Abstract
Endoscopic assessment has a crucial role in the management of inflammatory bowel disease (IBD). It is particularly useful for the assessment of IBD disease extension, severity, and neoplasia surveillance. Recent advances in endoscopic imaging techniques have been revolutionized over the past decades, progressing from conventional white light endoscopy to novel endoscopic techniques using molecular probes or electronic filter technologies. These new technologies allow for visualization of the mucosa in detail and monitor for inflammation/dysplasia at the cellular or sub-cellular level. These techniques may enable us to alter the IBD surveillance paradigm from four quadrant random biopsy to targeted biopsy and diagnosis. High definition endoscopy and dye-based chromoendoscopy can improve the detection rate of dysplasia and evaluate inflammatory changes with better visualization. Dye-less chromoendoscopy, including narrow band imaging, iScan, and autofluorescence imaging can also enhance surveillance in comparison to white light endoscopy with optical or electronic filter technologies. Moreover, confocal laser endomicroscopy or endocytoscopy have can achieve real-time histology evaluation in vivo and have greater accuracy in comparison with histology. These new technologies could be combined with standard endoscopy or further histologic confirmation in patients with IBD. This review offers an evidence-based overview of new endoscopic techniques in patients with IBD.
Inflammatory bowel disease (IBD) includes Crohn’s disease (CD) and ulcerative colitis (UC), is a chronic, relapsing inflammatory disease in the gastrointestinal tract. The cause of IBD is unknown. It has been suggested that genetic, environmental, and immunologic factors are involved in the pathogenesis of IBD, but the precise etiologic mechanisms remain unclear.
Diagnostic and therapeutic approaches for IBD have evolved over the past decades, but precise diagnosis and assessment of disease status is still an important matter of concern for physicians and IBD specialists. Precise diagnosis and assessment of patients with IBD is particularly difficult because medical therapies, surgical approaches, and long-term prognosis differ by IBD subtypes, even if patients have similar signs and symptoms.
The most valuable tool for primary diagnosis of IBD is endoscopic assessment with tissue sampling [1,2]. It can be used to observe inflammatory changes in the intestinal mucosa, evaluate the extent of disease. It also plays a role in assessing treatment efficacy in terms of mucosal healing and the risk of postsurgical recurrence. Importantly, colonoscopy with random biopsy is essential to endoscopic diagnosis, management, and treatment of IBD. The relationship between longstanding IBD and increased colorectal cancer (CRC) risk has been well established [3]. CRC is regarded as the primary cause of death in up to 15% of IBD patients. The overall rate of CRC in UC patients is 3.7% with cumulative probabilities of 18% by 30 years, according to a metaanalysis of 116 studies on the subject [4]. There is also a 2–3 fold increased risk of CRC in CD than in patients without IBD 18.3 years after initial CD diagnosis [5]. Recent studies suggest a decreased risk of CRC in IBD patients as highly developed endoscopic surveillance techniques have been adopted. According to a one time-trend study, the relative risk of CRC decreased from 1.34 in 1979–1988 to 0.57 in 1999–2008 [6]. In this sense, proper cancer surveillance with conventional and novel endoscopic techniques has major clinical implications for patients with IBD [7].
Generally, the standard recommendations for random biopsy in surveillance colonoscopy for IBD patients include four quadrant biopsies taken every 10 cm. These biopsies generally begin 8 to 10 years after diagnosis. Extra biopsies can be obtained from strictured, raised, or color changed areas in the colorectum [8-13]. However, these biopsies can be time consuming and laborious. Recent endoscopic techniques are evolving with the aim of visualizing detailed surface architecture of the mucosa, vascular patterns, and even the cellular and subcellular structures in real time. Precise observation and targeted biopsy are possible with the progress of technologies such as high definition endoscopy, narrow band imaging, chromoendoscopy, confocal endomicroscopy, etc. The present review focuses on novel endoscopic technologies and diagnostic strategies for inflammation and dysplasia in IBD patients.
Endoscopic techniques have led to improved observation of mucosal details, which may lead to reduced random biopsies since biopsies will be able to be targeted for histological evaluation. These techniques include image enhancement with modifying conventional endoscopy and improvement in mucosal imaging with magnification or several optical techniques (Table 1).
Each of these techniques is at a different stage of development and use in clinical medicine. Some of the equipment, such as probe-based or scope-based confocal laser techniques or endocytoscopy, are available only in specialized academic centers, whereas high definition endoscopy has become the standard and is widely used in clinical practice. In addition, specialized training and adequate clinical experience are necessary to adequately perform these novel endoscopies. In the case of image-enhanced endoscopy, it is important to prepare the patient with bowel cleansing in order to ensure the efficacy and safety of the procedure prior to use. This technique should be used to visualize a specific area in detail rather than for observation of the entire colon. Each of the advanced endoscopies has their own advantages and limitations. These techniques are far from being used as the gold standard in IBD, and some studies have been controversial. Thus, it requires more experience before implementing them in clinical practice and cautious use for patients on clinical use.
High definition or high resolution endoscopy presents signal images with 850,000 to 1 million pixels, while standard definition signals offer 100,000 to 400,000 pixels on an SD format [14,15]. High resolution endoscopy results in visualization of subtle mucosal details and improves the sensitivity and specificity of dysplastic lesion detection. Furthermore, it facilitates endoscopic resection by delineating borders of neoplastic lesions in IBD patients.
The majority of published data comes from non-IBD patients and found high definition endoscopy to be superior compared with conventional endoscopy. A retrospective study with 160 colonoscopies including long-standing (>7 years) colonic IBD patients demonstrated 2.21 greater likelihood (95% confidence interval [CI], 1.09 to 4.45) adjusted prevalence ratio of detecting any dysplastic lesions and 2.99 (95% CI, 1.16 to 7.79) of detecting dysplastic lesions on targeted biopsy with high definition colonoscopy compared to conventional endoscopy [16]. There was also a 3-fold higher neoplasia detection rate with high definition endoscopy when compared with standard definition endoscopy in IBD patients.
Chromoendoscopy is considered a cost-effective technique intended to enhance visualization of mucosal detail, submucosal vascular patterns, and lesion characterization. In particular, chromoendoscopy can facilitate the identification of flat lesions harboring intraepithelial neoplasia. With this, it can guide biopsies and reduces the number of biopsies. It is divided into dye-based and dye-less imaging techniques.
Dye-based chromoendoscopy has been used for over a decade and increases the rate of dysplastic lesion detection, especially in patients with long-standing IBD (Fig. 1A). In addition, Dye-based chromoendoscopy allows for improved assessment of disease severity and extent. Absorptive agents (e.g., Lugol solution, methylene blue, toludine blue, and cresyl violet), contrast agents (e.g., indigo carmine and acetic acid), agents for tattooing (e.g., India ink, Indocyanine green, and methylene blue), and reactive staining agents (e.g., congo red and phenol red) can be used in dye-based chromoendoscopy [17,18]. Several studies have shown the superiority of chromoendoscopy compared to conventional white light endoscopy. Dye-based chromoendoscopy has a moderate to high sensitivity for diagnosis, improved dysplasia detection, and prediction of mucosal change using magnification techniques (Fig. 1B). Two meta-analyses also demonstrated the superiority of targeted biopsy with dye-based chromoendoscopy in diagnosing and assessing mucosal ulcerations and dysplasia [19,20] while reducing the number of biopsies. Most recently, Soetikno et al. [20] included 665 patients from 6 studies and confirmed that the rate of detection of any dysplasia was approximately 9 times higher with dye-based chromoendoscopy with targeted biopsy than using white light endoscopy, with an 8.9 pooled odds ratio (95% CI, 3.4 to 23.0). When comparing the difference in the mean procedure time, dye-based chromoendoscopy is 10.9 minutes shorter than white light endoscopy, including the time spent on random biopsies.
Dye-less chromoendoscopy is a novel imaging technology that allows for a detailed examination of both the mucosal surface and the mucosal vascular pattern by pushing a button on the handle of the endoscope, thereby enabling high-contrast imaging of the mucosal surface in real time without the use of special equipment. These dye-less chromoendoscopy techniques are divided into two types. One is an optical filter system including narrow band imaging (NBI) from Olympus, Tokyo, Japan and Compound Band Imaging from Aohua, Shanghai, China, and the other is digital chromoendoscopy with a post-processing system including i-Scan from Pentax, Tokyo, Japan and FICE (Fuji intelligent color enhancement from Fujinon, Tokyo, Japan) [21,22].
Optical chromoendoscopy techniques are based on optical lenses integrated within the light source of the endoscope, usually in front of the excitation white light source, to narrow the bandwidth in the blue and green regions of the spectrum [23,24]. In contrast, digital chromoendoscopy uses digital postprocessing of endoscopic images made in real-time by the video processor [25]. Recent studies indicate that dye-less chromoendoscopy, including optical and digital ones, are useful and practical for the differentiation of adenoma versus hyperplastic colon polyps and have good histological correlations [26-28].
NBI is the most recognized among the virtual chromoendoscopy. This in vivo method uses optical filters in front of the light source to narrow the wavelength of the projected light to a 30 nm wide blue (415 nm) and green (540 nm) spectra, which enables visualization of micro-vessel morphological changes in superficial neoplastic lesions. NBI enhances the visibility of the small irregularities that accompany non-neoplastic inflammatory changes using the same logic as dye-based chromoendoscopy (Fig. 2).
However, the role of NBI in detecting dysplasia in IBD remains somewhat uncertain due to conflicting results in the literature. A paper by East et al. [29] was the first to describe the use of NBI to distinguish dysplastic from nondysplastic mucosa in patients with longstanding. Subsequent to this case report, several randomized controlled studies have been published. Dekker et al. [30] demonstrated that NBI does not improve the detection rate of neoplasia in UC compared with high-definition white light endoscopy with a randomized crossover study of 42 patients. Of 11 patients with neoplastic lesions, four were detected with both modalities, four with NBI alone, and three with standard white light colonoscopy alone.
Two additional randomized trials comparing NBI to white light endoscopy also found no significant difference in the detection of neoplastic lesions. Random background biopsies were also ineffective in detecting dysplasia. According to Ignjatovic et al. [31], dysplasia detection was 9% in each arm and the yield of dysplasia detection from random nontargeted biopsies was 0.04%. Van den Broek et al. [32] found 13 of 16 neoplastic lesions (81%) using high definition-NBI compared with 11 of 16 neoplastic lesions (69%) using high definition -white light endoscopy. A study using a new-generation NBI system compared with dye based chromoendoscopy for the early detection of colitis-associated dysplasia and cancer in patients with longstanding colonic IBD demonstrated that NBI is less time-consuming (26.87±9.89 minutes vs 15.74±5.62 minutes, p<.01), but has no advantages over conventional endoscopy for the detection of intraepithelial neoplasia [33]. However, NBI has some advantages over dye-based chromoendoscopy, as it does not require additional dye agents and is easier to use in practice. These findings have led to controversy regarding the real role of NBI in dysplasia detection in IBD patients.
Currently, two virtual chromoendoscopy techniques are available, including FICE and i-Scan is a new endoscopic system using post processing light filter technology based on software algorithms with real time image mapping. It enhances different elements of the mucosa by three different image processes such as surface enhancement, tone enhancement, and contrast enhancement. Activation between different modes is done by pushing a button on the handle of the endoscope [34,35]. To date, most randomized trials have not shown that NBI or FICE can improve the detection of colorectal neoplasia when comparing colonoscopy with and without filter enhancement.
A randomized controlled study was conducted on 78 IBD patients in Germany to identify whether i-Scan has the potential to enhance assessment of disease severity and extent in mild or inactive IBD patients. The average duration of the examination for high definition—white light endoscopy and i-Scan groups was 18 and 20.5 minutes, respectively, but these differences were not statistically significant. When comparing the endoscopic prediction of inflammatory extent and activity with the histological results, there was overall agreement of 48.71% and 53.85% in the high definition—white light endoscopy group and 92.31% and 89.74% in the i-Scan group (p<.001 and p=.066) [36].
Patients with intestinal food allergy present with lymphoid hyperplasia, slight mucosal edema, and blurred mucosal vascular pattern in the colon. Based on this, an observational study reported on the potential of i-Scan for prediction of mucosal changes with suspected food allergy. Positive and negative predictive values for i-Scan to predict food allergy were 92% and 80%, respectively. Moreover, i-Scan predicted food allergy with a sensitivity, specificity, and accuracy of 85%, 89%, and 86%, respectively [37].
Observation and characterization of the colonic mucosal surface and abnormalities of blood vessel architecture are crucial in predicting histology, and this can be performed more efficiently with chromoendoscopy. However, histologic confirmation is needed to determine whether the presence of mucosal abnormalities is a result of IBD or not. This can be accomplished by confocal endomicroscopy in vivo, which may provide images similar to histologic findings in real time (Fig. 3). Endomicroscopy is regarded as optical biopsy that can achieve an image of the cellular structure of the mucosa with 1,500 fold magnification [38]. Currently, two endomicroscopy systems are available including an integrated endoscopy system (iCLE, Pentax) and a probe-based system (pCLE, Cellvizio, Mauna Kea Technologies, Paris, France). In vivo CLE uses an excitation wavelength of 488 nm with a single line laser; the laser power output is up to 1 mW at the tissue surface. Images are collected at a scan rate of 0.8 frames per second at a resolution of 1,024×1,024 pixels or 1.6 frames per second with 1,024×512 pixels [39]. It can capture the z axis which enables interrogation of the epithelium and lamina propria 0–250 mm below the surface layer [40]. The pCLEsystem uses a fixed laser power and a fixed image plane depth. The purpose of the system is to observe mucosal microarchitecture with an increased field of view (4×2 mm) through postpocessing with Cellvio Viewer (Fig. 4A). It enables virtual staining of mucosal structures to further enhance tissue contrast. The probe requires an accessory channel of 2.8 mm and has a resolution of 1 μm with a field of view of 240 μm and a fixed image plane depth varying between 55–65 μm (Fig. 4B).
Crypt architecture, microvascular alterations, fluorescein leakage, and cellular infiltrates within the lamina propria are important observational markers in CLE evaluation [12,41-44]. CLE can aid in demonstrating mucosal healing in terms of deep remission beyond the absence of mucosal ulceration.
Watanabe et al. [45] investigated the features of CLE in the inflamed and noninflamed rectal mucosa of 17 UC patients and compared these results to standard histology. In this study, the crypts of colonic mucosa in active UC were large, variously shaped and irregular in arrangement. Numerous inflammatory cells and capillaries were visible in the lamina propria with CLE. Li et al. [42] also assessed crypt architecture, fluorescein leakage, and microvascular alterations in 73 consecutive UC patients and showed a correlation with histological results (p<.001). On post-CLE objective assessment, subjective architectural classifications were supported by the number of crypts per image (p<.001), but not fluorescein leakage results by gray scale (p=.194). Most recently, CLE also proved a sensitive tool in predicting UC relapse. In this study, 17 of 20 patients (85%) with histologically confirmed normal or chronic inflammation were diagnosed as having nonactive inflammation by real-time CLE. Twenty two of 23 patients (96%) with histologically confirmed acute inflammation were diagnosed as having active inflammation by CLE. The results of CLE were highly consistent with those of conventional histology (kappa value=0.812). Eleven percent of patients in the nonactive inflammation group relapsed, while 64% of patients in the active inflammation group relapsed. The relapse rate of patients with active inflammation was significantly higher than of those with nonactive inflammation (p<.001).
Neumann et al. [46] proposed the Crohn’s Disease Endomicroscopic Activity Score for assessing CD activity in vivo from comparison data between CD patients and a normal control group with standard white-light endoscopy followed by CLE. Active CD patients showed a higher proportion of increased colonic crypt tortuosity, enlarged crypt lumens, microerosions, augmented vascularization, and increased cellular infiltrates within the lamina propria. In the case of quiescent CD patients, there was a significant increase in crypt and goblet cell number compared with controls.
Autofluorescence imaging (AFI) is a technique using the natural principle that cells contain molecules that become fluorescent when excited by UV/Vis radiation of a certain wavelength. Among the endogenous fluorophores, collagen and elastin have a relatively high quantum yield, so the extracellular matrix usually contributes to the autofluorescence emission more than cellular components. Autofluorescence imaging videoendoscopy produces real-time pseudo-color images based on tissue autofluorescence emitted by excitation of endogenous tissue fluorophores.
It is well known that cell and tissue state change resulting from modifications of the amount and distribution of endogenous fluorophores and the chemical-physical properties of their microenvironment during physiological and/or pathological processes. Therefore, AFI can be utilized in order to obtain information about the morphological and physiological state of cells and tissues (Fig. 2).
AFI has been used to highlight various lesions, such as neoplastic tissue, minimal changes in reflux esophagitis, the extent of chronic atrophic fundal gastritis, and Barrett’s esophagus [47-50]. AFI improves detection rates of neoplasia in patients with IBD and decreases the number of random biopsies needed. In a randomized, comparative study with 50 UC patients, neoplasia miss-rates for AFI and white light endoscopy were 0% and 50%, respectively (p=.036) [51]. AFI had 100% of sensitivity since all neoplasia was colored purple on AFI, while NBI had a 75% of sensitivity according to the Kudo classification.
AFI also has the ability to detect inflammatory lesions, including microscopic activity, in the colonic mucosa. Osada et al. [52] evaluated 572 images from 42 UC patients including white light endoscopy and AFI to validate the clinical relevance of AFI endoscopy for the assessment of the severity of inflammation. The green color component of AFI corresponded more closely with mucosal inflammation sites (r=–0.62, p<.01) than the red (r=0.52, p<.01) or blue (r=0.56, p<.01) color components. There were significant differences in green color components between limited (0.399±0.042) and extensive (0.375±0.044) (p=.014) polymorphonuclear cell infiltration within MES-0. It was observed that the green color component of AFI decreased as the severity of the mucosal inflammation increased.
Endocytoscopy (Olympus, Tokyo, Japan) is a new technique, enabling observation of the gastrointestinal mucosa at the cellular level. Microscopic imaging for the gut mucosal layer can be observed at a magnification up to 1,400-fold with a contact light microscope [53]. It requires preparation of the mucosal layer with absorptive contrast agents like methylene blue or toluidine blue. Thus, endoscopists can distinguish architectural details such as epithelial structure, cellular features, and vascular patterns in terms of size, leakage, and tortuosity [54-56].
Some studies suggest that endocytoscopy has a potential role in in vivo evaluation. A study in patients who have colorectal aberrant crypt foci demonstrated that endocystoscopy was able to detect tissue abnormalities in the normal mucosa surrounding CRC and to identify neoplasia in aberrant crypt foci with 91.4% sensitivity [56]. A pilot study with IBD patients showed that endocytoscopy could reliably distinguish single inflammatory cells with high sensitivities and specificities (neutrophilic [60% and 95%], basophilic [74.43% and 94.44%], eosinophilic granulocytes [75% and 90.48%], and lymphocytes [88.89% and 93.33%]). It also showed that the concordance between endocytoscopy and histopathology for grading intestinal disease activity in IBD was 100% [54]. This new imaging technique introduces possibilities for the development of in vivo research while allowing surface magnification at cellular and subcellular resolution, but little data is currently available on endocytoscopy.
Diagnostic techniques in the field of IBD including endoscopy, molecular pathology, genetics, epigenetics, metabolomics, and proteomics have emerged over the past few decades. An improvement in endoscopic techniques has enabled precise diagnosis and identification of dysplasia with advanced image processing software and optical filter technology. The two major advances provide better recognition of abnormalities enabling a refined classification and characterize the extent and depth of the inflammation or mucosal healing, facilitating targeted biopsy. Real-time microscopy during the ongoing endoscopy at a subcellular resolution is noninvasive and timesaving. These features provide high diagnostic accuracy for the detection of disease activity, location, severity, and complications and can provide valuable guidance for choosing medical and surgical treatments (Table 2).
Despite the promising data, the generalizability of the procedure should be confirmed with more well designed clinical investigations. Moreover, the utility of these techniques are dependent on the skill of the observers, so it is practically impossible to avoid “intra-observer variation” and “inter-observer variation.”
The new endoscopic imaging modalities used in clinical practice still warrant further investigation. In addition, even if endoscopy in IBD patients is clear, final diagnosis of intraepithelial neoplasia and disease activity still remains on histopathology. It will be important to identify the challenges associated with implementing these advanced endoscopy techniques in clinical practice.
REFERENCES
1. Hommes DW, van Deventer SJ. Endoscopy in inflammatory bowel diseases. Gastroenterology. 2004; 126:1561–73.


2. Stange EF, Travis SP, Vermeire S, et al. European evidence based consensus on the diagnosis and management of Crohn’s disease: definitions and diagnosis. Gut. 2006; 55 Suppl 1:i1–15.


3. Rubin DC, Shaker A, Levin MS. Chronic intestinal inflammation: inflammatory bowel disease and colitis-associated colon cancer. Front Immunol. 2012; 3:107.


4. Eaden JA, Abrams KR, Mayberry JF. The risk of colorectal cancer in ulcerative colitis: a meta-analysis. Gut. 2001; 48:526–35.


5. Laukoetter MG, Mennigen R, Hannig CM, et al. Intestinal cancer risk in Crohn’s disease: a meta-analysis. J Gastrointest Surg. 2011; 15:576–83.


6. Jess T, Simonsen J, Jørgensen KT, Pedersen BV, Nielsen NM, Frisch M, et al. Decreasing risk of colorectal cancer in patients with inflammatory bowel disease over 30 years. Gastroenterology. 2012; 143:375–81. e1.


7. Cheon JH, Kim WH. Recent advances of endoscopy in inflammatory bowel diseases. Gut Liver. 2007; 1:118–25.


8. Rutter MD, Saunders BP, Wilkinson KH, et al. Thirty-year analysis of a colonoscopic surveillance program for neoplasia in ulcerative colitis. Gastroenterology. 2006; 130:1030–8.


9. Panaccione R. The approach to dysplasia surveillance in inflammatory bowel disease. Can J Gastroenterol. 2006; 20:251–3.


10. Biancone L, Michetti P, Travis S, et al. European evidence-based consensus on the management of ulcerative colitis: special situations. J Crohns Colitis. 2008; 2:63–92.


11. Eaden JA, Mayberry JF; British Society for Gastroenterology; Association of Coloproctology for Great Britain and Ireland. Guidelines for screening and surveillance of asymptomatic colorectal cancer in patients with inflammatory bowel disease. Gut. 2002; 51 Suppl 5:V10–2.


12. Kiesslich R, Goetz M, Lammersdorf K, et al. Chromoscopy-guided endomicroscopy increases the diagnostic yield of intraepithelial neoplasia in ulcerative colitis. Gastroenterology. 2007; 132:874–82.


13. Hlavaty T, Huorka M, Koller T, et al. Colorectal cancer screening in patients with ulcerative and Crohn’s colitis with use of colonoscopy, chromoendoscopy and confocal endomicroscopy. Eur J Gastroenterol Hepatol. 2011; 23:680–9.


14. ASGE Technology Committee. High-definition and high-magnification endoscopes. Gastrointest Endosc. 2014; 80:919–27.
15. Udagawa T, Amano M, Okada F. Development of magnifying video endoscopes with high resolution. Dig Endosc. 2001; 13:163–9.


16. Subramanian V, Ramappa V, Telakis E, et al. Comparison of high definition with standard white light endoscopy for detection of dysplastic lesions during surveillance colonoscopy in patients with colonic inflammatory bowel disease. Inflamm Bowel Dis. 2013; 19:350–5.


17. Kiesslich R, Neurath MF. Chromoendoscopy in inflammatory bowel disease. Gastroenterol Clin North Am. 2012; 41:291–302.


19. Subramanian V, Mannath J, Ragunath K, Hawkey CJ. Meta-analysis: the diagnostic yield of chromoendoscopy for detecting dysplasia in patients with colonic inflammatory bowel disease. Aliment Pharmacol Ther. 2011; 33:304–12.


20. Soetikno R, Subramanian V, Kaltenbach T, et al. The detection of nonpolypoid (flat and depressed) colorectal neoplasms in patients with inflammatory bowel disease. Gastroenterology. 2013; 144:1349–52. e1-6.


21. Goetz M, Kiesslich R. Advanced imaging of the gastrointestinal tract: research vs. clinical tools? Curr Opin Gastroenterol. 2009; 25:412–21.


23. Kuznetsov K, Lambert R, Rey JF. Narrow-band imaging: potential and limitations. Endoscopy. 2006; 38:76–81.


24. Subramanian V, Bisschops R. Image-enhanced endoscopy is critical in the surveillance of patients with colonic IBD. Gastrointest Endosc Clin N Am. 2014; 24:393–403.


25. Tontini GE, Vecchi M, Neurath MF, Neumann H. Review article: newer optical and digital chromoendoscopy techniques vs. dyebased chromoendoscopy for diagnosis and surveillance in inflammatory bowel disease. Aliment Pharmacol Ther. 2013; 38:1198–208.


26. van den Broek FJ, Reitsma JB, Curvers WL, Fockens P, Dekker E. Systematic review of narrow-band imaging for the detection and differentiation of neoplastic and nonneoplastic lesions in the colon (with videos). Gastrointest Endosc. 2009; 69:124–35.


27. Pohl J, Nguyen-Tat M, Pech O, May A, Rabenstein T, Ell C. Computed virtual chromoendoscopy for classification of small colorectal lesions: a prospective comparative study. Am J Gastroenterol. 2008; 103:562–9.


28. Teixeira CR, Torresini RS, Canali C, et al. Endoscopic classification of the capillary-vessel pattern of colorectal lesions by spectral estimation technology and magnifying zoom imaging. Gastrointest Endosc. 2009; 69(3 Pt 2):750–6.


29. East JE, Suzuki N, von Herbay A, Saunders BP. Narrow band imaging with magnification for dysplasia detection and pit pattern assessment in ulcerative colitis surveillance: a case with multiple dysplasia associated lesions or masses. Gut. 2006; 55:1432–5.


30. Dekker E, van den Broek FJ, Reitsma JB, et al. Narrow-band imaging compared with conventional colonoscopy for the detection of dysplasia in patients with longstanding ulcerative colitis. Endoscopy. 2007; 39:216–21.


31. Ignjatovic A, East JE, Subramanian V, et al. Narrow band imaging for detection of dysplasia in colitis: a randomized controlled trial. Am J Gastroenterol. 2012; 107:885–90.


32. van den Broek FJ, Fockens P, van Eeden S, et al. Narrow-band imaging versus high-definition endoscopy for the diagnosis of neoplasia in ulcerative colitis. Endoscopy. 2011; 43:108–15.


33. Pellisé M, López-Cerón M, Rodríguez de Miguel C, et al. Narrowband imaging as an alternative to chromoendoscopy for the detection of dysplasia in long-standing inflammatory bowel disease: a prospective, randomized, crossover study. Gastrointest Endosc. 2011; 74:840–8.


34. Hoffman A, Sar F, Goetz M, et al. High definition colonoscopy combined with i-Scan is superior in the detection of colorectal neoplasias compared with standard video colonoscopy: a prospective randomized controlled trial. Endoscopy. 2010; 42:827–33.


35. Kodashima S, Fujishiro M. Novel image-enhanced endoscopy with i-scan technology. World J Gastroenterol. 2010; 16:1043–9.


36. Neumann H, Vieth M, Günther C, et al. Virtual chromoendoscopy for prediction of severity and disease extent in patients with inflammatory bowel disease: a randomized controlled study. Inflamm Bowel Dis. 2013; 19:1935–42.
37. Neumann H, Vieth M, Tontini GE, et al. 33 High-definition endoscopy with virtual chromoendoscopy for prediction of food allergy in real-time: a prospective, randomized study with cross-over design. Gastroenterology. 2014; 146(5 Suppl 1):S-9–S-10.


38. Liu JT, Loewke NO, Mandella MJ, Levenson RM, Crawford JM, Contag CH. Point-of-care pathology with miniature microscopes. Anal Cell Pathol (Amst). 2011; 34:81–98.


40. Iacucci M, Panaccione R, Ghosh S. Advances in novel diagnostic endoscopic imaging techniques in inflammatory bowel disease. Inflamm Bowel Dis. 2013; 19:873–80.


41. Watanabe O, Ando T, Maeda O, et al. Confocal endomicroscopy in patients with ulcerative colitis. J Gastroenterol Hepatol. 2008; 23 Suppl 2:S286–90.


42. Li CQ, Xie XJ, Yu T, et al. Classification of inflammation activity in ulcerative colitis by confocal laser endomicroscopy. Am J Gastroenterol. 2010; 105:1391–6.


43. Kiesslich R, Burg J, Vieth M, et al. Confocal laser endoscopy for diagnosing intraepithelial neoplasias and colorectal cancer in vivo. Gastroenterology. 2004; 127:706–13.
44. Li CQ, Liu J, Ji R, Li Z, Xie XJ, Li YQ. Use of confocal laser endomicroscopy to predict relapse of ulcerative colitis. BMC Gastroenterol. 2014; 14:45.


45. Watanabe C, Sumioka M, Hiramoto T, et al. Magnifying colonoscopy used to predict disease relapse in patients with quiescent ulcerative colitis. Inflamm Bowel Dis. 2009; 15:1663–9.


46. Neumann H, Vieth M, Atreya R, et al. Assessment of Crohn’s disease activity by confocal laser endomicroscopy. Inflamm Bowel Dis. 2012; 18:2261–9.


47. Asaoka D, Nagahara A, Kurosawa A, et al. Utility of autofluorescence imaging videoendoscopy system for the detection of minimal changes associated with reflux esophagitis. Endoscopy. 2008; 40 Suppl 2:E172–3.


48. Inoue T, Uedo N, Ishihara R, et al. Autofluorescence imaging videoendoscopy in the diagnosis of chronic atrophic fundal gastritis. J Gastroenterol. 2010; 45:45–51.


49. Asaoka D, Nagahara A, Oguro M, et al. Utility of autofluorescence imaging videoendoscopy in screening for Barrett’s esophagus. Endoscopy. 2009; 41 Suppl 2:E113.


50. Nakaniwa N, Namihisa A, Ogihara T, et al. Newly developed autofluorescence imaging videoscope system for the detection of colonic neoplasms. Dig Endosc. 2005; 17:235–40.


51. van den Broek FJ, Fockens P, van Eeden S, et al. Endoscopic trimodal imaging for surveillance in ulcerative colitis: randomised comparison of high-resolution endoscopy and autofluorescence imaging for neoplasia detection; and evaluation of narrow-band imaging for classification of lesions. Gut. 2008; 57:1083–9.


52. Osada T, Arakawa A, Sakamoto N, et al. Autofluorescence imaging endoscopy for identification and assessment of inflammatory ulcerative colitis. World J Gastroenterol. 2011; 17:5110–6.


53. Neumann H, Vieth M, Neurath MF. Image of the month. Endocytoscopy-based detection of focal high-grade intraepithelial neoplasia in colonic polyps. Clin Gastroenterol Hepatol. 2011; 9:e13.
54. Neumann H, Vieth M, Neurath MF, Atreya R. Endocytoscopy allows accurate in vivo differentiation of mucosal inflammatory cells in IBD: a pilot study. Inflamm Bowel Dis. 2013; 19:356–62.
55. Pohl H, Rosch T, Tanczos BT, Rudolph B, Schlüns K, Baumgart DC. Endocytoscopy for the detection of microstructural features in adult patients with celiac sprue: a prospective, blinded endocytoscopyconventional histology correlation study. Gastrointest Endosc. 2009; 70:933–41.


56. Cipolletta L, Bianco MA, Rotondano G, et al. Endocytoscopy can identify dysplasia in aberrant crypt foci of the colorectum: a prospective in vivo study. Endoscopy. 2009; 41:129–32.
Fig. 1.
Chromoendoscopy using indigocarmine (A) and combined with magnification technique (B) for colonic dysplasia in ulcerative colitis (Courtesy of Dr. Jeong-Sik Byeon at Asan Medical Center).
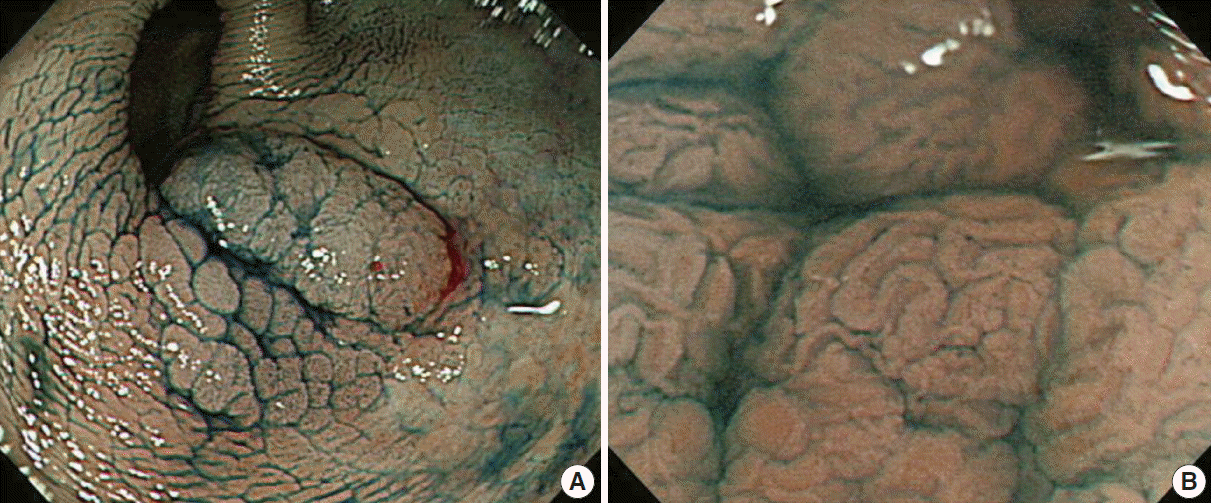
Fig. 2.
Observation findings of colonic dysplasia using white light endoscopy (A), narrow band imaging technique (B), and autofluorescence imaging technique (C) in ulcerative colitis (Courtesy of Dr. Jeong-Sik Byeon at Asan Medical Center).
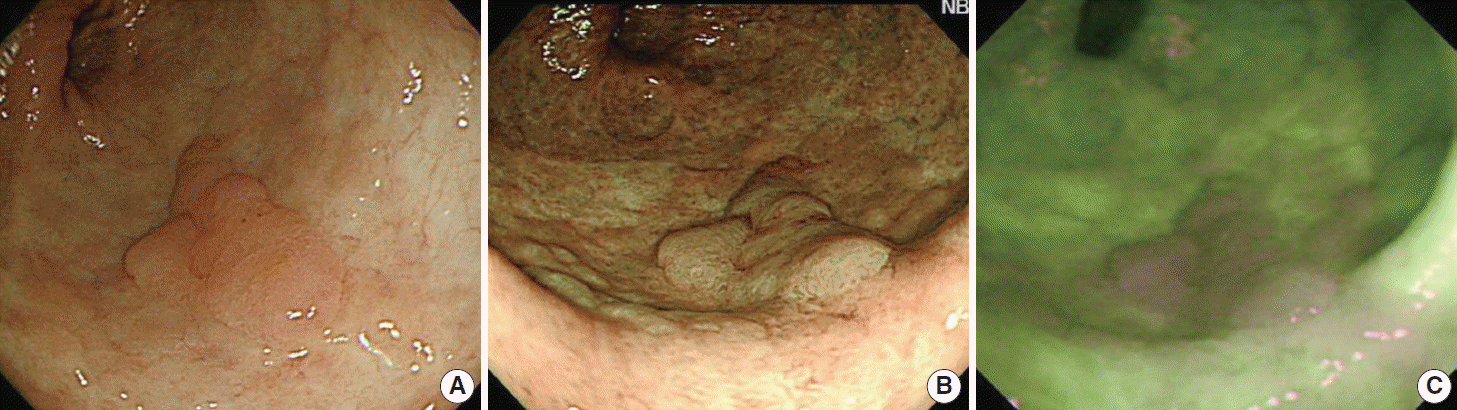
Fig. 3.
Confocal laser endomicroscopic findings for normal mucosa (A) and mucosa in active ulcerative colitis (B). In ulcerative colitis, lamina propria widening, inflammatory infiltrates, goblet cell depletion, and crypt distortion are observed.
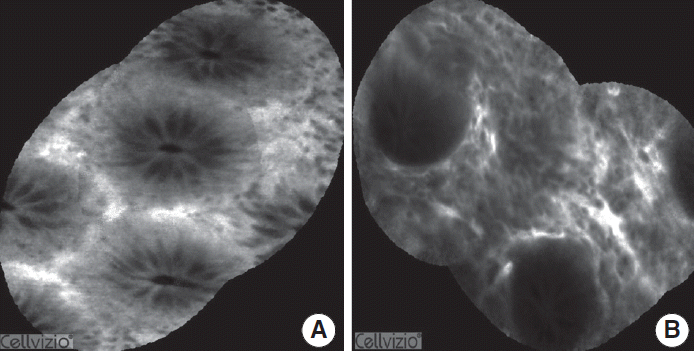
Table 1.
Categories of endoscopic techniques used in inflammatory bowel disease
Table 2.
Potential clinical use of image-enhanced endoscopy in inflammatory bowel disease