostly an indirect effect mediated through scavenging cytotoxic hydrogen peroxide in the culture medium and that the increased G6PD by APM was not due to the hydrogen peroxide produced during ascorbate degradation.
Dehydroepiandrosterone is a well-known G6PD inhibitor (14). To determine whether the cell growth enhancement by ascorbate was associated with the increase of G6PD activity in cells, dehydroepiandrosterone was added to the Adj.PC-5 cell cultures. As anticipated, dehydroepiandrosterone was able to inhibit G6PD activity in the cytosolic fraction prepared from the Adj.PC-5 cells (Fig. 6A). Meanwhile, daily administered dehydroepiandrosterone efficiently abolished the APM-induced increase of cell growth, as measured by colony formation in the Adj.PC-5 cell culture (Fig. 6B). Thus, this data suggests that the enhancement of growth and G6PD activity in Adj.PC-5 cells that was observed following APM treatment may be closely associated.
The ascorbic acid concentrations in most of the studies that have examined tumor cell cytotoxicity were higher than those that are observed in plasma samples. Since any plasma ascorbate higher than a threshold level is excreted in the urine via the kidneys, it is difficult to observe cytotoxic concentrations of ascorbate in plasma, unless it is given by intravenous infusion at a very high dose. Furthermore, ascorbate is more stable in plasma than in culture medium because a variety of other antioxidants or reducing mechanisms in vivo efficiently extinguish oxidant stresses.
Meanwhile, there has been a steady stream of reports on the stimulatory effects of ascorbic acid on the growth of tumor and primary cells. Compared with growth suppression, growth enhancement was generally observed when cells were treated with relatively low ascorbate concentrations. The result showing greater colony formation at 100µM than at 200µM of ascorbate (Fig. 1A) suggests that ascorbate has a growth stimulatory effect rather than an inhibitory effect under physiological conditions. To retain the tumor cells' dependence on ascorbate for their growth, the Adj.PC-5 cells were passaged in vivo by intravenous administering these cells to BALB/c mice. APM was the most efficient among the ascorbate derivatives we tested for enhancing cell growth (Fig. 1B). This superiority of APM for cell growth seems to be due to its slow conversion to ascorbate. The slow conversion of APM prevented attaining a cytotoxic ascorbate concentration in the culture medium (Fig. 3). While APM did not increase the ascorbate concentration in the culture medium over the threshold level (about 80µM) even after treatment with ascorbate at 200µM, it took about 24 h for the medium concentration of ascorbate to fall below the threshold. Thus, the slight decrease in colony growth at 200µM of ascorbate is likely to be due to exposure to the high concentration of ascorbate for the first 24 h. In contrast to the extracellular kinetics, similar patterns of intracellular ascorbate kinetics were observed following treatment with either ascorbate or APM. These results imply that intracellular ascorbate is responsible for the stimulation of cell growth while extracellular ascorbate explains the observed growth suppression.
Extensive studies have been devoted to elucidating the mechanism of ascorbate-induced growth modulation. H2O2 has been proposed as a feasible cytotoxic mediator produced during ascorbic acid oxidation because the addition of catalase abrogated the cytotoxic effect of ascorbic acid (19,20). In addition, ascorbic acid alone was reported to produce reactive oxygen species, including H2O2, through reactions with molecular oxygen (20). In contrast, the mechanism by which ascorbate stimulates cell growth has not yet been thoroughly studied. This was probably because the nonspecific protective roles of ascorbate as an antioxidant were considered to be the mechanism. Protecting cells from the reactive oxygen species, whether they are introduced exogenously or they are produced endogenously during oxidative phosphorylation in mitochondria, could be a way to eliminate the negative environment for cell growth and so this contributes to growth enhancement (21). Not only ascorbate, but also its oxidation products dehydroascorbate and diketogulonic acid have been demonstrated to efficiently eliminate hydrogen peroxide (8).
The role of ascorbate as a scavenger of reactive oxygen species, which protects cells against peroxidatives, seems to be particularly important for fast growing cells such as tumor cells that produce more endogenous reactive oxygen species. However, it was reasonable for us to consider that positive regulatory mechanisms as well as negative ones were involved in the ascorbate-induced growth enhancement. After a series of experiments investigating the effects of ascorbate treatment on the enzymes involved in the regulation of the cellular redox state, such as glutathione reductase, superoxide dismutase, catalase and G6PD, we found that only the G6PD activity was significantly increased in the Adj.PC-5 cells treated with ascorbate. Of interest is that G6PD was reported to be a rate-limiting enzyme for cell growth (15). The increased level of G6PD activity was both concentration-dependent and time-dependent. Moreover, dehydroepiandrosterone was able to inhibit G6PD activity in the Adj.PC-5 cells and it abrogated the growth enhancement induced by APM. Thus, these results imply that the increased G6PD activity following ascorbate treatment is at least in part responsible for the observed growth enhancement. It is interesting to see that ascorbic acid has a regulatory function in glucose metabolism. Ascorbic acid is synthesized from glucose via gulonolactone and has a structural similarity with glucose. Dehydroascorbic acid, an oxidized form of ascorbic acid, is transported into cells through glucose transporters (22).
The present study suggests that the ascorbate-induced growth enhancement of Adj.PC-5 cells is accompanied by the augmentation of G6PD mRNA and activity. However, the molecular mechanism by which ascorbate induces a rise of G6PD activity in cells is still open to debate. Although G6PD is a housekeeping enzyme and it is constitutively expressed in all tissues, its expression level varies between tissues and is subject to tissue-specific regulation by hormones, growth factors, nutrients and oxidative stress (23). Like the other housekeeping genes, a high G+C content and two functional GC boxes were found in the promoter of the G6PD gene (24). Further studies are required to elucidate the transcriptional and post-transcriptional regulation of the G6PD expression by ascorbate. It is also interesting to see that ascorbic acid has a regulatory function in glucose metabolism since ascorbic acid is synthesized from glucose via gulonolactone. Gulonolactone oxidase, which does not function in human, is the enzyme that catalyzes the final step in the biosynthesis of ascorbic acid.
References
1. Elmore AR. Final report of the safety assessment of L-Ascorbic Acid, Calcium Ascorbate, Magnesium Ascorbate, Magnesium Ascorbyl Phosphate, Sodium Ascorbate, and Sodium Ascorbyl Phosphate as used in cosmetics. Int J Toxicol. 2005; 24(Suppl 2):51–111. PMID: 16154915.
2. Casciari JJ, Riordan NH, Schmidt TL, Meng XL, Jackson JA, Riordan HD. Cytotoxicity of ascorbate, lipoic acid, and other antioxidants in hollow fibre in vitro tumours. Br J Cancer. 2001; 84:1544–1550. PMID: 11384106.


3. Koh WS, Lee SJ, Lee H, Park C, Park MH, Kim WS, et al. Differential effects and transport kinetics of ascorbate derivatives in leukemic cell lines. Anticancer Res. 1998; 18:2487–2493. PMID: 9703897.
4. Park CH, Amare M, Hoogstraten B. Analysis of the growth enhancing effect of L-ascorbic acid on human leukemic cells in culture. Exp Hematol. 1980; 8:853–859. PMID: 16398016.
5. Park CH, Bergsagel DE, McCulloch EA. Ascorbic acid: a culture requirement for colony formation by mouse plasmacytoma cells. Science. 1971; 174:720–722. PMID: 5123422.


6. Venezian R, Shenker BJ, Datar S, Leboy PS. Modulation of chondrocyte proliferation by ascorbic acid and BMP-2. J Cell Physiol. 1998; 174:331–341. PMID: 9462695.


7. Nowak G, Schnellmann RG. L-ascorbic acid regulates growth and metabolism of renal cells: improvements in cell culture. Am J Physiol. 1996; 271:C2072–C2080. PMID: 8997210.


8. Deutsch JC. Ascorbic acid oxidation by hydrogen peroxide. Anal Biochem. 1998; 255:1–7. PMID: 9448835.


9. Mendiratta S, Qu Z, May JM. Erythrocyte defenses against hydrogen peroxide: the role of ascorbic acid. Biochim Biophys Acta. 1998; 1380:389–395. PMID: 9555101.


10. Yoshimoto K, Nakamura T, Ichihara A. Reciprocal effects of epidermal growth factor on key lipogenic enzymes in primary cultures of adult rat hepatocytes. Induction of glucose-6-phosphate dehydrogenase and suppression of malic enzyme and lipogenesis. J Biol Chem. 1983; 258:12355–12360. PMID: 6355085.


11. Baba M, Yamamoto R, Iishi H, Tatsuta M, Wada A. Role of glucose-6-phosphate dehydrogenase on enhanced proliferation of pre-neoplastic and neoplastic cells in rat liver induced by N-nitrosomorpholine. Int J Cancer. 1989; 43:892–895. PMID: 2565888.


12. Deshpande N, Mitchell I, Millis R. Enzyme studies in human breast tumours. Eur J Cancer. 1977; 13:1261–1267. PMID: 145367.


13. Heyden G. Histochemical investigation of malignant cells. Histochemistry. 1974; 39:327–334. PMID: 4859353.


14. Shepherd A, Cleary MP. Metabolic alterations after dehydroepiandrosterone treatment in Zucker rats. Am J Physiol. 1984; 246:E123–E128. PMID: 6230014.


15. Tian WN, Braunstein LD, Pang J, Stuhlmeier KM, Xi QC, Tian X, et al. Importance of glucose-6-phosphate dehydrogenase activity for cell growth. J Biol Chem. 1998; 273:10609–10617. PMID: 9553122.


16. Park CH, Bergsagel DE, McCulloch EA. Mouse myeloma tumor stem cells: a primary cell culture assay. J Natl Cancer Inst. 1971; 46:411–422. PMID: 5115909.
17. Kornberg A, Horecker BL. Colowick SP, Kaplan NO, editors. Glucose-6-phosphate dehydrogenase. Methods of enzymology. 1955. New York: Academic Press;p. 323.
18. Dunnett CW. A multiple comparison procedure for comparing several treatments with a control. J Am Statist Assoc. 1955; 50:1096–1121.


19. Arakawa N, Nemoto S, Suzuki E, Otsuka M. Role of hydrogen peroxide in the inhibitory effect of ascorbate on cell growth. J Nutr Sci Vitaminol (Tokyo). 1994; 40:219–227. PMID: 7965211.


20. Miwa N, Yamazaki H, Nagaoka Y, Kageyama K, Onoyama Y, Matsui-Yuasa I, et al. Altered production of the active oxygen species is involved in enhanced cytotoxic action of acylated derivatives of ascorbate to tumor cells. Biochim Biophys Acta. 1988; 972:144–151. PMID: 3191161.


21. May JM. Ascorbate function and metabolism in the human erythrocyte. Front Biosci. 1998; 3:d1–d10. PMID: 9405334.


22. Kuo SM, MacLean ME, McCormick K, Wilson JX. Gender and sodium-ascorbate transporter isoforms determine ascorbate concentrations in mice. J Nutr. 2004; 134:2216–2221. PMID: 15333707.


23. Kletzien RF, Harris PK, Foellmi LA. Glucose-6-phosphate dehydrogenase: a "housekeeping" enzyme subject to tissue-specific regulation by hormones, nutrients, and oxidant stress. Faseb J. 1994; 8:174–181. PMID: 8119488.


24. Philippe M, Larondelle Y, Lemaigre F, Mariame B, Delhez H, Mason P, et al. Promoter function of the human glucose-6-phosphate dehydrogenase gene depends on two GC boxes that are cell specifically controlled. Eur J Biochem. 1994; 226:377–384. PMID: 8001555.


Fig. 1
Ascorbate-induced stimulation of Adj.PC-5 cell growth. (A) Colony growth in the Adj.PC-5 cell cultures in the presence (open circles) or absence (closed circles) of ascorbate oxidase (AO). Spleen cells (3×105 nucleated cells/dish) from the plasmacytoma (Adj.PC-5)-infiltrated spleens of BALB/c mice were cultured on soft-agar (0.3%) plates. The cultures were daily fed with 0.5 ml of complete RPMI 1640 medium with ascorbate in the presence or absence of ascorbate oxidase (0.5 unit/ml). The colony counts were normalized to the untreated control (52 colonies/dish). (B) Effects of ascorbate derivatives on the proliferation of Adj.PC-5 cells. Ascorbate derivatives were directly added to cell suspensions (3×105 nucleated cells/ml), which were then transferred in 200µl aliquots and cultured on 96-well microtiter plates at 37℃ and 5% CO2 for 48 h. Cell growth was determined by the incorporation of [3H]-thymidine for the last 6 h of the culture period. Each point shows the mean±SD of quadruplicate determinations. *significantly different from control (p<0.05). ASC, ascorbic acid (closed circles); ASS, sodium ascorbate (open circles); APM, magnesium ascorbyl 2-phosphate (closed triangles).
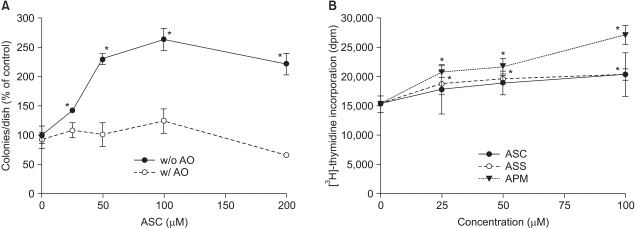
Fig. 2
Effects of the various forms of ascorbate on colony growth in Adj.PC-5 cell cultures. Adj.PC-5-infiltrated spleen cells were adjusted to 3×105 nucleated cells/dish and then cultured as described in Fig. 1. The cultures were daily fed with 0.5 ml of complete RPMI 1640 medium containing either 50 or 100µM of the indicated ascorbate derivative. The colony counts were normalized to the untreated control. Each bar represents the mean±SD of triplicate determinations. *significantly different from control (p<0.05). DHA, dehydroascorbic acid.
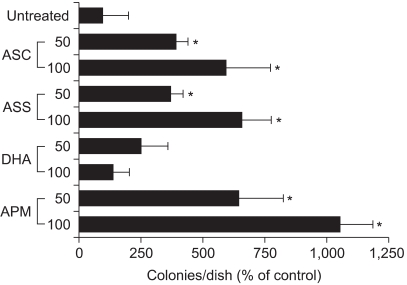
Fig. 3
Differential kinetics of ascorbate and APM in the cells and the culture medium. Prior to the experiments, Adj.PC-5 cells were maintained in medium that contained no ascorbate for at least 10 days to confirm they had no endogenous ascorbate. Experimental cultures were treated with either 100µM (closed circles) or 200µM (open circles) of ascorbate or either 100µM (closed triangles) or 200µM (open triangles) of APM for the indicated times and then the cells and supernatants separately collected. (A) Extracellular ascorbate concentration. The culture supernatant was mixed with an equal volume of metaphosphoric acid (10%) to extract the ascorbic acid. (B) Intracellular ascorbate concentration. The cells were washed with phosphate-buffered saline and extracted in 5% metaphosphoric acid solution for 10 min. The extracts from both the cells and culture supernatants were filtered (pore size: 0.22µm) and then applied to the HPLC analysis as described in Materials and Methods. Each point represents the mean±SD of triplicate determinations.
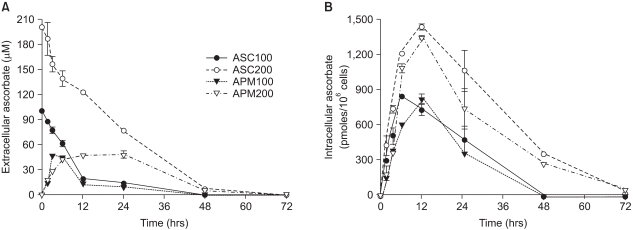
Fig. 4
Augmented G6PD activity and mRNA in the Adj.PC-5 cells cultured in the presence of APM. (A) The G6PD activity increase induced by APM was concentration dependent. Adj.PC-5 cells (approximately 5×106 cells) were harvested after 72 h incubation with APM and then homogenized. The supernatants after centrifugation at 14,000 rpm for 10 min were used for G6PD assays. (B) Time course of the increased G6PD activity. G6PD activities were measured from the Adj.PC-5 cells incubated with 0 (closed circles), 100 (open circles), or 200 (closed triangles)µM of APM for the indicated times. G6PD activity was measured by spectrophotometry at 340 nm by the reduction of NADP+ in the presence of glucose-6-phosphate. Each point represents the mean activity±SD of triplicate determinations. (C) Northern blot analysis of the G6PD mRNA. The total RNA was isolated from the cells treated with APM (200µM) for 0, 3, 12, 24 and 48 h, respectively. The RNA (30µg) was electrophoresed, transferred to nylon membranes and hybridized with a 32P-labeled probe of mouse G6PD cDNA. The control, 28S and 18S RNA in the gel.
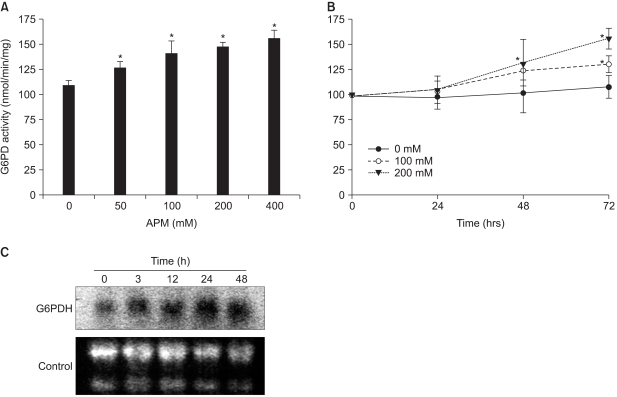
Fig. 5
Effect of adding catalase to the Adj.PC-5 cell cultures. (A) Colony growth stimulated by APM. Colony forming assay was performed as described in Materials and Methods. Catalase and APM were prepared in the feeding medium and this was added daily to culture. (B) G6PD activity enhanced by APM. The cells were cultured with the indicated treatments for 72 h and then the cells were collected. G6PD activity was determined as described in Materials and Methods. Each bar represents the mean±SD of triplicate determinations. *significantly different from control (p<0.05). Catalase (10 units/ml); APM (200µM).
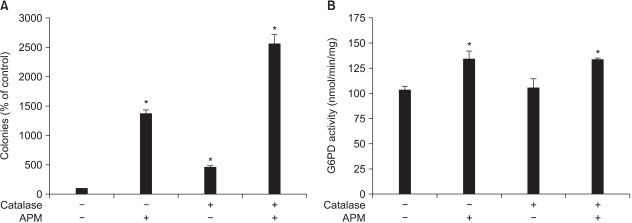
Fig. 6
Effect of DHEA. (A) Effect on G6PD activity in Adj.PC5 cell lysates. The cell lysate for G6PD assay was prepared as described in Materials and Methods. DHEA was tested to determine its inhibitory effect on G6PD activity. Each point represents the mean±SD of triplicate determinations. The effect of DHEA, dehydroepiandrosterone, on colony growth enhanced by the addition of APM. The colony forming assay was performed as described in Materials and Methods. DHEA was dissolved in dimethylsulfoxide (final dimethylsulfoxide concentration in medium: 0.1%) and this was added daily to the Adj.PC-5 cell cultures that were also treated with APM. Each bar represents the mean±SD of quadruplicate cultures. *significantly different from control (p<0.05).
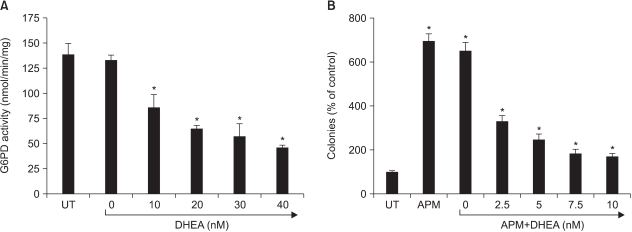