Abstract
Background
Although the correlation between low claudin-1 expression and worse prognosis has been reported, details on the prognostic implications of claudin-1 expression in various malignant tumors remain unclear. The present study aimed to elucidate the prognostic roles of claudin- 1 immunohistochemistry (IHC) in various malignant tumors through a meta-analysis.
Methods
The study included 2,792 patients from 22 eligible studies for assessment of the correlation between claudin-1 expression and survival rate in various malignant tumors. A subgroup analysis based on the specific tumor and evaluation criteria of claudin-1 IHC was conducted.
Results
Low claudin-1 expression was significantly correlated with worse overall survival (OS) (hazard ratio [HR], 1.851; 95% confidence interval [CI], 1.506 to 2.274) and disease-free survival (DFS) (HR, 2.028; 95% CI, 1.313 to 3.134) compared to high claudin-1 expression. Breast, colorectal, esophageal, gallbladder, head and neck, and lung cancers, but not cervical, liver or stomach cancers, were significantly correlated with worse OS. Breast, colorectal, esophageal, and thyroid cancers with low claudin-1 expression were associated with poorer DFS. In the lower cut-off subgroup (< 25.0%) with respect to claudin-1 IHC, low claudin-1 expression was significantly correlated with worse OS and DFS.
Claudins comprise a large family of tetraspan trans-membrane proteins and are required for tight junction formation [1]. Claudin-1 expression is regulated by the β-catenin–T-cell factor/lymphoid enhancing factor signaling pathway [2,3]. Claudins have potentially different functions driven by the formation of homotypic or heterotypic interactions across the junction in various tissues [4-8]. The epithelial-to-mesenchymal transition, which is correlated with tumor invasiveness, is affected by the change of expression and the redistribution of tight junction proteins including claudin [9]. Expression patterns of claudins can differ by tumor or tissue type, which can affect tumor behavior and prognosis [10]. The prognostic roles of claudin-1 have been diverse in several studies involving various tumor types [9,11-31]. However, in stomach cancer, increased claudin-1 expression in tumor cells has been significantly correlated with worse prognosis in the intestinal type, but not in the diffuse type. In kidney and lung cancers, high claudin-1 expression has likewise been correlated with worse prognosis [12,14]. Although a correlation between low claudin-1 expression and worse prognosis has been reported, details on the prognostic implications of claudin-1 expression in various malignant tumors remain unclear.
To elucidate the prognostic role of claudin-1 immunohistochemistry (IHC), the correlation between claudin-1 IHC expression and survival rates was investigated in various malignant tumors. A subgroup analysis based on the evaluation criteria of claudin-1 IHC was conducted.
Relevant articles were obtained by searching the PubMed and MEDLINE databases through February 28, 2018. The search was performed using ‘claudin-1’ and ‘survival’ as search terms. The titles and abstracts of all returned articles were screened for exclusion. Review articles were also screened to find additional eligible studies. English language studies addressing claudin-1 IHC expression in human malignant tumors and the correlation between claudin-1 IHC expression and survival rate were included. Case reports were excluded. This meta-analysis did not require the approval of an institutional review board.
The following information was collected and verified from the full texts of eligible studies: [9,11-31] first author’s name, publication date, study location, number of patients analyzed, antibody manufacturer, dilution ratio, cut-offs for assessing high claudin-1 IHC expression, tumor type, and data allowing estimation of the impact of claudin-1 IHC expression on overall survival (OS) and disease-free survival (DFS). We did not define a minimum number of patients to be included in a study. Any disagreements were resolved by consensus.
To perform the meta-analysis, all data were analyzed using the Comprehensive Meta-Analysis software package (Biostat, Englewood, NJ, USA). Correlations between claudin-1 IHC expression and survival were measured by hazard ratios (HR) obtained from the eligible study data. We aggregated the estimated HR and its standard error using given parameters, which were the HR point estimate, log-rank statistic or its p-value, O–E statistic (difference between numbers of observed and expected events), or its variance [32]. If the extractable data only included the survival curve, two persons independently extracted survival rates to reduce reading variability, according to Parmar’s recommendation [32]. Meta-analysis was performed using fixed-effects and randomeffects models. The values pooled using the random effects model were utilized for interpretation. Subsequently, a study showing results of an estimated HR > 1, with a 95% confidence interval (CI) that does not include 1, implied poor survival with a low or a loss of claudin-1 expression. Because eligible studies used various antibodies and evaluation criteria, a random-effects model was more suitable than a fixed-effects model. Subgroup analyses based on specific organs and cut-off value for high expression of claudin-1 IHC were performed. In addition, heterogeneous and sensitivity analyses were conducted to assess the heterogeneity of eligible studies and the impact of each study on the combined effect, respectively. Heterogeneity between studies was checked by the Q and I2 statistics and demonstrated p-values. For assessment of publication bias, Begg’s funnel plot and Egger’s test were performed. The results were considered statistically significant when p < 0.05.
One hundred seventy reports were identified in the database search. Of these, 44 were excluded due to lack of sufficient information. Other studies were excluded because they reported the results of other diseases (n = 38), used animals or cell lines (n = 57), were not written in English (n = 5), or were non-original articles (n = 3) (Fig. 1). In addition, one article was excluded due to duplication. After applying the inclusion and exclusion criteria, 22 reports were finally included in the meta-analysis (Table 1). Eligible studies used various manufacturers’ antibodies and variable dilution ratios, as shown in Table 1. The cut-off values to distinguish between low or high claudin-1 IHC expressions varied between 1% and 50%.
The correlation between low expression of claudin-1 IHC and survival was investigated by subdividing data according to OS in 15 studies and according to DFS in 15 studies. Low expression of claudin-1 IHC was significantly correlated with worse OS (HR, 1.851; 95% CI, 1.506 to 2.274) (Fig. 2A) and DFS (HR, 2.028; 95% CI, 1.313 to 3.134) (Fig. 2B). Eligible studies showed significant heterogeneity in OS and DFS. Sensitivity analysis showed that eligible studies had no effect on the pooled HR. In sensitivity analysis, the ranges of HRs were 1.745–1.917 and 1.869–2.324 in OS and DFS, respectively. There was no evidence of publication bias in Egger’s test (p = .505 in OS and p = .956 in DFS) and no asymmetry in Begg’s funnel plot.
We conducted subgroup analysis based on specific tumors. In OS, low expression of claudin-1 IHC was significantly correlated with a lower survival rate in breast, colorectal, esophageal, gallbladder, head and neck, and lung cancers, but not in cervical, liver, or stomach cancers (Table 2). In addition, there was significant correlation between low expression of claudin-1 IHC and worse DFS in breast, colorectal, esophageal, and thyroid cancers, but not in liver, lung, kidney, or stomach cancers (Table 2). However, in DFS of head and neck cancer, low claudin-1 IHC expression showed a reverse correlation (HR, 0.396; 95% CI, 0.160 to 0.765). The cancer with the highest HR was breast cancer in both OS (HR, 3.364; 95% CI, 1.898 to 5.961) and DFS (HR, 5.182; 95% CI, 3.749 to 7.162).
Next, to evaluate the optimal criteria of low claudin-1 IHC expression, we created subgroups using the median value of cutoffs from eligible studies (25.0%). The results of subgroup analysis based on the evaluation criteria are shown in Fig. 3. In OS, HRs were 1.738 (95% CI, 1.251 to 2.415) and 1.805 (95% CI, 1.252 to 2.602) in the ≥ 25.0% and < 25.0% cut-off subgroups, respectively. In DFS, HRs were 1.492 (95% CI, 0.808 to 2.753) and 2.611 (95% CI, 1.218 to 5.597) in subgroups with the ≥ 25.0% and < 25.0% cut-offs, respectively.
While claudin-1 has been studied in various malignant tumors, the prognostic role of claudin-1 IHC has not been fully elucidated and may vary [9,11-31]. The current study is the first meta-analysis of published studies on the prognostic role of claudin-1 IHC in various malignant tumors.
Although 27 subtypes of claudin are currently known in human tissues [33], the expressions and functions of claudins in malignant tumors are not fully understood. In tumor cells, claudin-1 participates in the up-regulation of ZEB-1, which induces reduction of E-cadherin expression and leads to invasive activity [34]. Loss of claudin-1 expression might induce loosening of tight junctions, which would alter the cohesion and invasiveness of tumor cells [1,7,8,35]. Furthermore, by loosening tight junctions, diffusion of nutrients and other growth factors may be increased in tumor cells, which could induce proliferation of tumor cells. Claudin-1 overexpression inhibits the dissociation of cancer cells and suppresses migration, invasion, and metastasis [13,36]. Claudin-1 can also be expressed in normal cells, but the function and expression pattern of claudin-1 might vary in different organs. More studies are needed to clarify the potential gamut of functions.
In the present meta-analysis, low expression of claudin-1 IHC was significantly correlated with worse OS and DFS in human malignant tumors (HR, 1.851; 95% CI, 1.506 to 2.274 and HR, 2.028; 95% CI, 1.313 to 3.134, respectively). Unlike the results of most reports showing the correlation between low expression of claudin-1 IHC and a lower survival rate, inverse correlations or no correlations were found in stomach cancer, clear renal cell carcinoma, and lung adenocarcinoma. Among eligible studies with stomach cancers, Huang et al. reported that low claudin-1 expression was significantly correlated with better survival rate [17]. The prognostic role of claudin-1 was different between intestinal and diffuse types of gastric cancer [10]. In addition, in meta-analysis for clear cell renal cell carcinoma, the estimated HR was 0.873 (95% CI, 0.385 to 1.981; p = .746) [14,27]. However, the estimated HR of papillary renal cell carcinoma was 1.972 (95% CI, 0.671 to 5.795), but there was no statistical significance (p = .217). Although low claudin-1 IHC expression was significantly correlated with poor prognosis in lung cancer, significant correlation was found in adenocarcinoma, but not squamous cell carcinoma [12,13,21,31]. Cancers with an HR exceeding 1 in both OS and DFS were breast, colorectal, and esophageal cancers.
This meta-analysis included studies using IHC for the evaluation of claudin-1 expression. The rate of low claudin-1 IHC expression ranged from 15.8% to 84.9%, which varied according to specific tumor type. Various cut-off values (1%–50%) were used in eligible studies; therefore, the rates of low or high claudin-1 IHC expression could be largely affected by the cut-off value. Consequently, this discrepancy might affect the correlation between claudin-1 IHC expression and survival rate. In addition, discrepancies between investigators were possible concerning the rates of low claudin-1 IHC expression. Detailed evaluations, such as subgroup analysis based on median cut-off values, were required to elucidate the cause of the large difference between eligible studies. In OS, the HR of cases with low claudin-1 IHC expression was significantly higher in both cut-off subgroups (Fig. 3). However, in the higher cut-off value subgroup, low claudin-1 IHC expression was not significantly correlated with worse DFS (HR, 1.492; 95% CI, 0.808 to 2.753). Our results suggest that a lower cut-off value might be more suitable for evaluation of low claudin-1 expression rather than a higher cut-off value. However, further studies based on specific tumor types will be needed to elucidate the effect of cut-off value on the correlation with survival.
There are a number of limitations in the current study. First, the study investigated the correlation between claudin-1 expression and prognosis in various malignant tumors. Although several studies have reported the prognostic roles of claudin-1, they were limited in their interpretive value because of the small number of studies for specific tumor types. Interestingly, in head and neck cancer, the prognostic roles of low claudin-1 IHC expression were different between OS and DFS [18,25]. The discrepancy between included studies could not be found due to the small number of studies. Second, the adequate antigenicity of included tissues in eligible studies could not be guaranteed due to the use of old specimens in some studies. Whether the low expression of claudin-1 reflected this problem could not be determined in the present study. Third, to avoid bias from follow-up periods, survival data were extracted at a 60-month follow-up. This follow-up period had no effect on the correlation between claudin-1 expression and survival in the present study, and the correlation between claudin-1 expression and survival could differ from those in previous reports.
In conclusion, low claudin-1 expression was significantly correlated with lower survival rates in various malignant tumors. Lower criteria for claudin-1 IHC expression could be suitable for prediction of a patient’s prognosis. More detailed criteria for claudin-1 IHC expression in various malignant tumors is needed for application of claudin-1 IHC expression in daily practice.
Notes
REFERENCES
1. Colegio OR, Van Itallie C, Rahner C, Anderson JM. Claudin extracellular domains determine paracellular charge selectivity and resistance but not tight junction fibril architecture. Am J Physiol Cell Physiol. 2003; 284:C1346–54.


2. Miwa N, Furuse M, Tsukita S, Niikawa N, Nakamura Y, Furukawa Y. Involvement of claudin-1 in the beta-catenin/Tcf signaling pathway and its frequent upregulation in human colorectal cancers. Oncol Res. 2001; 12:469–76.
3. Bhat AA, Sharma A, Pope J, et al. Caudal homeobox protein Cdx-2 cooperates with Wnt pathway to regulate claudin-1 expression in colon cancer cells. PLoS One. 2012; 7:e37174.


4. Tsukita S, Furuse M. The structure and function of claudins, cell adhesion molecules at tight junctions. Ann N Y Acad Sci. 2000; 915:129–35.


6. Krug SM, Schulzke JD, Fromm M. Tight junction, selective permeability, and related diseases. Semin Cell Dev Biol. 2014; 36:166–76.


7. Koval M. Claudin heterogeneity and control of lung tight junctions. Annu Rev Physiol. 2013; 75:551–67.


8. Capaldo CT, Nusrat A. Claudin switching: physiological plasticity of the tight Junction. Semin Cell Dev Biol. 2015; 42:22–9.


9. Huang J, Li J, Qu Y, et al. The expression of claudin 1 correlates with beta-catenin and is a prognostic factor of poor outcome in gastric cancer. Int J Oncol. 2014; 44:1293–301.
10. Suh Y, Yoon CH, Kim RK, et al. Claudin-1 induces epithelial-mesenchymal transition through activation of the c-Abl-ERK signaling pathway in human liver cells. Oncogene. 2013; 32:4873–82.


11. Bouchagier KA, Assimakopoulos SF, Karavias DD, et al. Expression of claudins-1, -4, -5, -7 and occludin in hepatocellular carcinoma and their relation with classic clinicopathological features and patients’ survival. In Vivo. 2014; 28:315–26.
12. Chae MC, Park CK, Keum DY, Hwang I, Kwon KY, Jang BC. Prognostic significance of claudin 4 in completely resected adenocarcinoma of the lung. Korean J Thorac Cardiovasc Surg. 2014; 47:262–8.


13. Chao YC, Pan SH, Yang SC, et al. Claudin-1 is a metastasis suppressor and correlates with clinical outcome in lung adenocarcinoma. Am J Respir Crit Care Med. 2009; 179:123–33.


14. Fritzsche FR, Oelrich B, Johannsen M, et al. Claudin-1 protein expression is a prognostic marker of patient survival in renal cell carcinomas. Clin Cancer Res. 2008; 14:7035–42.


15. Higashi Y, Suzuki S, Sakaguchi T, et al. Loss of claudin-1 expression correlates with malignancy of hepatocellular carcinoma. J Surg Res. 2007; 139:68–76.


16. Hoellen F, Waldmann A, Banz-Jansen C, et al. Claudin-1 expression in cervical cancer. Mol Clin Oncol. 2017; 7:880–4.


17. Jung H, Jun KH, Jung JH, Chin HM, Park WB. The expression of claudin-1, claudin-2, claudin-3, and claudin-4 in gastric cancer tissue. J Surg Res. 2011; 167:e185–91.


18. Li WJ, Zhang ZL, Yu XM, Cai XL, Pan XL, Yang XY. Expression of claudin-1 and its relationship with lymphatic microvessel generation in hypopharyngeal squamous cell carcinoma. Genet Mol Res. 2015; 14:11814–26.


19. Ma F, Ding X, Fan Y, et al. A CLDN1-negative phenotype predicts poor prognosis in triple-negative breast cancer. PLoS One. 2014; 9:e112765.


20. Matsuoka T, Mitomi H, Fukui N, et al. Cluster analysis of claudin-1 and -4, E-cadherin, and beta-catenin expression in colorectal cancers. J Surg Oncol. 2011; 103:674–86.
21. Merikallio H, Kaarteenaho R, Pääkkö P, et al. Impact of smoking on the expression of claudins in lung carcinoma. Eur J Cancer. 2011; 47:620–30.


22. Miyamoto K, Kusumi T, Sato F, et al. Decreased expression of claudin-1 is correlated with recurrence status in esophageal squamous cell carcinoma. Biomed Res. 2008; 29:71–6.


23. Morohashi S, Kusumi T, Sato F, et al. Decreased expression of claudin-1 correlates with recurrence status in breast cancer. Int J Mol Med. 2007; 20:139–43.


24. Resnick MB, Konkin T, Routhier J, Sabo E, Pricolo VE. Claudin-1 is a strong prognostic indicator in stage II colonic cancer: a tissue microarray study. Mod Pathol. 2005; 18:511–8.


25. Sappayatosok K, Phattarataratip E. Overexpression of claudin-1 is associated with advanced clinical stage and invasive pathologic characteristics of oral squamous cell carcinoma. Head Neck Pathol. 2015; 9:173–80.


26. Shibutani M, Noda E, Maeda K, Nagahara H, Ohtani H, Hirakawa K. Low expression of claudin-1 and presence of poorly-differentiated tumor clusters correlate with poor prognosis in colorectal cancer. Anticancer Res. 2013; 33:3301–6.
27. Shin HI, Kim BH, Chang HS, Kim CI, Jung HR, Park CH. Expression of claudin-1 and -7 in clear cell renal cell carcinoma and its clinical significance. Korean J Urol. 2011; 52:317–22.


28. Tzelepi VN, Tsamandas AC, Vlotinou HD, Vagianos CE, Scopa CD. Tight junctions in thyroid carcinogenesis: diverse expression of claudin-1, claudin-4, claudin-7 and occludin in thyroid neoplasms. Mod Pathol. 2008; 21:22–30.


29. Xiong L, Wen Y, Miao X, Yang Z. Expressions of cell junction regulatory proteins and their association with clinicopathologic parameters in benign and malignant gallbladder lesions. Am J Med Sci. 2011; 342:388–94.


30. Yoshida T, Kinugasa T, Akagi Y, et al. Decreased expression of claudin-1 in rectal cancer: a factor for recurrence and poor prognosis. Anticancer Res. 2011; 31:2517–25.
31. Zhang Z, Wang A, Sun B, Zhan Z, Chen K, Wang C. Expression of CLDN1 and CLDN10 in lung adenocarcinoma in situ and invasive lepidic predominant adenocarcinoma. J Cardiothorac Surg. 2013; 8:95.


32. Parmar MK, Torri V, Stewart L. Extracting summary statistics to perform meta-analyses of the published literature for survival endpoints. Stat Med. 1998; 17:2815–34.


33. Mineta K, Yamamoto Y, Yamazaki Y, et al. Predicted expansion of the claudin multigene family. FEBS Lett. 2011; 585:606–12.


34. Singh AB, Sharma A, Dhawan P. Claudin-1 expression confers resistance to anoikis in colon cancer cells in a Src-dependent manner. Carcinogenesis. 2012; 33:2538–47.


35. Kinugasa T, Akagi Y, Ochi T, et al. Increased claudin-1 protein expression in hepatic metastatic lesions of colorectal cancer. Anticancer Res. 2012; 32:2309–14.
Fig. 3.
Forest plot diagram for subgroup analysis based on cut-off value of low claudin-1 immunohistochemical expression. OS, overall survival; DFS, disease-free survival.
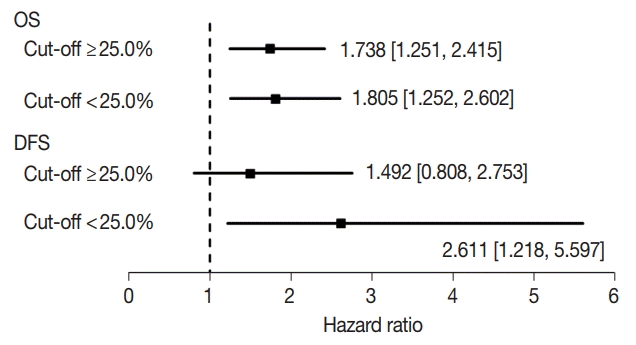
Table 1.
Main characteristics of eligible studies
Study | Location | Antibody company | Dilution | Cut-off value (%) | Organ | Tumor type |
Claudin-1 expression, n (%) |
|
---|---|---|---|---|---|---|---|---|
Low | High | |||||||
Ma et al. (2014) [19] | China | Zymed | 1:50 | 10 | Breast | - | 96 (55.5) | 77 (44.5) |
Morohashi et al. (2007) [23] | Japan | Zymed | 1:50 | 10 | Breast | - | 104 (52.0) | 96 (48.0) |
Matsuoka et al. (2011) [20] | Japan | Spring Bioscience | ND | 33.3 | Colorectum | - | 124 (79.5) | 32 (20.5) |
Resnick et al. (2005) [24] | USA | Zymed | 1:125 | NDa | Colorectum | - | 32 (25.0) | 96 (75.0) |
Shibutani et al. (2013) [26] | Japan | Zymed | 1:100 | 25 | Colorectum | - | 110 (32.0) | 234 (68.0) |
Yoshida et al. (2011) [30] | Japan | Zymed | 1:400 | 30 | Colorectum | - | 92 (49.7) | 93 (50.3) |
Miyamoto et al. (2008) [22] | Japan | Zymed | 1:100 | 10 | Esophagus | SCC | 11 (20.4) | 43 (79.6) |
Xiong et al. (2011) [29] | China | Fuzhou Maixin Biotech | ND | 25 | Gallbladder | - | 37 (55.2) | 30 (44.8) |
Li et al. (2015) [18] | China | Beijing ZSGB-BIO | ND | 40 | H&N | SCC | 26 (26.8) | 71 (73.2) |
Sappayatosok and Phattarataratip (2015) [25] | Thailand | Invitrogen | 1:200 | 50 | H&N | SCC | 33 (73.3) | 12 (26.7) |
Fritzsche et al. (2008) [14] | Switzerland | Zymed | 1:50 | 10 | Kidney | Clear cell RCC | 210 (75.5) | 68 (24.5) |
Papillary RCC | 6 (15.8) | 32 (84.2) | ||||||
Shin et al. (2011) [27] | Korea | Abcam | 1:200 | 5 | Kidney | Clear cell RCC | 101 (84.9) | 18 (15.1) |
Bouchagier et al. (2014) [11] | Greece | Zymed | 1:40 | 1a | Liver | HCC | 9 (13.4) | 58 (86.6) |
Higashi et al. (2007) [15] | USA | Zymed | 1:50 | ND | Liver | HCC | 28 (50.9) | 27 (49.1) |
Chae et al. (2014) [12] | Korea | Abcam | 1:500 | 50 | Lung | AdCa | ND | ND |
Chao et al. (2009) [13] | Taiwan | Zymed | ND | ND | Lung | AdCa | 34 (50.7) | 33 (49.3) |
Merikallio et al. (2011) [21] | Finland | Zymed | 1:50 | 25 | Lung | SCC | 15 (12.1) | 109 (87.9) |
AdCa | 2 (20.0) | 8 (80.0) | ||||||
Zhang et al. (2013) [31] | China | Zymed | ND | 10 | Lung | AdCa | 33 (40.7) | 48 (59.3) |
Huang et al. (2014) [9] | China | Zymed | 1:100 | 5 | Stomach | - | 63 (36.4) | 110 (63.6) |
Jung et al. (2011) [17] | Korea | Lab Vision | 1:200 | 25 | Stomach | - | 31 (43.1) | 41 (56.9) |
Tzelepi et al. (2008) [28] | Greece | Zymed | 1:100 | 5 | Thyroid | - | 24 (26.4) | 67 (73.6) |
Hoellen et al. (2017) [16] | Germany | Thermo Fisher Scientific Inc. | ND | > 0 | Uterine cervix | SCC | 14 (13.2) | 92 (86.8) |
Table 2.
Meta-analysis for the correlation between low claudin-1 expression and overall and disease-free survival
No. of subset | Fixed effect (95% CI) | Heterogeneity test p-value | Random effect (95% CI) | Egger’s test p-value | |
---|---|---|---|---|---|
Overall survival | |||||
Breast* | 1 | 3.364 (1.898–5.961) | > .99 | 3.364 (1.898–5.961) | - |
Cervix | 1 | 0.765 (0.321–1.823) | > .99 | 0.765 (0.321–1.823) | - |
Colorectum* | 3 | 2.354 (1.618–3.425) | .013 | 2.906 (1.244–6.786) | .286 |
Esophagus* | 1 | 1.988 (1.009–3.916) | > .99 | 1.988 (1.009–3.916) | - |
Gallbladder* | 1 | 1.779 (1.071–2.955) | > .99 | 1.779 (1.071–2.955) | - |
Head and neck* | 1 | 1.759 (1.020–3.034) | > .99 | 1.759 (1.020–3.034) | - |
Liver | 2 | 1.652 (0.995–2.742) | .508 | 1.652 (0.995–2.742) | - |
Lung* | 4 | 1.731 (1.275–2.350) | .391 | 1.731 (1.275–2.350) | .648 |
Stomach | 2 | 1.411 (0.985–2.022) | .431 | 1.411 (0.985–2.022) | - |
Disease-free survival | |||||
Breast* | 2 | 5.182 (3.749–7.162) | .353 | 5.182 (3.749–7.162) | - |
Colorectum* | 4 | 2.599 (1.963–3.441) | .025 | 3.012 (1.763–5.146) | .178 |
Esophagus* | 1 | 2.375 (1.177–4.791) | > .99 | 2.375 (1.177–4.791) | - |
Head and neck* | 1 | 0.396 (0.160–0.765) | > .99 | 0.396 (0.160–0.765) | - |
Liver | 1 | 1.394 (0.800–2.427) | > .99 | 1.394 (0.800–2.427) | - |
Lung | 1 | 1.014 (0.634–1.623) | > .99 | 1.014 (0.634–1.623) | - |
Kidney | 3 | 0.866 (0.589–1.274) | .056 | 1.072 (0.510–2.254) | .133 |
Stomach | 1 | 0.838 (0.395–1.778) | > .99 | 0.838 (0.395–1.778) | - |
Thyroid* | 1 | 8.697 (3.120–24.241) | > .99 | 8.697 (3.120–24.241) | - |